Introduction
Esterases (acyl hydrolases, EC. 3.1.1.X) and more specifically lipases (triacylglycerol acyl hydrolases, EC 3.1.1.3) are a widely researched group of enzymes, due to their application as green catalysts in the regioselective transesterification, hydrolysis or synthesis of acyl groups aiming to prepare fine chemicals and pharmaceuticals (Daiha, Angeli, de Oliveira, & Almeida, 2015; Kapoor & Gupta, 2012; Kumar, Dhar, Kanwar, & Arora, 2016; Liu, Zheng, Zhang, & Zheng, 2012; Lopes, Fraga, Fleuri, & Macedo, 2011).
Analytical determinations of lipase activity are usually carried on using p-nitrophenol (pNP) esters as substrates, such as p-nitrophenyl butyrate, p-nitrophenyl laurate, and p-nitrophenyl palmitate. The reaction is generally carried out in emulsified media. In one of the classical methods of lipase activity determination (Winkler & Stuckmann, 1979), the hydrophobic ester p-nitrophenyl palmitate is dissolved in isopropanol and then mixed with the buffer of choice, also containing gum arabic and sodium deoxycholate
as stabilizers. In this method, due to the excess of water, the equilibrium is shifted toward the hydrolysis reaction. Since then, several modifications to improve the method have been published (Hernández-García, García-García, & García-Carmona, 2017; Mayordomo, Randez-Gil, & Prieto, 2000; Pratama, Helianti, Suryani, & Wahyuntari, 2017).
After the enzymatic hydrolysis, pNP, as well as the carboxylic acid forming the original pNP ester, are released to the emulsion (Figure 1) and the released pNP is quantified spectrophotometrically measuring its absorption at 405-420 nm (Alcantara et al., 2014; Farnet et al., 2010; Stoytcheva, Montero, Zlatev, A. Leon, & Gochev, 2012).
In alkaline conditions, the phenol group releases one proton to the media to form a phenolate base (see Figure 2). Once formed, the phenolate base undergoes a resonance equilibrium (see Scheme 3). The unprotonated resonant forms absorb in the range from 400 to 420 nm (Biggs, 1954).
The first study describing the dependence of pNP absorbance on the pH of the solution (Biggs, 1954) found that there are two absorption maxima: the first one, in the 310-320 nm range occurs at low pH and is due to the presence of a completely protonated form, while the second one at around 420 nm appears at alkaline pH, due to the presence of a completely deprotonated form. The currently accepted value for the pKa for the pNP dissociation in water at 25°C (see Figure 3) was established as 7.15 (Serjeant & Dempsey, 1979).
Since dissociation constants are pH-dependent and may also be affected by temperature, it is necessary to access how pH and temperature may affect pNP determination in aqueous emulsions. On this regard, Prof. Colas group warned in 1999 that spectrophotometric determinations using aqueous solutions of pNP derivatives may be affected by both temperature and pH, and pointed out the importance of considering these effects on the absorbance of the released pNP (Fourage, Helbert, Nicolet, & Colas, 1999). In the present work, the pNP dissociation concept is revisited, by showing the interrelationship of pH, temperature and pNP absorbance in emulsified media, by means of three-dimensional plots. This work aims to offer a graphical update of the most fundamental concepts in the quantification of pNP emulsions for esterase and lipase researchers.
Based on widely used methods to prepare p-nitrophenyl palmitate emulsions (Gupta, Rathi, & Gupta, 2002; Orlando Beys Silva, Mitidieri, Schrank, & Vainstein, 2005; Palacios, Busto, & Ortega, 2014), a traditional emulsifying media for p-nitrophenyl palmitate was selected for this study. In this paper, the effects of pH and temperature in the absorption spectra of pNP dissolved in an assay mixture for the determination of lipase are discussed.
Materials and methods
Materials: p-nitrophenol (pNP), gum arabic, triton X-100, isopropanol, and sodium dibasic phosphate were purchased from Sigma Aldrich. Other chemical reagents were of analytical grade.
Solutions A. Buffered lipase assay emulsions: All Solutions A (buffer solutions) were freshly prepared by dissolving 550 mg of gum arabic in 1 L of the 50 mM buffer (for each corresponding pH) and mixing to achieve a complete solution. These solutions were 0.55 g/L gum arabic and 50 mM in the corresponding buffer.
Thirteen buffer solutions were prepared for this work: five 50 mM citric acid/disodium phosphate buffer solutions with pH of 4.00, 4.50, 5.00, 5.50 and 6.00; five 50 mM disodium phosphate buffer solutions with pH of 6.50, 7.00, 7.50, 8.00; and 8.50 and three 50 mM sodium carbonate buffer solutions with pH of 9.00, 10.00 and 11.00.
Solution B. Detergent solution: 1 volume of Triton X-100 was dissolved with 4 volumes of isopropanol and thoroughly mixed using a Vortex mixer.
Buffered pNP preparations: To achieve the final emulsion, 20 mL of Solution A (buffered lipase assay emulsion) were mixed with 2.00 mL detergent solution. This final emulsion was used as the solvent to prepare a 2.40 mM pNP emulsion (13 pH values from 4.0 to 11.0 were tested).
Analytical procedure: Aliquots amounting to 15 µL of pNP emulsion were placed in microplate wells, then 285 µL of the respective buffer was added to each well and the plate was stirred for mixing. The final pNP concentration in each well was 0.120 mM. All solutions and emulsions were freshly prepared prior to their use, pH in the microplate was assumed as the same value of the added buffer.
UV-visible spectrophotometric determinations were recorded on a Synergy™ HT Multi-Detection Microplate Reader. The pH values were measured at 25°C using a HI 2211 pH meter (Hanna Instruments).
At each pH value, three pNP samples were prepared as described above and absorption spectra ranging from 270 nm to 500 nm, with a 2 nm variation per reading, were run for each microplate well. Surfactant solutions without pNP were also analyzed to study their effect in the absorbance of this analyte.
At each pH, samples in microplates were progressively heated to reach each of 6 different temperatures: 25°C, 30°C, 35°C, 40°C, 45°C and 50°C. For each temperature, the mixture was previously incubated at the required temperature for 5 minutes before the spectrophotometric measurement. Overall errors in curves were less than 0.3%. Absorbance blanks were performed at different pHs and temperatures with only surfactant emulsion.
Graphical analysis: Data obtained from spectrophotometric measurements were analyzed in three dimensional plots correlating three of the following variables: temperature, pH, wavelength, and absorbance. Plots were made using Origin Pro 5.0 software (OriginLab, Massachusetts, USA).
Results and discussion
As shown in Figure 4, the absorbance maxima at 35°C and different pHs for the surfactant emulsifier were around 290 nm.
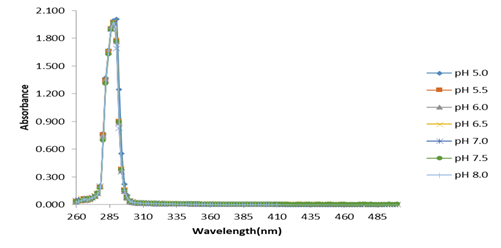
Figure 4 Absorbance spectrum from surfactant solutions at 35°C and different pH values. Source: own research.
Also, in Figure 5, it can be seen that at pH 5 and different temperatures, the absorbance maxima for the surfactant emulsifier were also around 290 nm. The fact that these absorbance maxima, at different temperature and pH conditions, do not appreciably change, can be considered as evidence of the surfactant emulsifier stability under these reaction conditions. These results showed that the emulsifying medium employed in lipase measurements does not interfere with the analysis of pNP in the different pH and temperatures tested, which can. Thus, triton X-100, isopropanol and gum arabic act as proper emulsifying agents for these types of substrates, due to their capacity to stabilize pNP esters in aqueous emulsions, their stability at different pHs and temperature conditions, and their null effect on pNP spectrophotometric quantification.
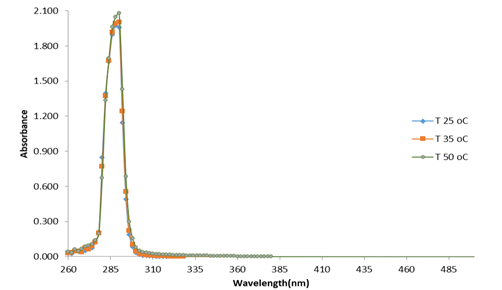
Figure 5 Absorbance spectrum from surfactant solutions at pH 5.00 and different temperatures. Source: own research.
As shown in Figure 6, at pH 6.00 and lower, the pNP absorbance maxima are in the 310 nm to 320 nm band, due to the relative abundance of the protonated form of this substance. On the other hand, at alkaline pH 7.00 and higher, due to the abundance of anionic form, the pNP absorbance maxima are in the 400 nm to 420 nm range. (Fourage et al., 1999).
At pH 6.00 and lower, there is a very low concentration of unprotonated yellow form of pNP, making it difficult to measure spectrophotometrically at 410 nm. Therefore, it would be more convenient to measure, at pH 6.00 and below, the UV absorbance at 317 nm as it has been reported in scientific papers related to the inorganic chemistry field (Shen, Sun, Zhou, Li, & Yeung, 2014; Tang et al., 2011; Zhang et al., 2013). However, this wavelength is not normally reported in lipase and biocatalysts research papers, probably due to the fact that it is close to absorbance maxima of the pNP esters. In the case of p-nitrophenyl palmitate the absorbance maximum, according to our experimental data, is at 300 nm but is has appreciable absorption at 317 nm (see Figure 7).
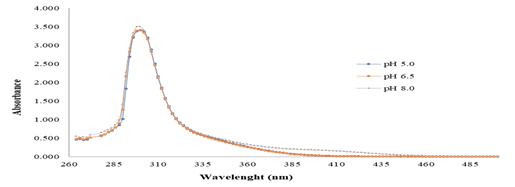
Figure 7 Absorbance spectra of p-nitrophenyl palmitate (pNPP) at 25 °C and three different pH values in a surfactant solution. Source: own research.
The results hereby discussed indicate that the pH has a more decisive role than the temperature in the absorbance of pNP. However, an appreciable effect of temperature on the deprotonated form of the pNP has been found. At higher temperatures, the absorbance at 410 nm from anionic pNP was favored, while the absorbance at 317 nm of the protonated form of pNP did not change. In Figure 8, the above-described effect of temperature at pH 6.50 can be noticed.
Figure 8 raises an interesting concern since temperature affects the absorbance of the deprotonated form of the pNP but not of the protonated one. Thus, the increase in absorbance with temperature seems not to be related to interconversion of protonated and deprotonated forms; but to a variation in absorbance of the deprotonated one. A possible explanation for this effect is that the resonant forms have different absorptivity and the higher temperatures shift the resonance equilibrium toward the forms with higher absorptivity. Possibly, the least thermodynamically stable resonance form of the deprotonated pNP, which might be the ketone form (d, in Scheme 3), is the most unstable at low temperatures. The instability of this contributor of the resonance hybrid may be attributed to two main factors, 1) it is the resonance
contributor that most affects the benzene ring stability, since it generates a system of four pairs of ( electrons on the ring, affecting aromaticity, a condition that could only be favored at high temperatures; 2) it places the double bond on nitrogen, an electronegative atom with a formal charge of +1.
In order to analyze the effect of temperature at a fixed pH value, two additional absorbance measurements were made in the range from 25 ° C to 50 ° C. One of them at pH 4.0 where there is a predominance of the protonated form of the pNP that absorbs at 317 nm and another at pH 8.0 where the anionic form of the pNP that absorbs at 410 nm predominates. As shown in Figure 9, it is possible to appreciate that under both pH conditions, where one of the two forms of the pNP predominates, the effect of temperature on the acid dissociation equilibrium of the pNP and therefore of its absorbance is barely appreciable.
Many analytical determinations using pNP release for enzyme-catalyzed reactions in aqueous solutions are based on reaction quenching by enzyme denaturation using alkaline reagents such as Na2CO3 or NaOH (Lam, Cortez, Nguyen, Kato, & Cheruzel, 2016; Sandoval et al., 2012). This is a useful alternative when
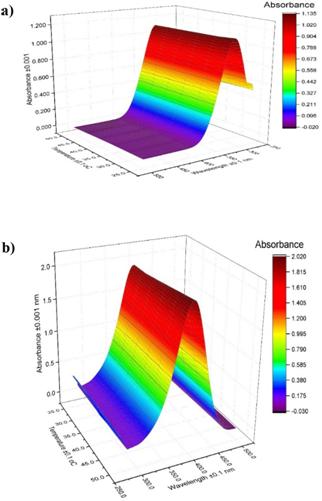
Figure 9 Temperature effect over pNP absorbance at a) pH 4.00, and b) pH 8.00. Source: own research.
the substrate is an alkaline stable compound due to reaching higher pNP dissociation (sodium carbonate solutions are about pH ( 11,20 and the pNP equilibrium is completely shifted to the dissociated form). However, p-nitrophenyl esters are easily hydrolyzed in moderate alkaline environments. Therefore, it is not recommended to determine the real pNP amount released in the reaction due to enzymatic catalysis.
Figure 10 depicts the kinetics of the spontaneous hydrolysis of p-nytrophenyl palmitate (pNPP) in buffer solution at pH 8.00. As it can be seen, lower pH values did not present absorbance at 410 nm which means high stability of these pNPP below pH 7.00. On the other hand, under alkaline pH conditions, the substrate releases pNP as a result of its spontaneous hydrolysis. Because of this, a good choice is the use of continuous determinations of the pNP in the spectrophotometer by continuous monitoring of the absorbance at different times, with no need of the alkaline quenching of the reaction (Sandoval et al., 2014), but keeping in mind that any analytical determination of pNP must require establishing the pNP absorptivity in the same pH, buffer and temperature conditions than the enzymatic assay. To this respect, a recent publication (Peng, Fu, Liu, & Lucia, 2016) proposed, as an alternative method, the use of the isosbestic point of p-nitrophenol at 347 nm, a stable
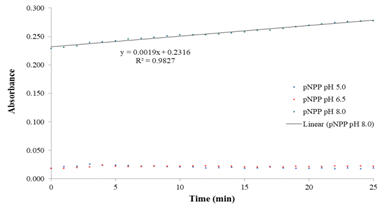
Figure 10 Spontaneous hydrolysis kinetics of pNPP in surfactant solution at 25°C and different pH. Source: own research.
wavelength, for the appropriate determination of this compound, since the absorbance of this chromophore remains without change regardless the pH. Another option for the accurate determination of the p-nitrophenol released by this reaction is the use of organic solvents (such as ethanol, acetone and other organic mixtures) as alternative quenchers, since they do not interfere in the analytical quantification of pNP and do not favor the hydrolysis of pNP esters (Lam et al., 2016; Palacios et al., 2014; Sandoval et al., 2012).
Conclusions
From the current study, the following conclusions are proposed:
Triton X-100, gum arabic and isopropanol are suitable emulsifying agents to stabilize aqueous suspensions of p-nitrophenyl palmitate. They have shown to be stable at different pH and temperature values, with absorbance ranges that do not overlap either the protonated or anionic forms of pNP and possess great capacity to stabilize pNP esters in aqueous emulsions.
According to experimental results, above pH 10.0 there is total deprotonation of pNP and under pH 3.5 there is total protonation of this compound. However, at pH values close to 7.0 there are varying amounts of both forms of pNP and, since many of the enzyme assays are carried out at pHs close to neutral, it is important to include strict pH and temperature controls for assay methods that release this substance as a chromophore.
The use of 317 nm to perform measurements of the protonated form of the pNP can be considered, but it should be kept in mind that this wavelength is very close to the maximum absorbance of the p-nitrophenyl palmitate -which absorbs at 300 nm- thus requiring a complex mathematical design to blank out the varying substrate contribution.
At pH 6.5, it was found that temperature affects the absorbance of the deprotonated form but not of the protonated form of the pNP. This phenomenon could be associated with electronic aromatic phenomena that might involve the resonance hybrid contributors of deprotonated pNP.
The p-nitrophenol esters, particularly p-nitrophenyl palmitate, are susceptible to spontaneous hydrolysis under alkaline aqueous medium. This leads the researcher to consider the pH value and the use of appropriate blanks for this type of substrates.