-
The Intergovernmental Panel on Climate Change (IPCC, www.ipcc.ch) published a report on land management, food security and GHG fluxes on August 8, 2019 (IPCC, 2019)[1]. The bottom line of this report is that practices such as sustainable and diversified farming, dietary change and increase in soil carbon content could have an immediate large effect on climate change mitigation and adaptation. These practices can also improve the effectiveness of efforts to control land degradation and increase regional food security. There is high confidence that actions to build individual and collective capacities and accelerate transfer of knowledge can bring social, ecological, economic and development co-benefits in the near future.
Within this framework, both school and community gardens represent beneficial strategies for optimization of resources and land use (e.g. López-Iturri et al., 2018)[2] as well as responsible practices for local environmental management (Anderson, 2012)[3]. The modern paradigm of urban gardening focuses on these spaces as living systems (Williams & Brown, 2013)[4] and thus, gardens could be interpreted as complex biosystems (Ong, 2017)[5] with direct implication on climate change action (Stevenson, Nicholls, & Whitehouse, 2017)[6]. Biofertilization as a practice, falls within that scope (Altieri et al., 2015).[7] However, as pointed out by Burt et al. (2017)[8], one key limitation to the potential effect of school garden practices is the low level of institutionalized and sustained programs. School gardens have been documented to be highly effective for the wellbeing of children. For instance, fruit and vegetable consumption have been observed to increase in children that have been involved in experiential activities related to local organic gardening (Jones et al., 2012)[9]. Similarly, children that have been exposed to school gardens have shown healthier Body Mass Index values and lower levels of fast food consumption (Utter et al., 2016)[10]. When the concept of school garden is coupled with organic practices, a larger effect has been recorded on cultural and food practices in the broader school communities (Ferguson et al., 2019)[11]. In this manner, exposure to gardens in the context of climate change has been shown to help increase cognitive abilities related with conceptual integration (Sellman & Bogner, 2013)[12] and these spaces have also been associated with higher levels of innovation in sustainability (Barrón Ruiz & Muñoz Rodríguez, 2015)[13].
One key challenge when studying the effect of school gardens and organic practices is that most of the published literature is centered on research based in cities in the United States (Guitart et al., 2012)[14] or developed countries (e.g. Browning & Rigolon, 2019)[15]. As such, a vast number of experiences in developing areas are still poorly documented. In Latin America, for instance, the full potential of school gardens as educational tools have been understudied (e.g. Castillo Echeverría, 2018[16]; Jiménez, 2018[17]), despite the fact that school gardens are fantastic spaces to construct different paradigms, such as sustainable farming as a rule, for the future (Cairns, 2017)[18]. In this manner, the present study was designed with the objective of studying a series of biofertilization treatments within a school garden in Costa Rica, to evaluate technical feasibility, as well as the role of organic gardening on the level of bioliteracy (through the STEM activity represented by the garden) as a proxy for climate change mitigation and adaptation perceptional change on children exposed to this project. This study can provide some insight on the role of small scale, community oriented, sustainable gardening within school premises and highlight the importance of knowledge transfer for true sustained efforts over time.
Materials and Methods.
This project was carried out in Escuela Las Américas, Turrialba, Costa Rica between 2017 and 2019. First, an unused area of approximately 43.2 m2 was selected (Fig 1A, marked with red rectangle). The area corresponded with an old easement where the topsoil had originally been removed to place water ducts that were covered back with the same material. With help from school workers and volunteers, large rocks were first removed, and the area was prepared for the garden and biofertilization project (Fig 1B). A series of 21 1x1m planting beds were set up in a spatial arrangement alternating experimental and non-experimental series of three beds in seven groups (Fig 1C, 1D). Each non-experimental series was used as normal garden space. The experimental series where used to set up a biofertilization experiment aimed to test the technical feasibility of using liquid vs solid organic fertilizer produced in the school. The garden was maintained in productive stage for 18 months (Fig 1E).
The complete experiment was replicated twice, at different times of the year, to control for potential environmental influence and to have two space and time-independent true replicates. During each one, four biofertilization treatments were tested. The first one was a solid fertilizer that corresponded with a simple compost made with the organic waste from the school kitchen and plant material recovered from maintenance of the school grounds. For this, all organic material was transferred twice a week onto a pile, alternately layering the food waste with dry grass clippings and leaves and a small amount of soil from the immediate area. After approximately two months, the decomposing material was mixed and transferred to a second pile where a second period of one month was used to produce the final compost used in the garden. At all times the compost was covered to maintain levels of humidity. The other three biofertilization treatments corresponded with liquid media that were made with the method of activated effective microorganisms recommended by AELBI (2010)[19] with three different fermentation times. With this method, leaf litter from a forest was combined with molasses and rice bran to reproduce microorganisms. The material was stored for eight days, in the presence of air, to induce the reproduction of aerobic microorganisms; and it was stored for one month in a sealed container, for anaerobic ones. After both periods, a 2:1 relation of anaerobic to aerobic material was combined with one part of molasses and 20 parts of water to produce a solution that was fermented for one, two and three weeks. These fermentation times corresponded with the three treatments used. The solutions were planned to be simultaneously ready and viable for biofertilization. All biofertilizers were characterized chemically and the number of colony forming units by volume (CFU/ml) was determined for liquid biofertilizers.
The experiment included two negative control, a blank one consisting of water and molasses but no active material, and a full negative where nothing but water was added to planting beds. In this manner, the complete design of the experiment consisted of six treatments, four functional and two controls. Treatment positions were randomly assigned in the garden space (Fig 3C) and in each bed a series of nine plants of either pepper (Capsicum) or cabbage-like (Brassica) cultivars were planted for a total of 650 plants, 325 of each type, during the complete experiment. In this manner, during each replicate, each planting bed had a unique combination of plant and fertilization treatments, and the position of the latter was not changed between experiment replicates to account for potential residual effects from the first run. A period of two months of experimental inactivity was given to the garden between replicates. During that time, lettuce and arugula were planted across all planting beds.
For each treatment, plant height and number of leaves were measured directly from plants on weeks three, six and nine. The number of fruits on pepper plants was counted at the end of each replicate for all plants and the fresh weight of both aerial and root structures as well as the length of the latter were determined for a sample of plants at the same time. Also, a basic chemical and structural characterization of the soil was performed, for each treatment, before the garden was established and after the experiment was completed. The conductivity of soil solutions and textural composition were measured directly from soil samples before the experiment started and at the end of each replicate as well. After 18 months of activity in the garden, the microbiological activity of the soil was established by determining soil respiration and incidence of dictyostelids. The former was calculated in a similar manner to Umaña et al. (2017)[20], whereas the Cavender method (see Sibaja-Matarrita et al., 2017)[21] was used for the latter.
In terms of the school garden, beyond the experiment, school children were considered the active participants of any social interaction and were allowed to participate in all stages of the experiment when they approached researchers to do so. This method followed the idea of the “scientist in the school environment” for STEM induction placement in primary schools (Choudry et al., 2016)[22]. Even though administrative personnel and teachers were briefed about the project, school children were not given any type of instructions and were simply allowed to approach researchers working in the new school garden. As such, the garden was used as the location for interactions, which following the work of Woods-Townsend et al. (2016)[23] could provide high educational value to the children.
To determine the impact of the activities in the garden on the school population, third grade children were selected for an assessment of basic knowledge on organic farming practices before the garden was established. All children in such level were assessed. This process was carried out by school teachers and consisted on a survey of six closed questions on a five-level scale in which a score of one corresponded with a full negative answer and a score of five with a full positive response.
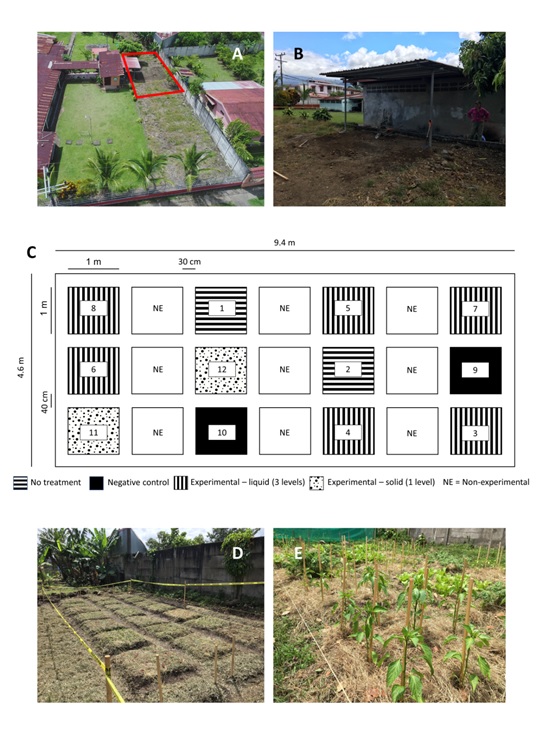
Source: Own preparation based on data recopilation above mentioned
Figure 1 Establishment of the school garden in Escuela Las Américas, Turrialba (A, B, D, E) and experimental layout (C) of treatments and controls for the biofertilization experiment conducted during this study.
The ordinal scale was represented by emojis in the original survey and students filled out the instrument by themselves in the classroom environment, without the influence of researchers. The questions shown to the student and intended to evaluate their level of bioliteracy on organic gardening activities were: 1) Have you heard of agriculture without fertilizers? 2) Have you heard the word “biofertilizer”? 3) Do you know what compost is? 4) Does your family separate waste at home? 5) Have you heard or seen the school garden? 6) Have you seen people working in the school garden? After the experiment was completely carried out, the same group of children, then in fourth grade, were requested to fill out the same survey, in the same manner. As such, the instrument worked to establish a pre/post quantification of potential impact of research activity within school premises. To account for potential differences with other institutions where researchers were not involved and no school gardens were present, fourth graders in two more schools within the same district were given the same survey around the same time as the post assessment. In the latter case, questions five and six were not considered.
After all data was collected, a series of statistical analyses were carried out. First, the evaluation of the biofertilization experiment was carried out using ANOVA/Tukey tests after normality was tested. This evaluation focused on differences in both soil and plant variables to determine if one or various fertilization treatments were associated with significant differences in soil quality and plant performance. Second, an evaluation of the effect of the school garden and the presence of researchers in the school was carried out by doing a pre/post evaluation of response values provided by the students. This task was performed by using contingency analyses and Chi-Square tests. Similar analyses were carried out when comparing responses from students in the experimental school and those in other institutions. All analyses were carried out in JMP, v 10, with a level of significance of 0.05 for the rejection of the respective null hypothesis.
Results
The school garden in Escuela Las Américas was successfully established in an unused easement where soil material had been mixed up several times before this project started. After rock removal and turning of the upper 40 cm of surface, the most important observed effect on the soil was an increment of 140% and 65% in available Potassium and Magnesium. Similarly, both Carbon and Nitrogen increased in 3% and 4%, respectively.
Liquid biofertilizers were rich in bacteria and yeasts with an average of 1.0X109 and 1.0X108 CFU/ml, and poor in fungi with an average of less than 1.0X103 CFU/ml. All nutrients were lower in the liquid biofertilizers, with a range between 1-12% the values of the solid fertilizer. The latter contained about 1% of Nitrogen and Phosphorus, about 0.30% of Potassium and Magnesium and approximately 12% of Carbon. The pH level of the solid biofertilizer oscillated around 7.5±0.1 whereas the values of the liquid fertilizers ranged between 2.7-4.3.
Brassica plants grew better with the 3-week liquid biofertilizer (see Table 1), but observed differences across treatments (e.g., F(5,318)=2.3, P=0.03 for height) were explained by the low height and low number of leaves on plants treated with the 2-week liquid biofertilizer. When the latter treatment was removed of the analysis, no differences in plant performance were observed (F(5,265)=1.6, P=0.15 for height). Capsicum plants showed better performance with the solid biofertilizer. Both height (F(5,318)=12.8, P=0.0001) and number of leaves (F(5,318)=16.7, P=0.0001) showed significant differences across treatments, and the lowest values were observed for the 3-week biofertilizer and blank controls. The number of pepper fruits after nine weeks was higher for the solid biofertilizer treatment(F(5,102)=4.9, P=0.0004), with an average of 1.6±1.0 fruits/plant contrasted with an average of 0.56±0.83 fruits/plant for the other treatments. No differences were recorded in root weight and length for any of the two studied plants across treatments. Fresh weight of the aerial Capsicum plant parts was higher for the solid biofertilizer treatment (F(5,29)=3.3, P=0.02) but no differences of fresh weight were observed for Brassica plants.
Most macro and micronutrients measured in the soil, increased with the establishment of the school garden (e.g., 23% increase in Carbon, see Table 2) and no differences were observed across treatments. However, levels of Copper and Iron decreased around 30 and 15%, respectively. Soil conductivity before the experiment was 325.0±8.4 mV, after the first replicate the value was 325.4±10.3 mV and after the second one was 297.1±11.4 mV, for a significant decrease of approximately 8% in 18 months (F(2,19)=18.7, P˂0.0001). The largest decrease of 16% was observed with the use of the solid treatment, which progressed from 336.0±7.0 mV before the experiment to 281.5±2.1 mV after the two replicates. In a similar manner, the percentage of sand particles increased significantly from 71.1±2.8% before the experiment to 72.5±7.3% after the first replicate and 79.2±4.3% after the second one (F(2,23)=3.7, P˂0.03). In this case, the solid biofertilizer showed the highest percentage of heavier particles at the end of the experiment with 79.8±5.0%, followed by the liquid 2-week biofertilizer with 77.1±7.1%. The lowest values of heavier particles were observed in the non-treatment beds with 73.7±3.5%.
Soil respiration values ranged from 720±280 mg CO2/g soil per day in no-treatment planting beds to 1420±395 mg CO2/g soil per day in those with solid biofertilizer. The latter treatment showed significantly higher values than any other treatment (F(5,30)=10.7, P˂0.0001). However, no differences were observed in the incidence of dictyostelids across treatments (F(5,11)=1.8, P=0.22).
Table 1 Averages (and standard deviations) of the plant growth variables measured over the nine weeks, arranged by plant type and treatment used as part of the biofertilization experiment. Bold values denote highest at respective time
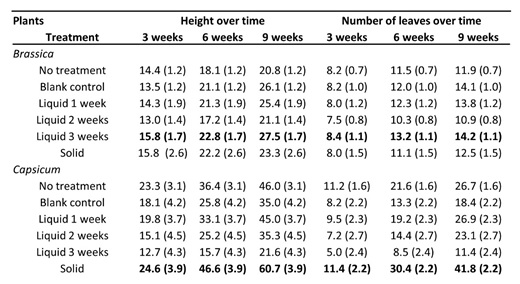
Source: Own preparation based on data recopilation above mentioned
Surveys carried out on school children showed that responses on some questions were different before and after the establishment of the school garden and carrying out the biofertilization experiment (see Figure 2). Differences were observed between the pre- and the post-garden surveys in relation with question number one, regarding whether or not students had heard of agriculture without fertilizers (2=13.7, d.f.=4, P=0.003) with a higher percentage of answers in stronger positive categories after 18 months of garden activity. A similar pattern was observed for questions three, about knowing what compost was (2=10.0, d.f.=3, P=0.01), five (2=27.8, d.f.=4, P˂0.0001); and six, about having observed people working in the school garden (2=22.6, d.f.=4, P=0.0001). No differences were observed in questions number two (Have you heard the word “biofertilizer”?) and four (Does your family separate waste at home?).
Table 2 Averages (and standard deviation) of soil variables measured before and after the biofertilization experiment, arranged by experimental treatment. Bold values denote lowest for each variable
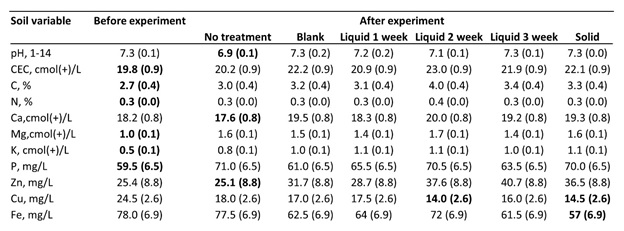
Source: Own preparation based on data recopilation above mentioned
Finally, when the post-garden responses of children in Escuela Las Américas were compared with those from children in other schools, differences were observed for the question “Do you know what compost was?” (2=17.7, d.f.=4, P=0.01) with a higher percentage of children responding more positively in the experimental school than in the other ones (78% vs 34%). For the question “Have you heard of agriculture without fertilizers?”, a higher percentage of responses in the strong positive category were documented for the experimental school (73% vs 53% in other schools) but the overall distribution of responses was not significantly different. The other two questions did not show any differences.
Discussion
The school garden implemented in Escuela Las Américas in Turrialba was successful at the two levels studied herein. This demonstrated that the practice of gardening in Costa Rican schools could be valuable for the promotion of sustainable practices among young citizens (e.g. under the scope of law 8591). However, as observed by Burt et al. (2017), a more institutionalized strategy of environmental practices in schools, such as green curricula, is still required for an effective effort that can be sustained over time. Realistically, this is the case for other environmental practices in local schools (i.e. unnecessary use of plastic), that depend of individual institutions and teachers, but are not mandated by the public system of education. In this sense, as pointed by the IPCC (2019), collaboration between stakeholders for the increment of collective capacities should lead such institutionalized effort. The Costa Rican government counts on a public policy plan for the integrated adaptation to climate change for the 2018-2030 period (Gobierno de la República de Costa Rica, 2018)[24], but its extent is still rather limited.
In terms of the biofertilization experiment, plant performance showed that both the solid and the more fermented (3-week) liquid biofertilizers were more effective for Brassica and Capsicum plants. Even though there was not one single treatment associated with better performance in both plants, it is important to highlight that for Brassica, no differences were observed among treatments when the 2-week liquid biofertilizer was not considered for analysis. This suggested that differences observed at plant performance by treatment in Brassica were based on poor values associated with one treatment and not on superior performance as it was the case of Capsicum plants. With those results and the evaluation of other indicators such as number of fruits and fresh weight of aerial plant parts, it was clear that the solid biofertilizer was the most effective treatment for the latter plants. For an implementation of a school garden and promotion of sustainable practices, the selection of plants may play a role on the effectiveness of the strategy since children seem to respond more positively when the garden is successful (e.g. Somerset & Markwell, 2009)[25].
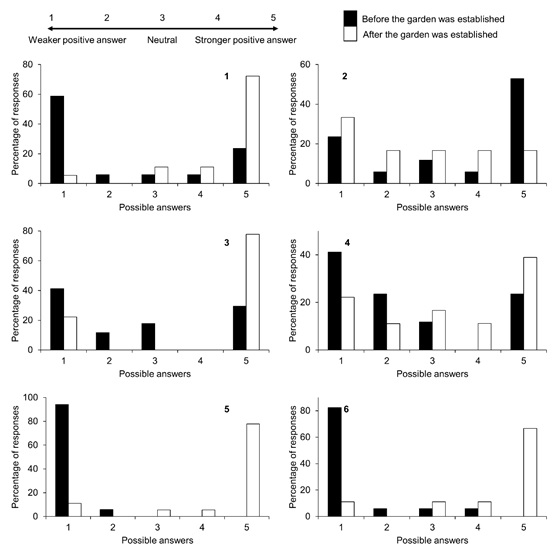
Source: Own preparation based on data recopilation above mentioned
Figure 2 Response patterns from selected school children to questions included in a survey before and after the establishment of the garden in Escuela Las Américas, Turrialba. For question numbers see Material and Methods.
The fact that the analysis of results at soil level also showed the positive significant effect of the solid biofertilizer made clear that such treatment was the best from the two analyzed perspectives. However, the implementation of biofertilization using solid media also has broader impacts. The carbon footprint of the liquid biofertilizers was necessarily higher than that of the compost, which was made with the organic waste from the school, due to the external expenses required for the generation of the former. The production of compost using these methods had the added benefit of reducing the overall waste produced by the school and promoting the practice of waste separation at the institutional level. Also, composting, as a sustainable strategy, is energy-efficient and can be generated with minimum equipment/facilities (Taiwo, 2011)[26]. Moreover, the observed 22% increment in soil carbon for the solid biofertilization treatment as well as the significant difference in soil respiration for the same treatment (which indicate more biological activity) necessarily link this strategy of biofertilization with global goals of climate change mitigation and adaptation (e.g. carbon increment in soils; see IPCC, 2019).
Interestingly, the public system of education in Costa Rica has included the topics of climate change and sustainability as mandatory subjects since first grade starting in 2016 (Ministerio de Educación Pública, 2016)[27] and sustainable agriculture is part of the science curriculum of primary schools since 2012 (Acta ordinaria N° 22, 2012). However, technical limitations in many schools and school districts due to the academic training of both teachers and school administrators (M. Nájera, personal communication, December 5, 2017) do not facilitate the integration of school gardens and climate change in simple curricular projects. This is ironic based on the fact that children exposed to school gardens have been documented to demonstrate higher awareness and sensitivity of sustainable processes through biophilic behaviors (Fischer et al., 2019[28]; Hand et al., 2017)[29]. That limitation, though, reveals one important area for technical improvement in the Costa Rican school system that could be addressed with proper training under the scope of a climate change and sustainability agenda.
Independent of the latter, it was highly valuable to observe that the response patterns of children to some of the questions literally switched between the pre- and post-garden scenarios. Of particular interest were the responses recorded on questions one (Have you heard of agriculture without fertilizers?) and two (Have you heard the word “biofertilizer”?), that albeit similar in construction, used different vocabulary. Those two questions were expected to show comparable response patterns due their similar conceptual idea, but the second one, that used the word “biofertilizer”, seemed to have confused children. Even though it was not expected for school participants to know the definition of the word, the more even distribution of responses after the establishment of the garden seemed more realistic and, in a way, promising. It has been argued that contemporary children have to adapt to new approaches of interaction with natural elements that also carry a new vocabulary (Kallis et al., 2015)[30] and in this way, exposure to new words is essential.
The effectiveness of the garden establishment in the school could be observed in those questions that showed significant differences. For example, it was very obvious that children knew the garden and the activity taking place in it after 18 months of researcher involvement (questions five and six). However, perhaps more valuable was the pattern recorded in question three, related to knowledge of the compost technique, that suggested a strong positive influence of the activity in the garden on the children´s knowledge. In fact, when children in other schools were asked the same question, the relative distribution of responses was different to those in Escuela Las Américas. Similarly, knowledge the children showed of agriculture without fertilizers was also lower in the other schools, suggesting that conceptual integration of elements through garden activities could have taken place, in a similar manner to other studies (see Sellman & Bogner, 2013). Whether or not such conceptual assimilation could have extended to the level of climate change adaptation and mitigation is an issue that required a more extensive documentation on the school population. However, as an observation, the establishment of the school garden promoted integrated discussions with teachers, administrative personnel and school children (those that voluntarily approached researchers) in the directions of sustainability, organic farming, climate change and good environmental practices.
In summary, based on the present study, compost could be recommended in Costa Rican public schools as a dual strategy of biofertilization and organic waste management. Further evaluations on compost innocuity could be performed as well but some data in this direction shows low risk (e.g., Camacho Céspedes et al., 2018).[31] School gardens can be highly valuable in Costa Rica for the conceptual integration of climate change and sustainability in the academic curriculum, and technical limitations at the school level could be addressed with training programs involving external stakeholders (i.e. higher education institutions). The establishment of the school garden in Escuela Las Américas affected positively the level of knowledge on organic gardening that children showed, and it demonstrated that this activity in the school has educational potential. Such activity with extended exercises on topics related to good environmental practices could be highly valuable in schools across the country.