Introduction
In Costa Rica and worldwide, there has been an increasing interest in the participating in and monitoring endurance running (Rojas-Valverde, 2019). This overcrowding has led to relatively uncontrolled participation from the point of view of planning and controlling body loads in runners, who often do not have professional advice to train or compete (Rojas-Valverde, Olicina et al., 2019). This lack of monitoring could trigger future physiological and physical complications, such as an increased potential risk of injury (Gabbett, 2016). That is why different devices have been created to maximize the performance and safety of athletes (Seshadri et al., 2017). The utility of wearable sensors to measure those factors that could affect the performance and safety of athletes has been developed. In this sense, variables such as muscle oxygen saturation, heart rate, sleep quality, and external load variables have been assessed to allow athletes and the general population to stay safer, perform better, and recover faster (Seshadri et al., 2019).
Understanding technology development and its impact on exercise performance are fundamental for any sport-related process (Cooper, 2006). Technology to monitor body load, also called wearable technology, is booming (Li et al., 2016). Particularly in running, these devices have become highly relevant and have been the subject of study in the last decade (Alexander et al., 2016; Gutiérrez-Vargas et al., 2018; Rojas-Valverde, Sánchez-Ureña et al., 2019).
This is the case of inertial measurement devices (IMU), which integrate multiple devices such as accelerometers, magnetometers, gyroscopes, and sensors that are linked to different positioning systems such as Global Navigation Satellite Systems, Geographic Information Systems, and Local Positioning Systems (Aroganam et al., 2019; Camomilla et al., 2018). These devices have previously been used to quantify variables such as impacts, accelerations, decelerations, and the magnitude of body load, among other variables in different group sports (Pino-Ortega et al., 2019, 2020) and individual sports (Gutiérrez-Vargas et al., 2018; Rojas-Valverde et al., 2020). Gómez-Carmona et al. (2019) described the most used IMU devices; the authors draw attention to known manufacturers such as Catapult Sports, RealTrack Systems, and StatSports, among others. Monitoring of these variables, called accelerometry-based external load indicators (Gómez Carmona et al., 2019), could be used to control physical load and potential exercise-related injuries (Colby et al., 2014; Rojas-Valverde, Sánchez-Ureña, et al., 2019). The ability to accurately determine injuries is of increasing interest due to the demonstrated relationship between runners with the greatest impact on footfall and the increased incidence of injury. The impact is characterized by a force impulse transmitted through the foot, with a short duration and, therefore, with high frequencies in running events (García-Pérez et al., 2014; Shorten & Mientjes, 2011). An optimal level of impact is appropriate to develop and maintain the health of bone tissue without causing overuse and producing basic physiological adaptations at the neuromuscular level. However, repetitive impacts generated at each step have been reported as a mechanical cause of stress fractures and kidney and muscle damage (Rojas-Valverde, Sánchez-Ureña, et al., 2019; Small & Relph, 2018; Vernillo et al., 2017). As a consequence, recent studies have focused on how to reduce the level of impact when running to bring about a better running economy (Cochrum et al., 2017; Quinn & Manley, 2012; Saunders et al., 2004).
Studies have shown that when runners are fatigued, their bodies are exposed to high-impact forces and, therefore, need to be monitored. The tibia has been reported as the most common site for stress fracture injuries in runners, with a prevalence between 35% and 49% of all stress runner injuries (Clansey et al., 2012). Shock waves generated during a race can be quantified by calculating the impact forces experienced in the tibia by accelerometry (Sinclair et al., 2018). Additionally, it has been explored how these forces can generate muscle and even liver and kidney injuries (Rojas-Valverde, Sánchez-Ureña, et al., 2019). That is why the quantification of variables, called accelerometry-based external load indicators (ABELIs), has been proposed to be considered as performance indicators in the running (Gómez-Carmona et al., 2019; Rojas-Valverde et al., 2020).
The application of electronic devices, as well as the subsequent treatment of the measurements obtained with them, in detection and action systems in the field of sports science and medicine, is of relevant importance. Technological advances allow athletes to be able to exploit 100% of their potential. The combination of sports talent and the most advanced technology allows for crossing borders that have never been crossed before. In this sense, understanding that the use of wearable devices in sports and exercise science to allow coaches, physiotherapists, strength and conditioning coaches, and sports scientists to better understand the physical demands in real time is a real concern and need (Seshadri et al., 2017). This study aims to systematize the practical experience of developing an inertial measurement device to quantify external load in real-time.
Methods
This section will describe the method and process to integrate and de velop an inertial device called SafeRun to quantify external load in runners, systematizing the practical experience of multi- and interdisciplinary professional personnel.
A multi- and interdisciplinary approach to IMU development
Constant inter- and multidisciplinary meetings were held to coordinate and redirect the project. Academic staff from the National University of Costa Rica (UNA) provided the basic guidelines so that the device had the technical characteristics necessary for it to be used in sports and exercise science and fulfill its final objective. Personnel from the Costa Rican Institute of Technology (TEC) made sure that all engineering parameters fully complied with all the requirements to make this device an easy-to-use and high-quality technological tool.
Finally, it was agreed to use a device that could quantify the external load of runners to control possible muscle damage and to be able to redirect, in real-time, the intensity and volume of exercises performed. The above considers ABELIs as load indicators for the pre-prescription of physical exercise. For this purpose, the ideal solution was found in inertial devices that adjusted to the established parameters: 1) An easy-to-use interface, 2) portable, 3) economically accessible, 4) with a wireless connection to any mobile device, and 5) which quantifies and processes variables in real time. To achieve the above, the planning of the present technology development project followed the Stage-Gate® process model, but understanding that traditional management techniques do not necessarily apply to non-traditional projects (Cooper, 2006). The present IMU development made specific modifications in order to adjust this planning to the project's particularities; the flowchart of the process followed is described in figure 1.
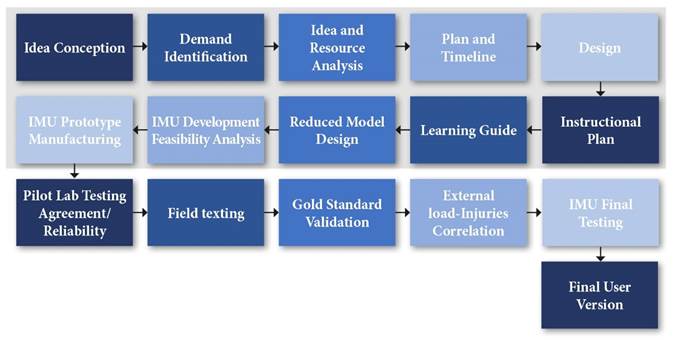
Figure 1 Schematic presentation of the IMU development plan. The gray background indicates phases already done by the project
Inertial Measurement Unit Integration
Obtaining absolute orientation required integrating three sensors in a single IMU device (BNO055, Adafruit Industries, New York): 1) A micromachined electromechanical system (MEMS) accelerometer (16g) that detects linear motion and gravitational forces (g); 2) A gyroscope (±2000, 20Hz) that quantified the rate of rotation in space (roll, pitch, yaw); and 3) a magnetometer (13g) that assesses the strength, direction, and relative change of earth's magnetic field at a particular location. The IMU has the following output: absolute orientation of 100Hz (360º, Euler vector, and quaternion), angular velocity vector (100Hz), acceleration vector using linear and gravity motion (100Hz, m/s2), magnetic field strength vector (20Hz, uT), and gravity vector (100Hz, m/s2).
These sensors were supplemented with some additional hardware. A processing plate (Feather M0 Bluefruit LE) and an incorporated Bluetooth® Low Energy (4.0, Bluetooth Special Interest Group, Washington, United States) transmitter for low-range communication using radiofrequency were selected to link the IMU and the mobile application. The processing plate had an ATSAMD21G18 processor and a microcontroller of 48 MHz (3.3v) with the following dimensions and weight: 68.6 mm x 53.2mm and 5.7g. The processing plate has 256K flash memory and 32K RAM.
The processing plate integration with IMU used the Adafruit BN0055. In the case of Bluetooth® Low Energy, the integration needed to use Arduino SAMD Boards and Adafruit SAMD. In order to monitor temperature drifts, an internal temperature sensor (1Hz, in degrees Celsius) was incorporated. Concerning the energy autonomy (~48h when turned on but stand by), IMU has a 1200 mAh rechargeable lithiumpolymer battery (3.7v PKCell, LP503562). The IMU was protected and sealed by a 3D printed hardcover made of acrylonitrile butadiene styrene polymer. The total dimensions of the IMU device were 35mm wide, 18mm height, and 80mm long, with a total weight of 36g. The spatial sensor distribution of the IMU is shown in figure 2.
Body Segment Attachment system
The IMU device was developed to be attached 3 cm cephalic from the dominant malleolus peroneus (Rojas Valverde, Sánchez-Ureña, et al., 2019). The anchorage was made with a neoprene band with a bag so that the device can be inserted easily; this neoprene band has a Velcro closure system so that it can be easily removed. This design allows freedom of movement and easy anchoring, helps skin injury prevention, and aids the skin breath and sweat.
This anatomical point was selected because it has been shown that ML is directly related to the increase in different factors of muscle damage during physical exercise, specifically during a race (Rojas-Valverde, Sánchez-Ureña, et al., 2019). The exact attachment point is shown in figure 3.
Mobile Application IMU Interface and Real-Time Monitoring
Capturing data in real time and storing it needed to develop a mobile application under the Android Operating System, but it could run in other systems, which connects to the device using radiofrequency under Bluetooth® standard receiving technology and intelligently fuses raw data from multiple sensors. It was developed using the Ionic 4 framework, an open code tool to create mobile apps of high quality and high performance. The mobile application prototype provides an interface to select and connect to the device; it is also capable of capturing, processing, and displaying the data received on an interface (see figure 4). As shown, it is necessary to link the IMU device through the mobile application.
This application connected to the device remotely, obtained the data in real time, saved the data extracted, and exported it in excel format. The deployment diagram is shown in figure 5.
Data Processing
The mobile application captured the data and downloaded it to a data sheet. The raw data was unprocessed at this step, but the manufacturer of the IMU introduced some data filtration processes depending on sampling frequency and output range. All these filtration processes (IMU chipset and accelerometer manufacturer) are usually applied before the raw data is available for the final user (Gómez-Carmona et al., 2019). At this time, the raw data was available for the user if some software filtration could be applied depending on the data usage.
Variables extracted
Three variables were obtained from the IMU; they are fundamental for quantifying external loads in sports, in this case in running (Rojas-Valverde et al., 2021; Rojas-Valverde, Sánchez-Ureña, et al., 2019). These variables were the number of impacts, footfall angle, and total accelerometry load (a(t)). The calculation of a(t) (au) refers to the squared root of the sum of the accelerations in three different axes (x, y, and z) (Rowlands et al., 2015; Staunton et al., 2018), representing the combination of the all three axis motion and it is obtained using the following equation (Gómez-Carmona et al., 2019):
Only the data greater than 1g is registered for a(t) extraction.
The second variable was the number of impacts made, greater than 1g (Gutiérrez-Vargas et al., 2018). This variable is represented in g forces (g). The footfall angle is a variable that calculates the initial angle (º) of the attached device with respect to the ground.
Pilot Testing: agreement and reliability
The agreement and reliability of the data obtained by the IMU were tested to confirm these statistical parameters. This procedure applied an incremental test as follows: start at 4.5mph and increments of 1mph every 2min until 8.5mph. The test was repeated twice to perform a test-retest agreement and reliability; it was performed with a physically active university student. The participant took rest between the sessions of the test for 24 hours.
The data obtained by the IMU are presented as means and standard deviation. The data agreement and reliability were performed following specific guidelines (Kottner & Streiner, 2011; Zaki et al., 2012). The over-time test consistency of the raw data was explored via reliability using the intraclass correlation coefficient (ICC) with the respective 95% IC. These results were confirmed via the linear correlation between measurements. ICC was qualitative interpreted following previous proposed ranking: 0 poor, 0.01-0.02 trivial, 0.21-0.4 regular, 0.41-0.6 moderate, 0.61-0.8 substantial and 0.81-1 almost perfect (Kramer & Feinstein, 1981). Bias and agreement between measurements were explored using the Bland Altman Plotting method. The results were compared using mean differences analysis between two measurements using t-tests. The magnitude of the significance was reported using Cohen’s d effect size (Cohen, 1988): < 0.2 trivial, 0.2-0.6 small, 0.6-1.2 moderate, 1.2-2 large, and 2.0-4.0 very large (Hopkins et al., 2009). Alpha was set at p< 0.05. All statistical analyses were made using special software (v.24, Statistic Package Social Sciences, Chicago, IL).
Results
The results obtained suggested a good agreement and reliability between the test. This shows that IMU outcomes are consistent in time. This is based on intraclass correlation, linear correlation, t-test values, and bias between tests from a total data set of 5892 data points for each variable and each test for a total of 35352 data points (see table 1).
Table 1 Agreement and reliability of data obtained from IMU.
Variable | Test | m (sd) | ICC (LA) | Bias | Correlation r (p value) | t-test (p value) rating |
a(t) (au) | A | 63871 .75(41572.33) | 1 (1; 1 ) | 1981 ,14 | 1 (<0.01) | 4,48 (<0.01I ) Large |
B | 61890.61 (40592.41) | |||||
Impacts (n) | A | 2561.7 (1538.23) | 0,933 (0.93, 0.936) | 33.5 | 0.935(<0.01) | 3.13(<0.01) Large |
B | 2528.2(1628.77) | |||||
FootfallAngle | A | 54.21 (20.82) | 0.394 | 127.6 | ||
B | 55.4(22.84) | (0.368, 0,418) | 45.22 | 0.394 (<0.01) | (<0.01)Very Large |
m: mean, sd: standard deviation, ICC: intraclass correlation, LA: limits of confidence.
Figure 6 shows linear behavior of increasing magnitude of a(t), impacts and footfall angle during both tests. Variables magnitudes tend to increase as speed also increases.
Recommendations and Future Applications
The information obtained from the IMU in real-time could be correlated with the level of muscle damage. The use of body loads and accelerometry-based external load indicators has been proposed as an innovative means of quantifying physical body loads. This could give runners a real perspective on physical loads and muscle damage and, in the future, give information to improve training processes such as planning and prescribing loads and preventing sports injuries due to overload.
It is necessary to explore software filtering for the raw data to have better quality signal depending on data usage. In addition, upgrading the IMU with an accelerometer that could assess actions with a high component of g forces should be considered. Therefore, the accelerometer should have more than 16g capacity or integrate another accelerometer with a wider range of measures.
Conclusions
The increasing trend and projection for the use of wearable sensors technology in the general population have led to the development of different inertial measurement devices to monitor activity and motion variables during exercise or sports. This shows how IMUs are influential in delivering a wide variety of information that could improve not only physical performance but could give information for the prevention of future complications such as injuries or overload. Developing sensors that can monitor external loads and give the information in real time to make opportune decisions could be an option to effectively redirect training or competition.
The development of this SafeRun IMU prototype by Costa Rican high education institutions, such as the Costa Rican Institute of Technology (ITCR) and National University of Costa Rica (UNA), is an example of how integrating knowledge of two different scientific areas could lead to great outcomes that respond to national, regional, and worldwide problematics.
The results showed an acceptable agreement and reliability of the data extracted from the IMU. This study has demonstrated the installed capacity of both institutions to integrate existing sensors available in the international market in a single device in order to program it and use it for different purposes. In this case, an IMU was developed with great success, capable of quantifying external load variables controlled from a mobile device in real time.
Intellectual property is in the process of a co-ownership agreement between UNA and TEC researchers to the extent that the results of the project are subject to legal protection. Also, the ownership of industrial property rights related to inventions that could be derived from the research project will be managed by the intellectual property units of each university. This device differs from other existing ones due to its small size and portability, which benefits its commercialization and massification. The preceding reflections confirm the quality culture of public universities in Costa Rica and show the fruit of joint work between institutions.
Funding
The author MM-S was funded by the Vice-Chancellor of Research (5402-1600-0401) of the ITCR and with a specific project budget of CIDISAD, Research and Diagnosis Center in Sports and Health of the National University of Costa Rica and SESLab, Electronic Engineering School of the Costa Rica Institute of Technology