Introduction
Symbiotic relationships between fungi and plants have drawn the attention of scientists since their initial description 140 years ago (De Bary 1879). Countless investigations have confirmed that most, if not all, plant species in natural ecosystems establish symbiotic relationships with endophytic microorganisms (Sun & Guo 2012). This diverse group of endophytes includes both fungi and bacteria, which are able to colonize the inner tissues of their plant hosts without causing any apparent damage (Bayman & Otero 2006, Bonfante & Anca 2009). The interactions established between endophytic microorganisms and plants can range from mutualism to saprophytism and have influenced the ecology, survival, and evolution of the host plants.
Endophytes seem to play a very important role in maintaining the structure and diversity of the plant community and, consequently, of the entire ecosystem (Laforest-Lapointe et al. 2017, Tao et al. 2008). However, despite their potential roles as promoters of orchid germination and development, endophytes have rarely been studied (Ma et al. 2015). This is striking considering the diversity of this group of microorganisms, containing over 110 genera, and the potential functional roles played by these fungi, among which stand out the promotion of seed germination and protocorm development in plants (Pant et al. 2017, Teixeira da Silva et al. 2015, Tsavkelova et al. 2008).
Regardless of being an old and highly specialized group, the Orchidaceae family still displays diversification and speciation processes (Otero, Ackerman & Bayman 2002, Zettler, Sharma & Rasmussen 2003). Orchid development depends on the establishment of symbiotic associations with fungi, which provide a source of nutrients, particularly during seed germination-a behavior known as mycoheterotrophy (Kottke et al. 2013, Riofrío et al. 2013, Yoder, Zettler & Stewart 2000, Zettler et al. 2003).
Pleurothallis coriacardia is a green orchid found in the high Andes of South America, which shows both epiphytic and lithophytic habits. According to CITES (Convention on International Trade in Endangered Species of Wild Fauna and Flora), Pleurothallis spp. commercialization should obey strict commerce regulations (CITES 1992). However, many of these orchid species are extracted from their natural environments and marketed illegally (Jiménez 2014, Pant et al. 2017), with only a few companies dedicated to their reproduction for commercial purposes. An effective alternative to avoid illegal extraction of orchids is their controlled propagation for horticultural purposes using germination media enriched with simple sugars and nutrients, such as PhytamaxTM and Murashige & Skoog (1962). However, it is also possible to take advantage of the natural functions played by endophytic fungi for orchid propagation and conservation purposes (Sharma et al. 2002).
Information concerning P. coriacardia ecology, distribution, and symbiotic relationships is scarce (Suárez et al. 2006, 2008, Suárez & Kottke 2016). Even though no experimental results are currently available, Pleurothallis spp. are considered very challenging to reproduce and grow under controlled conditions. Indeed, it has been shown that Pleurothallis spp. are dependent on insect pollination to achieve successful fruit development and to attain high seed viability, with reports of high inbreeding depression in natural populations (Borba, Semir & Shepherd 2001) and limited sexual reproduction (Cara Dona & Ackerman 2012).
Seeking to understand more about the ecological relationship between endophytes and epiphytic orchids, this work aimed to characterize endophytic fungi that naturally colonize the roots of P. coriacardia and to evaluate the endophytes potential as promoters of P. coriacardia seed germination in vitro.
Materials and methods
Study site.- Samples were collected in the Mazán Forest Reserve, located about 10 km east of the city of Cuenca (Azuay Province, Ecuador), at 02°50’S and 79°13’W (Fig. 1). This forest reserve occupies a total area of 2640 ha, ranging from 2800 to 3500 m in elevation. The annual precipitation in this region varies between 1000 and 2000 mm, with a temperature range between 6 and 12°C. Mazán Reserve is representative of the evergreen high montane forests and contains about 300 species of vascular plants, among which are 40 species of epiphytes, including orchids and bromeliads (Guzmán & Moreno 2014). The areas adjacent to the Mazán river are covered by both primary and mature secondary forests, with an important arboreal stratum represented by species such as “Arrayan” (Myrcianthes rhopaloides), “Romerillo” (Prumnopyts montana), “Sarar” (Weimannia fagaroides), “Pururug” (Hedyosmum luteunyii), “Tililin” (Piper andreaum), and “Jigua” (Ocotea sp.), among others. These trees foster the maintenance of a constant humidity and contribute to the absence of a marked dry season, creating a suitable place for the establishment of an important diversity of epiphytic species (Beltrán 2001).
Orchid species description.- Pleurothallis coriacardia Rchb.f. is an abundant species in Mazán Reserve, showing abundant wild populations in primary and secondary forests. Its growth habits are both epiphytic and lithophytic (Fig. 2). The most important morphological characteristics of this species, as recorded in the field include: i) sympodial growth, with unifoliar stalks covered in the base by purple bracts; ii) oblanceolate and leathery leaves, sub-petiolated at the base; iii) velamentous roots exhibiting a characteristic green-translucent color at the tip (Fig. 3A); iv) apical inflorescences with up to 5 flowers-exhibiting fused lateral sepals and yellow-colored edges-per stem; v) small and elongated petals, varying from brown/ reddish to yellow; vi) yellow to green lips and columns, with yellow colored pollinia (Fig. 3B); and, vii) apical capsules developing from each flower and containing thousands of pale-yellowish seeds of about 60-40 µm in length (Fig. 3C). The presence of asexual propagules emerging from the petiole, showing an abundant development of roots and new stems with no bulbs, was frequently observed (Fig. 3D). We also noticed the presence of numerous P. coriacardia protocorms, indicative of natural germination of the orchid seeds. Plant material.- From February to March 2016 root samples were collected from 35 different adult individuals of P. coriacardia colonizing either rock substrates, live phorophytes, or decomposing arboreal substrates. Living phorophytes were mainly M. rhopaloides, W. fagaroides and Ocotea sp., but also other less representative phorophytes were present. We selected five different sites, separated from each other by no less than 200 m, for collecting samples in the forest (Fig. 1). We took care to include orchids from at least one rock and three of the most representative phorophytes from each site. The samples consisted of several root fragments of approximately 3 cm in length, which included the green-translucent tips. Each sample was placed inside a sterile plastic bag and transported to the laboratory in Cuenca. Samples were refrigerated at 4°C for less than 24 hours after collection until fungal isolations were performed.
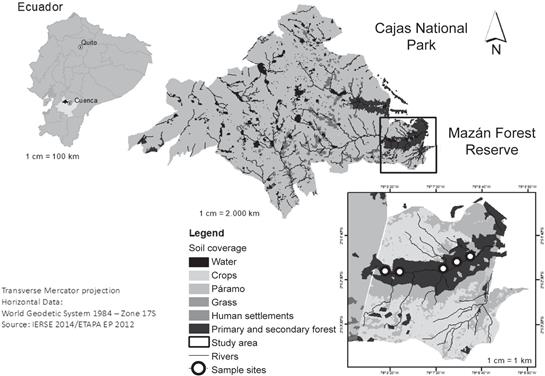
Figure 1 Map showing location of the study area in Mazán Forest Reserve, Cajas National Park, Ecuador.
Orchid root fragments were surface sterilized following a combination of the procedures of Currah (1987) and Zettler (1997). In brief, root fragments were rinsed with tap water to remove debris, and then submerged for one minute in a solution of 2% bleach: 70% ethanol: sterile water (1:1:1), followed by several washings with sterile distilled water. Roots were then cut into small pieces (<1mm) using a sterile scalpel, inoculated in molten fungal isolation medium (FIM) (Clements, Muir & Cribb 1986) that was supplemented with streptomycin (3 µg mL-1), and incubated at 19°C. The hyphae that emerged during the next 96 hours were sub-cultured several times in potato dextrose agar (PDA) (Latalova & Balaz 2010) until their purity was confirmed. The fungal isolates were classified based on their colony morphology, taking into consideration the mycelium color, texture, and pattern of growth. Pure cultures were preserved in oatmeal-agar medium (OMA) at 4°C (Zettler 1997).
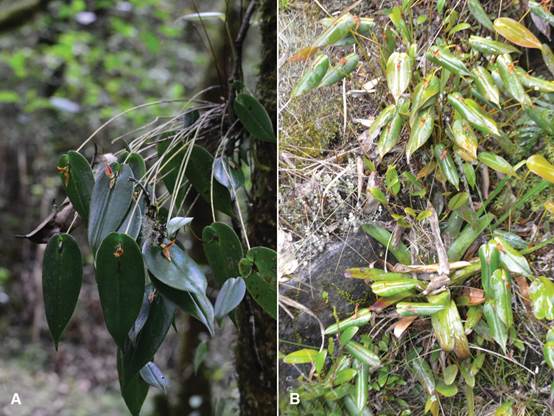
Figure 2 Pleurothallis coriacardia growth. Epiphytic (A) and lithophytic (B) habits. Mazán Forest Reserve, Cajas National Park, Ecuador.
Seed were harvested from mature, dry capsules of adult P. coriacardia individuals in their natural habitat (Mazán Reserve) at the same time that root fragments were collected. Seeds were processed less than 24 hours after collection. For this, the capsules were opened under sterile conditions and seeds were placed in sterile vials (one for each capsule) and stored at -20°C until used (Zettler & McInnis 1993). Prior to sowing, seeds
(of a single capsule) were disinfected using a protocol adapted from Zettler, Delaney & Sunley (1998). Briefly, seeds were immersed in a solution of 85% ethanol: 2.5% bleach: sterile distilled water (1:1:1) for one minute, followed by three successive rinses with sterile distilled water. Seeds were then suspended in 100 mL of sterile distilled water and transferred from the vial to the germination plates using a sterile syringe within ten minutes for the subsequent sowing in the germination plates, as described in the next section.
Selection of endophytic fungi for symbiotic germination assays.- In order to reduce the number of fungal isolates for symbiotic germination assays, we conducted a preliminary blind assay to select only those isolates showing a positive effect on the symbiotic germination of P. coriacardia seeds. For this, two milliliters of a suspension of disinfected seeds were spread on the surface of OMA plates. Then, in the center of each plate one block of agar (≈0.5 cm3) containing the mycelium of each fungal strain was inoculated. In total, we tested all 134 isolates twice. Uninoculated OMA plates were used as negative controls for germination. Plates were sealed with ParafilmTM and incubated in the dark at 19°C for two months; then, the plates were exposed to light under a 16/8 h light/dark photoperiod. Approximately ten randomly selected seeds in each plate were visually inspected with a stereo microscope (Olympus SZ61) every two weeks for five months.
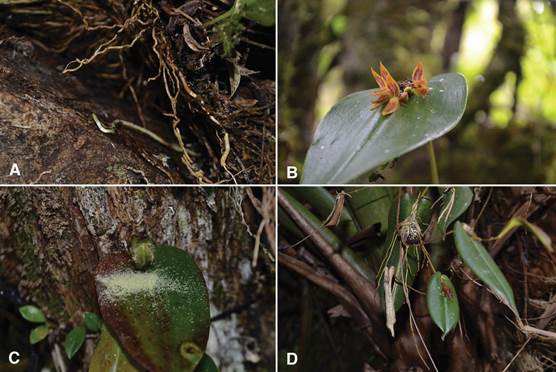
Photoghraps by L. A. Yarzábal (A) and G. A. Maldonado (B-D).
Figure 3 Pleurothallis coriacardia in the Mazán Forest Reserve. A. Roots of adult individual growing on rock substrate. B. Close-up of an inflorescence. C. Mature seed capsule of an adult individual growing on a phorophytes. D. Close-up of asexual propagules emerging from the stem.
The embryo development of each seed was scored according to a modification of the scale from Seaton & Ramsay (2009) as follows: 0=no germination/dead seeds; 1=seed with viable embryo; 2=swollen embryo; 3=testa rupture by enlarged embryo; 4=protocorm development; 5=appearance of first true leaf. The embryo diameters of 10 randomly selected seeds were recorded, per replicate and per treatment.
After obtaining the results of the preliminary symbiotic germination assay, genomic DNA was extracted from fungal isolates sharing the same macroscopic (color, appearance, consistency, size and shape) and microscopic (mycelium, hypha and conidia) characteristics. The 134 isolates were grouped into 76 morphotypes. DNA was extracted for polymerase chain reaction (PCR) amplification following the protocol of Cennis (1992). The ITS1-5.8S-ITS2 region was amplified by PCR using eukaryote universal primers ITS1 and ITS4 (White et al. 1990). The thermal profile was as follows: 94°C for 3 min, followed by 35 cycles of 94°C for 30 s, 57.4°C for 45 s and 72°C for 45 s, with a final extension step of 7 min at 72°C. The presence of amplicons was verified by agarose gel electrophoresis, using SYBR Safe DNA gel stain (Invitrogen, Carlsbad, CA). The amplicons were sent for further purification and sequencing to an external service provider.
To assign each fungal isolate to a particular taxon, we compared the obtained nucleotide sequences with those deposited in the GenBank database of the National Center for Biotechnology Information (NCBI 2017) and the UNITE fungal ITS reference database (Version 7.1) (Kõjalg et al. 2005, Nilsson et al. 2018) using BLAST (Basic Local Alignment Search Tool) (Altschul et al. 1997). Following a strict criteria, we provided genus names only to those isolates whose sequences exhibited an identity >95% to the reference sequence and with an E value lower than or close to 0.001. Isolates exhibiting lower sequence identities were labelled at the order- or family-level name, or referred to as ‘unknown’ fungi.
Symbiotic germination assays.- Seeds were surface sterilized as previously described and spread over the surface of OMA. Fifteen selected fungal isolates were then inoculated as described above, and the embryo development was monitored every four weeks for a total period of four months. The test was performed with ten replicates per fungal isolate. For a positive control of germination, seeds treated as previously indicated were spread on the surface of PhytamaxTM Orchid medium P6793 (Sigma-Aldrich) (pH 5.6), supplemented with gibberellic acid including the analysis of three different concentrations: 0, 10 and 20 µg mL-1 (Sigma Aldrich). This nutrient-rich medium is frequently used for asymbiotic germination of orchid seeds and was expected to provide the necessary nutrients to support germination of P. coriacardia seeds. The negative control of germination uninnoculated OMA as described above.
To score the seed development, we followed the same scale used in the preliminary assay, counting all seeds in each plate. To establish a relationship between seed diameter and developmental stage we only considered an average of 30 seeds, and conducted a Kruskal-Wallis non-parametric test and a sequential Bonferroni-corrected test (p=0.003) (Holm 1979). The same analysis was used to test whether there was a difference in the development rates of each stage with the different isolates and with the negative and positive control. Embryo development and seed diameter data were analyzed with XLSTAT (V. 2014.5.03) (Addinsoft 2014).
Results
In total, 134 pure fungal isolates were obtained from P. coriacardia roots and as previously stated, they were grouped into 76 morphotypes based on macroscopic and microscopic characteristics. Isolates were identified using BLAST searches.
Coprinellus (Psathyrellaceae) was the most frequently occurring genus in the entire collection (20% of the isolates), followed by Fusarium (Nectriaceae), Nigrospora (Trichosphaeriales), and Trichoderma (Hypocreaceae) (represented by 10%, 10%, and 8% of the isolates, respectively). The isolates originated from P. coriacardia individuals colonizing various substrates. We found 16 isolates originated from lithophytic plants; 82 from epiphytic individuals colonizing the most representative phorophytes (7 from W. fagaroides; 17 from Ocotea sp.; 30 from M. rhopaloides; 28 on other less abundant phorophytes); and the remaining 36 originated from P. coriacardia collected from decomposing substrates (Fig. 4). Information of the entire endophytic collection are detailed in Appendix 1.
Twenty-three out of 134 fungal isolates exerted a positive effect on P. coriacardia embryo development in the preliminary symbiotic germination assay (data not shown). The relationship between embryo diameter and growth stage was established from the results obtained in this assay in order to confirm the suitability of the scale used to determine the stage of development. As can be seen in Fig. 5, there were significant differences between the average embryo diameter of seeds at different developmental stages. Based on these results, we selected 15 fungal strains for the final assay and discarded strains that exhibited a systematic contamination of the growth medium during germination assays.
Isolates selected from this assay were identified as Ilyonectria (6MF3), Coprinellus (18.1MF3, 22MF2.1, 19MF1.4.1, 20MF3, 21MF1, and 22MF2.3),
Nigrospora (18.2MF2, 26MF1.4.1), Chaetomium (17MR4), Fusarium (29MF2.8), Trametes (33MF2.2), Trichoderma (3MF5), and Unidentified Endophyte (12.1MF3, 27MF3).
Three of these 15 selected isolates-6MF3, 18.1MF3, and 22MF2.1-significantly promoted embryo development after 12-weeks incubation, as compared to the negative control (Fig. 6). Noticeably, two of these fungal isolates were closely related to Coprinellus species. It is important to highlight that inoculation with eight of these selected isolates allowed the embryos to develop until stage 3, even though the percentages varied from 3% to 30% depending on the isolate.
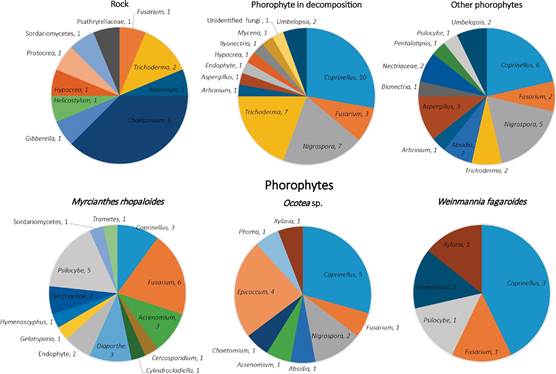
Figure 4 Distribution of endophytic fungi. In the upper panel there are three colonization substrates, in the lower panel there are three phorophytes substrates. The labels next to the fungus name represents the number of isolates.
Seeds of P. coriacardia sowed in GA- supplemented PhytamaxTM medium (included as a positive control of germination) produced some unexpected results: after 12-weeks incubation, all seeds reached stage 1 (viable embryo) regardless the concentration of GA, but there was no further development after that point. Surprisingly, 3% of seeds incubated in OMA medium (included as negative control of germination), reached stage 2 (embryo swelling) and then stopped developing. As can be seen in Fig. 5, the average diameter of the embryo was larger in seeds incubated in OMA medium (our negative control), when compared to those incubated in GA-supplemented PhytamaxTM medium (p=0.0105) (considered as positive control). We considered the experiment for 12 weeks, with a sequential revision of the plates, however, after this time some plates with developing seeds were reviewed but until week 16 we did not find evidence of changes in the development of P. coriacardia seeds.
Discussion
Our findings show that the cultivable fraction of endophytic fungi colonizing P. coriacardia roots was rich in species irrespective of the substrate type on which the orchids grew. The isolates belonging to the Coprinellus genus were the endophytic partners which are the most frequently associate to P. coriacardia roots. Furthermore, some of the isolates, such as those belonging to the Ilyonectria and Coprinellus genera, stimulated seed germination by promoting embryo development in vitro until testa rupture (stage 3). Endophytic fungi colonizing roots of orchids have been reported to have a crucial role in plant propagation, embryo development, seed germination, and protocorm nutrition (Rasmussen et al. 2015, Ma et al. 2015, Pant et al. 2017). For instance, Tolumnia variegata in the presence of Ceratobasidium have a positive influence in seed germination (Otero et al. 2005), Epulorhiza spp. promoted protocorm development until leaf production of Cyrtopodium glutiniferum (Pereira et al. 2015). Besides, Fusarium spp. have been described as active auxin- and gibberellic acid producers in Dendrobium sp., Pterostylis sp. and Cymbidium sp., promoting high germination rates and playing important roles in the development of protocorms (Tsavkelova et al. 2008).
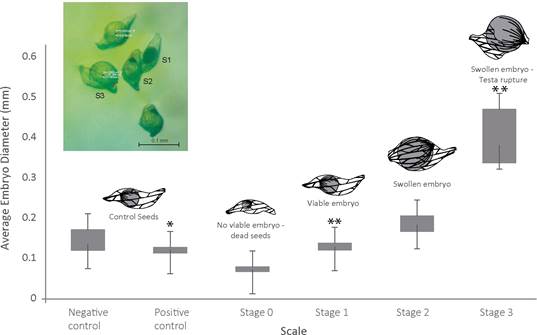
Figure 5 Diameter versus embryo developmental stage of P. coriacardia seeds. The schemes illustrate the morphology of the seeds at different stages. Inset: the picture shows seeds at different developmental stages, as seen under a stereo microscope, S1 represents Stage 1=Viable embryo; S2, Stage 2=swollen embryo; S3, Stage 3=Testa rupture. Average diameter identified with asterisks differ significantly from the negative control p < 0.001; the two asterisks is p < 0.01.
The root fungal endophytic communities of P. coriacardia and other green orchids in Andean ecosystems have been poorly studied. Greater research efforts have been made using massive sequencing techniques for understanding the mycorrhizal and endophytic fungi associated with green epiphytic Andean orchids like Odontoglosum pardinum, Epidendrum marsupial, Cyrtochilum pardinum, C. flexuosum, C. myanthum and Maxillaria calantha reported for the same study site (Cevallos et al. 2018, Guzmán & Moreno 2014, Herrera et al. 2018, Herrera et al. 2019). However, no information is available regarding the cultivable fraction of fungi for these species. We show high incidence of root endophytic fungi in adult plants of P. coriacardia from Mazán Reserve. We found that the orchids growing on live phorophytes-W. fagaroides, Ocotea sp., and M. rhopaloides-and over decomposing arboreal substrates were similarly colonized by fungi customarily defined as saprophytes and pathogens such as Coprinellus, Nigrospora, Trichoderma, and Fusarium. In contrast, orchids growing on rock substrate had a different composition in which we identified morphotypes closely related to Fusarium tricintum and Helicostylum, in addition to observing a greater abundance in individuals of the genus Chaetomium whereas fungal isolates closely related to Coprinellus were completely absent.
The fact that a fungus can be isolated from a section of root tissue does not mean it is mycorrhizal. Nonmycorrhizal endophytes, saprophytes, and pathogens are commonly reported in orchid roots (Bayman & Otero 2006, Bayman et al. 2016, Selosse et al. 2010) and members of this guild have shown positive roles in orchid development from seed germination to vegetative growth. The first non-Rhizoctonia-like genera reported to show a symbiotic role was Fusarium in the orchid Cypripedium reginae (Vujanovic 2000), with significance in seed germination. The known saprophytic fungi Mycena, Trichoderma, and Chaetomium have also been reported to play a specific role in orchid development (Pant et al. 2017); all of these genera have been reported in this study.
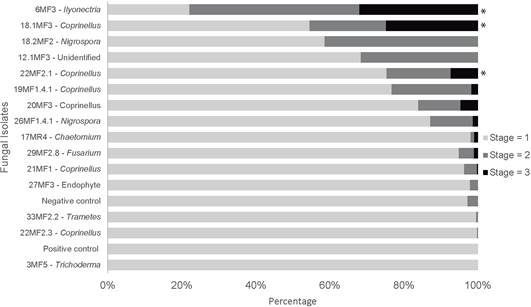
Figure 6 Percentage of P. coriacardia seeds that reached stage 1, 2 and 3 by week 12 of symbiotic germination in the presence of each fungal isolate. The percentage in each stage was calculated by dividing the number of seeds in each stage by the total number of viable seeds in each sample. The positive and negative controls of germination are also included. Treatments identified with asterisks differ significantly from the negative control p<0.001.
The genus Coprinellus, also saprophytic fungi, was first described as very frequently associated with the terrestrial orchid Epipogium roseum by Yamato et al. (2005), they suggest the existence of a highly specific relationship between this orchid species and members of the Psathyrellaceae family, owing to the high abundance of pelotons observed in their roots. Yagame et al. (2013) confirmed the mycorrhizal status of Coprinellus by symbiotic cultivation of seeds from Cremastra appendiculata, a photosynthetic terrestrial orchid, and confirmed the presence and mycorrhiza formation by Coprinellus in Cremastra aphylla (Yagame et al. 2018). Isolates belonging to this genus were detected very frequently in our study, a result which also confirm previous findings by Salazar et al. (2020). We also showed Coprinellus’s important role as a promotor of germination for this species, with two out of five tested isolates leading seeds to stage 3 (testa rupture) of development.
Previous studies about the germination of seeds from Pleurothallis orchids and symbiotic fungal associations are scarce. The only precedent to the present work is in relation to achievement for seed development of Pleurothallis truncata up to stage 4 after a 10-week incubation (León & Molina 2015) in Murashige & Skoog medium, supplemented with coconut water. Incidentally, the authors pointed out that attempts to achieve symbiotic germination of P. truncata seeds with the help of Rhizoctonia- like isolates were unsuccessful. On the contrary, we avoided the bias of selecting Rhizoctonia-like features through a preliminary blind germination assay, which probably lead to the unexpected discovery of an additional genus, Ilyonectria, that promoted embryo development of P. coriacadia.
Members of the Ilyonectra genus and their allied anamorphic taxa are often detected when studying rhizospheric communities of plants like Pyrola sp., Populus sp., Enkianthus sp., and Alnus sp. among others (Geml et al. 2014, Obase & Marsuda 2014, Unterseher, Per & Schnittler 2013). Even though there is not much information concerning the ecological role those fungi might play, recent findings support their functioning as promoters of germination of certain woody plant species (Bonito et al. 2014). The results presented here showing the promotion of P. coriacardia germination by Ilyonectria sp. strain 6M3 are in line with that hypothesis.
One particular result that deserves to be considered with caution was the slow rate of embryo development of P. coriacardia seeds in a GA-supplemented medium rich in simple sugars (PhytamaxTM). This is a widely used medium for in vitro asymbiotic germination of seeds from many orchid species, which was the reason we included it as a positive control of germination for our experiments. However, our results suggest that either PhytamaxTM is not well suited for P. coriacardia germination in vitro, or the germination of this species is particularly slow. We believe that the nutrient richness of this medium may be inhibitory to germination of P. coriacardia seeds, as previously demonstrated with other orchid species (Rafter et al. 2016). We are cautious to suggest a highly specialized relationship with P. coriacardia, despite our results, because we only isolated fungi from adult plants, and as it has been reported, mature plants are not implicated in the symbiotic germination of orchids seeds (Ovando et al. 2005). Besides, for epiphytic tropical orchids a low specificity condition between orchids and the fungal guests have been well documented in comparison to their terrestrial relatives (Jacquemyn et al. 2010, Johnson et al. 2007, Stewart & Kane 2006). We consider that the isolation of fungi from natural seedlings, probably using seed baiting techniques (Rasmussen & Whigham 1993), are necessary to confirm this association. It is important to point out that in the field it was common to observe protocorms and seedlings from epiphytic orchids, near to adult populations of P. coriacardia, located mostly in rock substrates, where we did not register Coprinellus genus.
After the 16-week analysis, no further development of P. coriacardia seeds was observed, which may be explained by various hypotheses. Halted seed development may be due to the necessity of another nutrient source not provided by the fungal endophytes (Zettler pers. comm. 2017). Another hypothesis is that fungal presence improved access to water (Ovando et al. 2005), considering the results of the negative controls, or that the fungi were used as an initial carbon source. Accordingly, the developing seed digested the fungi until it reached a large enough size to cause the testa to rupture, but after this point other obligate symbionts may be needed for establishment of the protocorm, and/ or possibly the fungi stopped acting as providers and became pathogenic, as in the case of Ilyonectria.
We hope our results could aid in improving descriptions of the ecology of Andean orchids and their endophytes and to support propagation programs of P. coriacadia both for conservation and commercial purposes which could in turn help reduce extraction from their natural habitats.