Services on Demand
Journal
Article
Indicators
-
Cited by SciELO
-
Access statistics
Related links
-
Similars in SciELO
Share
Medicina Legal de Costa Rica
On-line version ISSN 2215-5287Print version ISSN 1409-0015
Med. leg. Costa Rica vol.39 n.2 Heredia Sep./Dec. 2022
Original research
Beta-amiloid precursor protein ( β-App) and diffuse axonal damage after head injuries: a forensic point of view
1Servicio de Patología Forense. Instituto de Medicina Legal de Galicia. Santiago de Compostela (España)
2Departamento de Ciencias Morfológicas. Facultad de Medicina. Universidad de Santiago de Compostela (España)
3Departmento de cirugía y especialidades médico-quirúrgicas. Facultad de Medicina. Universidad de Santiago de Compostela (España)
β-Amyloid Precursor Protein (β-APP) is a membrane glycoprotein and a common component of neurons. It is involved in adhesion and cell growth processes after traumatic events. It is carried by anterograde fast axonal transport, and it accumulates inside neurons when the cytoskeleton is damaged. This is a vital biochemical process that consumes energy.
β-APP is not specific of traumatic events. It accumulates in any case of axonal damage, whatever its cause may be, like hypoxia, metabolic disorders, and any other circumstances that lead to brain swelling and intracranial pressure rising and in consequence to Diffuse Axonal Injury (DAI). In this study we review the expression of this protein in cases of traumatic brain injury with different chronological evolution.
The damage of cytoskeleton due to proteolysis in addition to the disturbance of kinases and phosphatases increase the permeability of the membrane. Calcium gets into the cell and activates calmodulin, thus neurofilaments compact, microtubules disappear and spectrin breaks. This disruption of the cytoskeleton has as consequence that the transported substances accumulate in the most affected areas by DAI. At the end of this process axon breaks, which is known as secondary axotomy.
The study of the accumulation of β-APP is useful to assess the extent of DAI and to determine the time elapsed after trauma or another insult to CNS.
Key words: brain injury; immunohistochemistry; beta amyloid precursor protein; diffuse axonal injury; traumatic brain injury dating
Introduction
Head trauma is one of the leading causes of death and disability in developed countries, above all in population aged under 45. This is partly due to traffic accidents. In fact, 25% of violent deaths are related with traumatic brain injury (TBI).(1,2)
On the other hand, it is important improve the diagnosis and prognosis of TBI, even though mild head trauma, because it involves serious long term secuelae, like neurodegenerative illness as Alzheimer and Parkinson disease. (3,4,5,6)
Nowadays, the most part of the studies about this issue are experimental in animals or they are clinical series with a diagnosis or prognosis perspective, especially those about β-APP, PGFA, and SB100 protein (7,8). There are also some retrospective studies based in autopsies, which relate behavioral alterations with the expression of certain markers like Tau protein or the apolipoprotein E (APO E) (9,10). Others highlight the relationship between the expression of β-APP and posttraumatic encephalopathy (11), but all of them have more clinical relevance than forensic.
Argentic techniques are still useful in obtaining interesting forensic, morphological, and functional information because they can show how the density of synapsis changes after head trauma. (12) However, immunohistochemistry has demonstrated being a more efficient method to study nervous tissue because it is more sensitive and specific to the study of each cellular type separately, and it is an easier laboratory procedure. (13)
Specifically, the β-APP reveals biochemical changes inside the neuron after acceleration and deceleration phenomena and torsion, which affects to the cellular membrane permeability.
β-APP is a transmembrane glycoprotein present in neurons. It seems to take part in cell adhesion, cell growth promotion, and autocrine processes, and it act as a binding site for some substances, like heparin. It is carried by fast anterograde axonal transport, and it accumulates after axonal cytoskeleton damage (14,15,16). It has been seen in neural soma and in damaged dystrophic neurites in damaged areas of the brain after trauma. When its expression begins to decrease in neurons, it is weakly expressed in microglia/macrophages (double stained with CD11b and β-APP) as a result of the phagocytosis of damaged axons (15).
The accumulation of β APP is not specific of trauma. It also takes place in axonal damage due to other causes like hypoxia, metabolic issues and all circumstances that lead to brain swelling and the increase of intracranial pressure. It means that its expression is a common response of the nervous tissue after any kind of insult. β-APP accumulates in neurons after the proteolysis of cytoskeleton. This fact, in addition to the alteration of the kinases and phosphatases, increases the permeability of the membrane. Therefore, calcium gets into the neuron and calmodulin becomes more active, then the cytoskeleton collapses due to the crush of neurofilaments, the loss of microtubules and the fragmentation of spectrine. Thus, axonal transport is interrupted and the materials that need it get accumulated in damaged areas until axons undergo secondary axotomy (17,18)
On the other hand, Staal and cols (19), proved the parallelism between the accumulation of calcium into the cell and the expression of β -APP. It is believed that the accumulation of BACE (b-secretase), PS-1 (presenilin-1), activated caspase 3 and CCA (caspase-mediated cleavage of APP) with β-APP in damaged axons in cases of diffuse axonal injury is due to the same mechanism of production of β-APP after the increase of membrane permeability, getting pathologically accumulated (20).
Actually, it has been highlighted the relationship between the presence of this protein and some neurodegenerative diseases like Alzheimer and Parkinson. The vascular changes and the hypoxia secondary to inflammation and intracranial pressure (ICP) rising activate beta and gamma secretases, which change β-APP into β-Amyloid plaques (AP). This activity seems to start four hours after the insult, and it is directly related with posttraumatic Alzheimer disease (10).
Likewise, chronic inflammation after TBI (unique or repetitive) promotes all this process, although there are lots of patients who accumulate the protein but finally do not undergo Alzheimer. All this suggests that it is necessary some kind of individual susceptibility, maybe a genetic kind of it. (21,22)
It may be hypothesized that further investigations about the evolution of β-APP into AP could be useful since a forensic point of view, relating deferred deaths with previous head trauma (unique or repetitive).
In this study we settle the usefulness of this marker in daily forensic work conditions, helping us to solve issues of great legal importance about diagnosis and date of TBI.
Material and methods
We took a series of 25 medico-legal autopsies: the cause of death of 21 was TBI and the other 4 cases were sudden cardiac deaths. We choose sudden deaths to avoid agonic phenomena that may cause brain hypoxia which could be misdiagnosed as a positive case. In the same way, patients with clinical record of ictus, seizures hypo or hyperglycemia were also rejected.
Our series consisted of 11 men and 10 women aged between 33 and 94. The time elapsed since trauma to death ranged between few minutes and 218 days.
Cases were divides into three groups: survival period shorter than 2 hours; survival period between 2 and 24 hours, and survival period longer than 24 hours.
Sampling was carried out not later than 24 hours since death, meanwhile the corpses remained refrigerated at 4ºC. In every case a complete autopsy was carried out paying special attention to macroscopic lesions that could involve TBI (skull fractures, intracranial hemorrhages, and iatrogenic procedures). Clinical files were thoroughly studied in those cases that had received medical assistance.
Once the brain has been extracted and weighted, we made 1-1,5 cm wide slices and obtained samples from contusion areas. These samples were fixed in 10% buffered formaldehyde during no more than 24 hours, then they were routinely included in paraffin as usual. Sections of 4μ were obtained and laid in silanized slides (Dako, Glostrup, Denmark).
Previous to the immunohistochemistry, samples were routinely stained with Hematoxylin-Eosin: After remove paraffin and hydration, sections were submerged in xylol, ethanol 100º, ethanol 96º, ethanol 70º, water and finally hematoxylin for 5 minutes, then they were washed with water and stained with eosin for 3 minutes.
Immunohistochemistry technique
First, antigen retrieval was carried out by heating the slides in a microwave oven for 20 minutes at 750 W in Tris-EDTA buffer (Tris 10mM, EDTA 1mM, pH9). After washing in PBS (Buffered saline phosphate, 100 mM phosphate pH 7,2; 150 mM NaCl), sections were incubated consecutively in: 1) primary antibody (1h) β-APP (Invitrogen, CA, USA, 1:50); 2) Peroxidase blocker (Dako) 10 minutes, 3) EnVision dual rabbit, mouse (Dako) 30 min, 4) diaminobenzidine. Liquid DAB+Substrate Chromogen System (Dako) 10 minutes as commercial instructions recommend (50 mL DAB in 1mL of substrate buffer). After each step, sections were washed twice during 5 minutes with PBS, and after DAB, they were washed with distilled water.
Solutions of primary antibody β-APP were prepared with a commercial antibody diluent (Dako). In every case, negative controls were included, in which primary antibody was replaced with PBS. At the end sections were counterstained with Harris Hematoxylin for 30 seconds.
Results
The macroscopic findings were wide ranged: brain swelling, petechiae in cortex (due to direct contusion or even to backlash), in cases of mild trauma. All kinds of intracranial hemorrhages in cases of severe head trauma.
In those cases of severe trauma, microscopic changes in the parenchyma were observed just with Hematoxylin-Eosin staining: eosinophilia, which means demyelination and necrosis; neurons with disorganized nucleus, eosinophilic lumps in the cytoplasm and surrounded by a retraction ring (phantom neurons); other neurons which showed nuclear changes that suggested apoptosis and some areas of gliosis
The quantity and extension of these findings depended on the time elapsed between trauma and death. Nevertheless, these changes found with conventional techniques were not classified, because they were carried out as a preliminary study for the selection of cases.
Immnohistochemistry
Control group: Whereas only patients who did not suffer any kind of neurological injury were selected, all of them were not stained with our marker.
Problem group: β-APP was positive in all cases to a greater or lesser degree. The staining intensity ranged since little pale spots scattered all over the cortex, to beaded axons and retraction bulbs in the white matter. These differences were clearly related with the survival period after the insult. (Table 1).
Table 1 Evolution of the expression of β-APP depending on survival period.
Shorter tan 2h | 2-24 h | Longer tan 24 h | ||
Scattered somas | WM* | |||
GM** | + | ++ | ++ | |
* | ||||
Beaded somas | WM | + | ++ | |
GM | ++ | |||
Retraction bulbs | WM | -/+ | ++ | |
GM | ||||
Pettern | WM | Diffuse | ''Z'' | |
GM | Diffuse | Diffuse |
* White Matter
** Gray Matter
The most common pattern was that multi focal diffuse, but there were cases with a mixed pattern: some regions showed a ''Z'' pattern mixed with others that showed a multi focal diffuse one
In those cases of deferred death which means that the survival period was very long (weeks or even months), this marker becomes negative, meanwhile astrogliosis gets spread. (Fig 1)
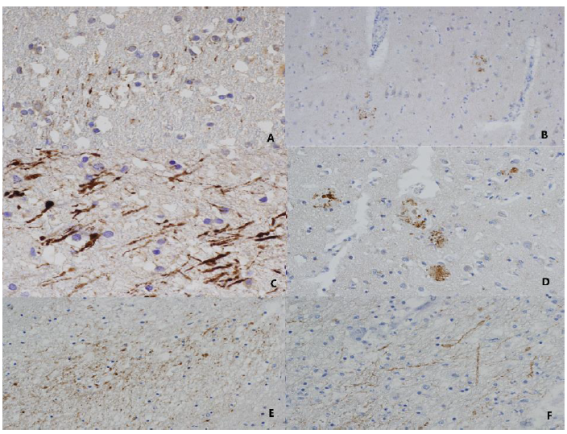
Figure 1 A: ''Z'' pattern in a case of 30-60 min. of survival. Gray matter. 40X. B: Multifocal pattern in a case of 2 h. of survival. Gray matter. 10X. C: ''Z'' pattern with swelling and beaded axons. Distal axonal bulbs. Five days of survival. White matter. 40X. D: Multifocal pattern. 26 days of survival. Gray matter. 20X E: Region of multifocal expression of β-APP in a case of mixed pattern in a case of 17 days of survival. White matter 20X. F Region of ''Z'' expression of β-APP in the same case of 17 days of survival. White matter. 20X. The staining is more intense and the changes in neurons and axons progress as the survival time is longer. In the case of 17 days of survival it can distinguish primary DAI from secondary damage thanks to the different patterns of expression in different areas of the brain.
Discussion
Our first results are similar to what different authors observed (14,15,16,17,18), because this marker is positive only in those cases that on went any kind of neuroinflammation, and it is totally negative in the control cases in which there were not any neurological insult involved in the physiopathology of the cause of death.
In the same way as Onyszchuk (23) Oehmichem (24) and Willemsee-van son (25) , in those cases with a long period of survival (months), we obtained negative results, meanwhile the glial scar progressed. That is why patients that remained in hospital during weeks or months after trauma were rejected, and because the cause and circumstances of death are well documented, so there are not any medico-legal issues to solve.
Distributions patterns of the expression of -app
First is important to know that amyloid pathology is different in each case of TBI because it is spatially heterogeneous depending on the sort, intensity, and location of the impact.
A simple but very practical classification of TBI is that one which divides it in focal or diffuse.
Focal TBI involves injuries that affects the cortex, which means head trauma with intracranial hemorrhages and/or rise of intracranial pressure.
Diffuse axonal injury involves (DAI) is consequence of rotational forces secondary to acceleration and deceleration which causes axonal disruption in the white matter. (26)
All this suggests that the kind of insult plays a very important role in the process of β-APP accumulation. (27).
In fact, experimental models that cause DAI using rotational forces at different and controlled radians per second, observed different focal and chronological expression of amyloid proteins in each group (28). Likewise, there are significant differences in the results of studies that use weight-drop model and those that use fluid percussion model (22).
On the other hand, hypoxia per se is not a cause of axonal damage but it is a consequence of vascular complications due to the rise of intracranial pressure and brain herniation. Given that β-APP transport is an active process (it consumes energy), it seems reasonable to assume that it accumulates also in cases of metabolic issues, as hypoglycemia, global hypoxia, infections and brain swelling. (29) This is why the assessment of β-APP allows for the diagnosis of the increase of intracranial pressure even when it is not high enough to cause herniation. The origin of this situation (cardiac arrest, CO poisoning, metabolic issues...) can be determined by the clinical context. (30)
Given that the accumulation of this protein is not a consequence of a unique injury mechanism, some authors (3,29) have searched for features that are unique to each type of injury. The pattern of distribution of β-APP can be useful to avoid false positives of traumatic causes of death. These authors have described three patterns:
Diffuse multifocal pattern: It is characteristic of axonal swelling in directly damaged areas and in injured areas by backlash, and of brain swelling in severe head trauma.
Zig-zag or Z pattern: It is seen in perivascular zones at the edges of an ischemic or infarct area. )
Mixed pattern: It shows groups of axons with diffuse damage mixed with areas of Z pattern due to the hypoxia secondary to the increase of intracranial pressure. This is characteristic in structures of encephalic midline mainly, in corpus callosum and pons, although they can also be seen in cortex.
It is mostly assumed that diffuse multifocal pattern of axonal varicosities is related with a traumatic cause of brain injury; the Z pattern is related with hypoxia and mixed pattern is associated with severe trauma that gives place to brain swelling or respiratory distress that adds a hypoxic damage to trauma.
This is consistent with our results: we observed how change the pattern of expression (especially in the gray matter) as the time of survival is longer, since inflammation and brain swelling take a minimum time to begin.
Onyszchuk et al. (23) settled a scale of intensity of staining related with the severity of trauma in cases with a survival period not longer than 2 h. It ranged since no staining at all, until mass staining of axonal bulbs. In grades 0-2 the immunohistochemistry could be very weak and may be due to ischemia. It is the time elapsed till death who determine the evolution of DAI and consequently the staining grade. When the survival period is shorter than 35 minutes, the intensity of staining is not more than a grade 2.
Our findings are not about the extension of the secondary brain damage, because we do not work in experimental conditions, but in daily forensic work conditions. Therefore we only sample the region of direct impact and thus we are not able to diagnose the extension of hypoxia which is probably that Onyszchuk observed. This point is relevant for clinicians, but it is not for postmortem diagnosis, so, given that the earliest changes occur in the gray matter, the study of this region is useful to relate the cause of death with mild head trauma where there is not an obvious macroscopic lesion. All this means that the presence of β-APP allows solving diagnosis questions when it is considered under its clinical and forensic context, as other authors also settled (31,32).
In fact, we were able to diagnose which head trauma was responsible of the death in one case of our series. At first, this case was included in the group with a survival period longer than 24 hours (five days), since the patient fell accidentally and on went a head trauma with neurological symptoms (unconsciousness), but she declined medical assistance. Five days later, she fell again and suffered another head trauma, but this time there was nothing wrong with the neurological assessment (Glasgow scale assessment 15). Shortly after meanwhile she was on going a CT, the Glasgow scale descended to 3 and she died some minutes later.
The CT showed an occipital skull fracture and a fatal intracranial hemorrhage. The first head trauma was clinically more serious, so the diagnose was a subacute subdural haematoma and therefore the first injury was considered the cause of death. Nevertheless, when we studied the brain with -APP, the pattern of expression was consistent with an evolution no longer than 2 hours. There was not any secondary axonal disruption that may suggest any rise of ICP and secondary hypoxia. All this pointed to an acute hemorrhage and death was a result of the second trauma. In this case there was not any legal consequences, but this assessment could be crucial in murders or accidents.
On the other hand, it is said that it is necessary an all brain wide sampling to diagnose DAI and see concomitant morphological changes that may suggest ischemia as eosinophilia and neuron necrosis in the region C1 of the hippocampus, which is the most sensitive region of the brain to hypoxia (33). Other authors conclude that it is necessary, at least, obtain 6 samples of the cortex of both hemispheres to diagnose DAI. (34)
Nevertheless, the study of samples from the contusion area is useful to determine the severity of trauma and the survival period, and it does not require special training or equipment, what allows gather them even in adverse conditions. In addition, the assessment of the contusion area has a high confidence level in forensic diagnosis, because the changes in this zone are more obvious and significant since this point is the ''ground zero'' from where the damage becomes widespread. (35)
Chronology of the β-app expression after trauma
It must be considered that DAI and protein accumulation are processes that take place in two phases: in the acute one, the insult provokes axonal disruption, especially in the cortex. This triggers protein accumulation. In the chronic phase, vascular changes difficult the clearance of these proteins, thus close this circular process of DAI which gets spread in regions not directly affected by injury. (27)
It has been established that there is a relationship between the immunoreaction for β-APP and the time elapsed since trauma to death. There is not any significant reaction to this marker in cases where survival time is shorter than 30 minutes, whatever the cause of death may be. In addition, the penumbra period in which β-APP is not detectable, gets shorter as the severity of trauma increases. (29)
In general, it is accepted that, in patients elder than 1 year, DAI cannot be diagnosed before than 1,5 hours post-injury. (17)
In earlier forensic studies, axonal bulbs are not observed in cases where survival time is shorter than 6 hours. This is the point in time in which β-APP and neurofilaments accumulate in the soma of neuron. (36). Far from the area directly affected by trauma, swelling axons and varicosities (stained with β-APP and neurofilaments markers) are present since the 3d to 7th days after injury. They begin to disappear after 6 months of the trauma. Nevertheless β-APP is positive 3 hours after injury in the contused area and it is still positive 6 months later in parenchyma and around vessels (37). Other authors proved the presence of β-APP staining 24 hours after trauma in swelling neurons that undergo dystrophy of their fibers. (15)
In cases of mild trauma, the β-APP shows a specific chronological behavior: its first immunoreactivity takes place between 1.5 and 3 hours after trauma. It reaches a maximal peak of expression at 10-15 hours, and it stays stable during days or weeks. (14)
Chronological differences between our results and those of other authors may be due to that they observe the expression of this marker in brain regions far from that directly affected. It means that they study the damage secondary to hypoxia and this phenomenon is time dependent according to the severity of trauma. Moreover, the sensibility to hypoxia in each part of the brain is different, so that they also show chronological differences expressing β-APP
Kelley (18) studied how axonal transport gets disturbed through the assessment of the β-APP expression in the thalamic nucleus after a controlled axotomy. This encephalic structure is very sensitive to hypoxia and in consequence to secondary axonal damage: fifteen minutes after axotomy the thalamic nucleus shows immunoreactivity to this protein. The diameter of the axons appears a little enlarged and since 60 minutes after trauma axonal bulbs begin to appear.
The first regions where β-APP is seen are the parasagittal tract and structures of the mid line of the brain including the caudal zone of the corpus callosum (38). Thus, the most common distribution of β-APP immunoreactivity involves the brain stem (95% of cases), the internal capsule and the thalamus (80% of cases), the corpus callosum and the parasagittal white matter (70% of cases). As we can see more expression of β-APP other than hemorrhages, it can be said that its accumulation does not depend on the presence of hemorrhages. (14). Our results confirm all this, since this marker was positive in all regions which showed external signs of contusion, regardless the severity of trauma.
In the pons, it is not possible to see any reaction for β-APP before three hours after trauma, regardless of the presence of subdural hematoma or cortical hemorrhage, which would demonstrate the vitality of the lesion. β-APP accumulates even in cases of non-mechanical trauma, but in cases of hypoxia or ischemia the expression of β-APP takes place later (8 to 24 hours after the insult) and the staining is weaker than in cases of mechanical trauma.
Diffuse axonal injury can be observed in the pons using β-APP regardless of the causes, because many times, its presence in this encephalic structure only represents the delayed consequences of complex changes in cytoskeleton after trauma or ischemia, which leads to the collapse of anterograde axoplasmatic transport. This situation evolves to axonal swelling, varicosities and axonal bulbs and ends with axonal disruption, as it has been described before. Nevertheless, the topographic pattern of distribution of the expression of axonal injury along the structures of midline of the brain may be useful to diagnose the differences between traumatic and non-traumatic causes of axonal injury (24)
On the other hand, the severity of the histopathological expression of diffuse axonal injury is assessed by the diameter of the swollen axons, which depends on several factors: the time elapsed since trauma, the diameter of the axons before swelling, length of time the secondary effect of trauma acts upon the axons and the time that any regeneration mechanism may take (25).
Finally, the diffuse axonal injury can begin even 84 hours after the insult, which demonstrate the presence of a continuous secondary axonal damage. In addition, the immunoreactivity is still positive months after the insult, which confirms the persistence of the fast axonal flow. Anyway, this situation can give rise to degenerative diseases like Alzheimer or Parkinson. Fortunately, during the first moments after trauma there is a significant period of regeneration, this is why axonal injury can be healed, to a certain extent, in cases of mild trauma. (39)
Another factor that makes some difference in the expression of β-APP is the diameter of axons. Its behavior in the optic chiasma and corpus callosum has been studied and the peak of expression in the former take place at 8 hours after trauma and at 28 hours in the latter, due to the thinner diameter of its axons and the higher proportion of microtubules of its cytoskeleton. The axons of the chiasma are thicker and have more density of neurofilaments. (40).
Summary, the active process of β-APP formation and accumulation is clearly obvious in the first 24 hours after trauma, reaches a plateau at 10-14 days and then, began to disappear as gliosis grows around. Using immunohistochemistry techniques, the first changes in β-APP transient (swelling axons) were seen 30 minutes after the injury and they are visible during weeks. Thus, we can consider that it is positive in the first hour post-trauma, not only in the contused area, but in remote regions of the brain. The maximal peak of expression occurs 48 hours after injury and can be seen until two weeks after in white matter of both hemispheres, hippocampus, and cortex. In the brain stem it remains positive only for one week. (41,42)
Once again, working under real work conditions restrict the interpretation of our results because it is no possible to know the intensity of the impact, and it is not practical to sample the whole brain. In the same way, this marker has some issues of sensibility (24,43,44).
Nevertheless, our results are not very far from consensus, specially about the penumbra period in the first two hours after trauma. This period is an important medico-legal issue, above all in cases of mild head trauma. We were able to see weakly stained somas between 30 minutes and 2 hours post-injury. On the other hand, in cases of deferred death after head trauma (unique or repetitive), the pattern (''Z'' or diffuse) allows us to settle the relationship between the traumatic event and the cause of death, even though after 24 hours or more after the injury.
Conclusion
β-APP is a very well-studied marker with clinical and prognostic purposes to settle long term sequelae after TBI. We highlight that it is also very useful to solve forensic issues. First, because it is affordable and available; second, because its only presence means axonal injury, since β-APP is negative in physiological conditions.
Our study reproduces the medical examiner daily work conditions, so it simplifies sampling and the interpretation of results. Thus, the different kind of changes highlighted with this marker (staining of neuronal somas, beaded axons, retraction bulbs) allow to reduce the penumbra period; and the pattern of expression of β-APP (diffuse or ''Z'') distinguish between primary traumatic injury or secondary ischemic damage.
All these phenomena don not depend on the kind or intensity of head trauma, although they are weaker in those cases without intracranial hemorrhage. Therefore, this marker is useful to link a deferred death with mild head trauma, which helps to solve issues of forensic significance.
Source: MeSH (Medical Subject Headings)
References
1. Morganti-Kossmann MC, Satgunaseelan L, Bye N, Kossmann T. Modulation of immune response by head injury. Injury Int J Care Injured. 2007;38:1392-1400 [ Links ]
2. Faul M, Conrado V. Epidemiology of traumatic brain injury. Handb Clin Neurol (2015) 127:3-13. [ Links ]
3. Graham DI, Smith C, Reichard R, Leclercq PD, Gentelman SM. Trials and tribulations of using Beta-amyloid precursor protein inmunohistochemistry to evaluate traumatic brain injury in adults. Forensic Sci Int. 2004;146:89-96 [ Links ]
4. Tagliaferri F, Compagnone C, Korsic M, Servadei F, Kraus J. A systematic review of brain injury epidemiology in Europe. Acta Neurochir (Wien) (2006) 148:255-68 [ Links ]
5. Maas AI, Stochetti N, Bullock R. Moderate and severe traumatic brain injury in adults. Lancet Neurol (2008) 7:728-41 [ Links ]
6. Roozenbeek B, Maas AIR, Menon DK. Changing patterns in the epidemiology of traumatic brain injury. Nat Rev Neurol (2013) 9:231-6 [ Links ]
7. Li DR, Zhang F, Wang Y, Tan XH, Quiao DF, Wang HJ, Michue t, Maeda H. Quantitative analysis of GFAP and S100 protein-immunopositive astrocytes to investigate the severity of traumatic brain injury. Legal Medicine. 2012;14:84-92 [ Links ]
8. Papa L, Silvestri S, Brophy GM, Giordano P, Falk JL, Braga CF, Tan CN, Ameli NJ, Demery JA, Dixit NK, Mendes ME, Hayes RL, Wang KKW, Robertson CS. GFAP Out-performs S100β in detecting traumatic intracranial lesions on computed tomography in trauma patients with mild traumatic brain injury and those with extracranial lesions. J. Neurotrauma. 2014;31:1815-1822 [ Links ]
9. Bieniek KF, Ross OA, Cormier KA, Walton RL, Soto-Ortolaza A, Johnston AE, DeSaro P, Boylan KB, Graff-Radford NR, Wszolek ZK, Rademakers R, Boeve BF, McKee AC, Dickson DW. Chronic traumatic encephalopathy pathology in a neurodegenerative disorders brain bank. Acta Neuropathol. 2015;130(6): 877-899 [ Links ]
10. Hamdeh SA, Waara ER, Möller C, Söderberg L, Basun H, Alafuzoff I, Hillered L, Lannfelt L, Ingelsson M, Marklund N. Rapid amyloid β oligomer and potofibril accumulation in traumatic brain injury. Brain Pathology 2018;28:451-462 [ Links ]
11. Stein TD, Montenigro PH, Alvarez VE, Xia W, Crary JF, Tripodis Y, Daneshvar DH, Mez J, Solomon T, Meng G, Kubilus CA, Cormier KA, Meng S, Babcock K, Kiernan P, Murphy L, Nowinski CJ, Martin B, Dixon D, Stern RA, Cantu RC, Kowall NW, McKee AC. Beta-amyloid deposition in chronic traumatic encephalopathy. Acta Neuropathol. 2015;130(1): 21-34 [ Links ]
12. Winston CN, Wilkins T, Barton DJ, Washington PM, Loane DJ, Zapple DN, Burns MP. Controlled cortical impact results in an extensive loss of dendritic spines that is not mediated by injury-induced amyloid-beta accumulation. J. Neurotrauma. 2013;30:1966-1972 [ Links ]
13. Siman R, Toraskar N, Dang A, McNeil E, McGarveyM, Plaum J, Maloney E, Grady MS.. A panel of neuron-enriched proteins as markers for traumatic brain injury in humans. J. Neurotrauma. 2009;26:1867-1877 [ Links ]
14. McKenzie KJ, McLellan DR, Gentleman SM, Maxwell WL, Gennarlli TA, Graham DI. Is beta-APP a marker of axonal damage in short-surviving head injury?. Acta Neuropathol 1996;92:608-613 [ Links ]
15. Itoh T, Satou T, Nishida S, Tsubaki M, Hashimoto S, Itoh H. Expression of amyloid precursor protein after rat traumatic brain injury. Neurol Res 2009;31:103-109 [ Links ]
16. Hayashi T, Ago K, Nakamae T, Higo E, Ogata M. Two different immunostaining patterns of beta-amyloid precursor protein (APP) may distinguish traumatic from nontraumatic axonal injury. Int J Legal Med 2015;129:1085-1090 [ Links ]
17. Oehmichen M, Meissner C, Schmidt V, Pedal I, König HG, Saternus KS. Axonal injury: a diagnostic tool in forensic neuropathology?. Forensic Sci Int 1998;95:67-83 [ Links ]
18. Kelley BJ, Farkas O, Liftshitz J, Povlishock JT (2006). Traumatic axonal injury in the perisomatic domain triggers ultrarapid secondary axotomy and wallerian degeneration. Exp Neurol 2006;198:350-360 [ Links ]
19. Staal JA., Dickinson TC., Gasperini R., Liu Y., Foa L., Vickers JC. Initial calcium release from intracellular stores followed by calcium dysregulation is linked to secondary axotomy following transient axonal stretch injury. J. Neurochem 2010;112:1147-1155 [ Links ]
20. Chen XH, Siman R, Iwata A, Meaney DF, Trojanowski JQ, Smith DH. Long-term accumulation of amyloid beta, beta secretase, presenilin-1, and caspase-3 in damaged axons following brain trauma. AJP 2004;165(2):357-371 [ Links ]
21. Kokiko-Cochran ON and Godbout JP. The inflammatory continuum of traumatic brain injury and Alzheimer's disease. Front Immunol 2018;9:672 [ Links ]
22. Shishido H, Ueno M, Sato K, Matsumura M, Toyota Y, Kirino Y, Tamiya T, Kawai N, Kishimoto Y. Traumatic brain injury by weight-drop method causes transiente amyloid-β deposition and acute cognitive deficits in mice. Behavioural Neurology 2019;Article ID 3248519, 8 pages [ Links ]
23. Onyszchuk G, LeVine SM, Brooks WM, Berman NEJ. Post-acute pathological changes in the thalamus and internal capsule in aged mice following controlled cortical impact injury; a magnetic resonance imaging, iron histochemica, and glial immunohistochemical study. Neurosci Lett. 2009;452:204-208 [ Links ]
24. Oehmichen M, Theuerkauf I, Meissner C. Is traumatic axonal injury (AI) associated with an early microglial activation? Application rl a double-labeling technique for simultaneous detection of microglia and AI. Acta Neuropathol. 1999;97:491-494 [ Links ]
25. Willemse-Van Son AHP, Ribbers GM, Hop WCJ, Van Duijin CM, Stam HJ. Association between apolipoprotein-ε4 and long-term outcome after traumatic brain injury. J Neurol Neurosurg Psychiatry. 2007;79:426-430 [ Links ]
26. Omalu BI. Diagnosis of traumatic diffuse axonal injury Am J Forensic Med Pathol. 2004;25(3):270-271 [ Links ]
27. Ubukata S, Oishi N, Higashi T, Kagawa S, Yamauchi H, Okuyama C, Watananbe H, Ono M, Saji H, Aso T, Murai T, Ueda K. Spatil patterns of amyloid deposition in patients with chronic focal or diffuse traumatic brain injury using F-FPYBF-2 PET. Neuropsychiatric Disease and Treatment. 2020; 16:2719-2732 [ Links ]
28. Grovola MR, Paleologos N, Brown DP, Tran N, Wofford KL, Harris JP, Browne KD, Shewokis PA, Wolf JA, Cullen DK, Duda JE. Diverse changes in microglia morphology and axonal pathology during the course of 1 year after mild traumatic brain injury in pigs. Brain Pathology. 2021;31:12953-12972 [ Links ]
29. Orthobagyi T, Wise S, Hunt N, Cary N, Djurovic V, Fegan-Earl A, Shorrock K, Rouse D, Al-Sarraj S. Traumatic axonal damage in the brain can be detected using beta-APP immunohistochemistry within 35 minutes after head injury to human adults.Neuropathol Appl Neurobiol 2007;33:226-23 [ Links ]
30. Reichard R, Smith C, Graham DI (2005). The significance of beta amyloid precursor protein inmunoreactivity in forensic practice. Neuropathol Appl Neurobiol 2005;31:304-313 [ Links ]
31. Rahaman P and Del Bigio M. Histology of brain trauma and hypoxia-ischemia. Acad Forensic Pathol 2018;8(3): 539-554. doi: 10.1177/1925362118797728 [ Links ]
32. Morrison C, McKenzie JM. Axonal injury in head injuries with very short survival times. Neuropathol Appl Neurobiol. 2008;34:124-125 [ Links ]
33. Ogata M. Early diagnosis of diffuse brain damage resulting from a blunt head injury. Leg Med 2007;9(2):105-8 [ Links ]
34. Smith C, Gentelman SM, Leclercq PD, Muray LS, Griffin WS, Graham DI, Nicoll JA. The neuroinflammatory response in humans after traumatic brain injury. Neuropathol. Appl. Neurobiol. 2013;39:654-666. [ Links ]
35. Romero MA, Blanco JM and Gallego R. Dating of traumatic brain injury in forensic cases using immunohistochemical markers(I): Neurofilaments and β-Amyloid precursor Protein. Am J Forensic Med Pathol 2018;39: 201-207 [ Links ]
36. Bisht A, Garg K, Agrwal D, Singh PK, Satyarthee GD, Gupta D, Sinha S, Kakkar A, Suri V, Lalwani S, Kale SS, Sharma BS. Histological changes in thalamus in short term survivors following traumatic injury: an autopsy study. Neurol. India 2013;61(6):599-605. [ Links ]
37. Uryu K, Chen XH, Martínez D, Browne KD, Johnson VE, Graham DI, Lee VM, Trojanowski JQ, Smith DH. Multiple proteins implicated in neurodegenerative diseases accumulate in axons after brain trauma in humans. Exp Neurol. 2007;208(2):185-92. [ Links ]
38. Li S, Sun Y, Shan D, Feng B, Xing J, Duan Y, Dai J, Lei H, Zhou Y. Temporal profiles of axonal injury following impact acceleration traumatic brain injury in rats. A comparative study with diffusion tensor imaging and morphological analysis. Int. J. Legal Med. 2013;127:159-167. [ Links ]
39. Wilkinson AE, Brideges LR, Sivaloganathan S. Correlation of survival tiem with size of axonal swellings in diffuse axonal injury. Acta Neuropathol. 1999;98:197-202 [ Links ]
40. Zakaria N., Kallakuri S, Bandaru S, Cavanough JM Temporal assessment of traumatic axonal injury in the rat corpus callosum and optic chiasm. Brain Res. 2012;1467:81-90 [ Links ]
41. Pierce Jean ES, Trojanowski JQ, Graham DI, Smith DH, McIntosh TK. Inmunohistochemical characterization of alterations in the distribution of amyloid precursor proteins and beta amyloid peptide after experimental brain injury in the rat. J Neuroscien. 1996;16(3):1083-1090 [ Links ]
42. Ekmark-Lewén S, Flygt J, Kiwanuka O, Meyerson BJ, Lewén A, Hillered L, Marklund N. Traumatic axonal injury in the mouse is accompained by a dynamic inflammatory response, astroglial reactivity and complex behavioural changes. J. Neuronfl. 2013;10:44-63 [ Links ]
43. Raghupathi R. Cell death mechanisms following traumatic brain injury. Brain Pathol 2004;14:215-222 [ Links ]
44. Dolinak D, Reichard R. An overview of inflicted head injury in infants and young children, with a review of beta amyloid precursor protein immunohistochemistry. Arch Pathol Lab Med 2006;130:721-717 [ Links ]
Received: April 24, 2022; Accepted: July 14, 2022