Introduction
Originally from South America, the free-floating aquatic plant Eichhornia crassipes (known as water hyacinth, camalote, aguapé) has invaded warm waters in over 50 countries on five continents (Villamagna & Murphy 2010). Floating meadow is a typical feature of the floodplain of large South American rivers such as Paraguay, Orinoco, and Pilcomayo. E. crassipes is the most frequent species in the floodplains of the Paraná River occupying the lakes that receive the frequent overflow of the river (Neiff, Casco, Mari, Di Rienzo, & Poi 2014), where may cover 30-100 % of the available surface of the lakes. In the low water phase, plants grow limited by nitrogen (Carignan & Neiff 1992) and during prolonged floods increase leaf length, leaf biomass and nutrient content in green leaves and decrease root biomass (Neiff, Casco, & Poi 2008).
The submerged roots are colonized by more than 79 taxa of macroinvertebrates in the Paraná River floodplain (Poi de Neiff & Neiff 2006). Above the water line, leaves have less species richness, mostly herbivorous (Franceschini, Poi de Neiff, & Galassi 2010). The abundance of macroinvertebrates on E. crassipes roots varied between 5 395 and 24 116 ind.m2 depending on the four variables: hydrological period, site, conductivity, and dissolved oxygen (Poi de Neiff & Carignan 1997). These values can be more than six times greater when samples include macroinvertebrates captured with mesh between 500 and 100 µm (Blanco Belmonte, Neiff, & Poi de Neiff 1998).
The diet of the dominant fish of the Paraná River floodplain had a high frequency of organisms associated with the floating macrophytes (Neiff, Poi de Neiff, & Canón Verón 2008). Plants provide food and habitat for fish, especially Prochilodus lineatus and Gymnotus omarorum, which are relevant for subsistence fishing by local indigenous communities in the Pilcomayo floodplain. According to Ferriz and Iwaszkiw (2014), G. omarorum feeds preferentially on larvae of odonates, shrimp and other macroinvertebrates living in the roots of E. crassipes.
Habitat complexity defined by the type of plant community that occurs in a wetland is one of the fundamental drivers that determine the distribution of macroinvertebrate assemblages because the different architectures and growth forms of aquatic plants affect their abundance, species richness, biomass and trophic structure (Batzer & Wissinger 1996, Thomaz, Dibble, Evangelista, Higuti & Bini 2008).
In order to investigate how much of the variation in macroinvertebrate assemblages is explained by the environmental factors, we aimed to describe the main traits of E. crassipes floating meadows, and analyze the abundance and composition of macroinvertebrate assemblages associated with their roots in two protected floodplain wetlands of the Paraná River (within the Ramsar site Wetlands Chaco) and of the Pilcomayo River (within the Pilcomayo River National Park). We hypothesized that 1) the traits of E. crassipes floating meadows and 2) the abundance and composition of macroinvertebrates associated with their roots vary in sites with different water and climate conditions. We predicted that E. crassipes floating meadows should respond to environmental changes (Carignan, Neiff & Planas 1994) and that longest root should appear when E. crassipes growing limited by nitrogen (Carignan & Neiff 1992). Under these circumstances, the macroinvertebrates associated with the root system should be dominated by tolerant species to oxygen depletion (Poi de Neiff & Carignan 1997).
Materials and methods
Study sites: We selected two floodplain lakes, 263 km distant, in the northeastern region of Argentina, where E. crassipes was found in monospecific stands with similar coverage. Laguna Blanca Lake (25˚09’48’’ S and 58˚08’51’’ W) is the unique floodplain lake located on the paleochannel-bed of the lower Pilcomayo River (Formosa) and El Puente Lake (27˚26’23” S and 58˚51’19” W) is located on the alluvial plain of the Paraná River (Chaco) downstream of the Paraná-Paraguay River confluence. Laguna Blanca (Fig. 1) is a shallow lake (1.60-1.90 m) with an approximate surface of 800 ha. The floating meadow with the absolute dominance of E. crassipes covers 10-20 % of the surface, distributed in a strip of three to 15 meters wide. This protected lake (within the Pilcomayo National Park), has warm water with high dissolved oxygen and nutrients availability. The water is turbid due to the abundance of silt and clay and has low to moderate salinity. The ionic typology is: HCO3 -> Na+> K+> Cl-> SO4 -> Mg+> Ca+.
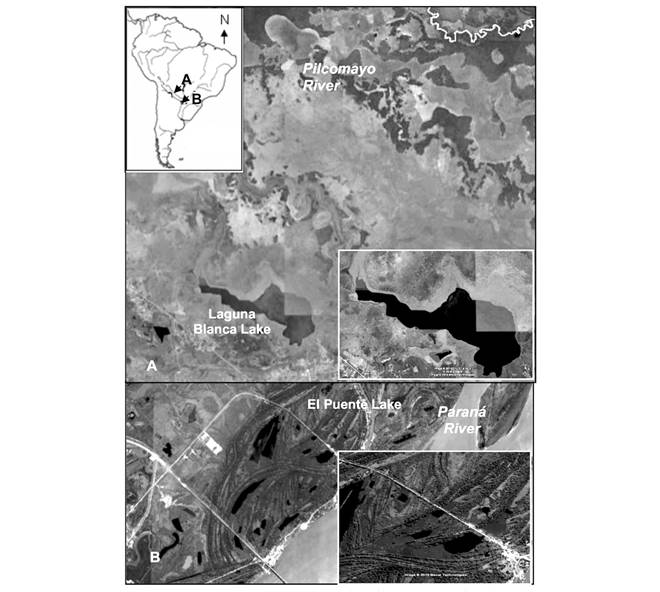
Fig. 1 Study sites A. Laguna Blanca Lake (within the Pilcomayo National Park and B. El Puente Lake in the Paraná River floodplain (within the Ramsar site Wetlands Chaco).
El Puente Lake belongs to a complex of several similar oxbow lakes (Fig. 1) located parallel to, and approximately 2 km from the Paraná River, and was selected based on the expertise accredited by previous research in the study area. It is small (200 × 3 000 m), shallow (0.90 - 2.5 m deep) and separated from the river by alluvial levees (10 - 20 m wide, 1 - 2 m high) occupied by gallery forests. Water hyacinth covered 40 - 60 % of its surface. The lake is fed periodically by the overflows of the Paraná River, but the frequency, duration, and timing of the connections vary with river stage. During the study period, the Paraná was at low water and the lake disconnected from the river.
The waters have moderate salinity and low nutrient content (Carignan & Neiff 1992) and belong to the ionic typology: HCO3 -> Na+> Cl-> Mg+> SO4 -> Ca+> K+.
Sampling methods: Macroinvertebrates were collected using a 35 cm diameter net, with 500 µm mesh size and 962 cm2 area (Poi de Neiff & Carignan 1997, USEPA 2002). The net with a 1.5 m long handle, operated from a boat, was introduced vertically under the roots (70 cm depth approximately) by lifting it into a horizontal position. During October and December 2012, 13 samples were obtained in El Puente Lake and 11 in Laguna Blanca Lake. Each sample constituted a discrete representation of plant and macroinvertebrate material collected from independent stands separated by 5 m from each other. At each point, two pseudo-replicates were extracted.
In the laboratory, the aquatic plants were thoroughly washed to detach the macroinvertebrates, and the obtained suspensions were filtered through sieves of 1 mm and 500 µm and preserved in 70 % ethanol. Macroinvertebrates were counted and identified to the lowest practical taxonomic level (usually family) using keys from Michat, Archangelsky & Bachmann (2008), Domínguez & Fernández (2009), Ramírez (2010) and Libonatti, Michat & Torres (2011). The macroinvertebrate abundance was expressed as individuals per 1 000 g roots dry weight and number of individuals per square meter.
At both floodplains, plants of water hyacinth with elongate leaves (large biotype), which occurs in dense mats, were dominant. The short inflated petiole with wider than long laminas (small biotype), which occurs in more open conditions, was exceptionally found during the study period. The mean number of leaves was determined on a circular plot of 0.30 m2 in each point where the macroinvertebrates were collected. All plants in the plot were cut and collected in plastic bags. In the laboratory, the leaves were separated and counted. The length of the green leaves was measured from the base of the petiole to the end of the leaf. The root length was measured from the rhizome to the end of the root cluster. The green leaves and roots were washed and dried at 105 ºC for 72 hours to obtain constant dry weight.
Measurements of the limnological characteristics were taken inside the floating meadows and simultaneously with the collection of the macroinvertebrate samples. Water temperature, electrical conductivity, and oxygen concentration were measured with an YSI 54A polarographic probe, conductivity was recorded with an YSI 33 SCT device, and pH was measured with a WTW 330/SET-1 digital pH meter.
For nutrient analyses, water samples were filtered within 1 - 2 h of collection on pre-washed Gelman DM-450 (0.45 mm - pore) membranes for spectrophotometric analyses of NH4 + (indophenol blue method), NO3 - + NO2 - (called NO3 -) by Cd reduction and orthophosphate called Phosphate (molybdenum blue method) with persulfate oxidation (APHA l995). The chlorophyll-a concentration was measured by the fluorometric method in 500 ml water samples taken adjacent to floating meadows at a depth of 20 cm. After 24 hours of acetone maceration, the algal suspensions were filtered onto Whatman GF/C filters of 0.7 a 1.2 µm.
Data Analyses: The non-parametric Kruskal-Wallis test was used to detect significant differences between both floodplains taking into account the limnological characteristics, the traits of E. crassipes floating meadows and the abundance of macroinvertebrates.
The abundance (ind.m2) of 14 macroinvertebrate taxa (those were more frequent and abundant) and the environmental variables that had significant differences between both floodplains (temperature, dissolved oxygen, electrical conductivity, pH, PO4 3-, NO3 -, roots length and roots dry weight) were ordered using a Non-Metric Multidimensional Scaling (NMDS). To evaluate statistical differences in the abundance of the macroinvertebrate taxa between both floodplains, a Analysis of Similarities (ANOSIM), were performed (Clarke 1993), using the Bray-Curtis distance and 9 999 random permutations to estimate the significance of the R statistic associated with ANOSIM. Prior to this analysis, abundance data have been standardized through logarithmic transformation (log10 x+1). The statistical analyses were performed using InfoStat (Di Rienzo, Casanoves, Balzarini, González, Tablada & Robledo 2013) and PAST 2.08 (Hammer, Harper & Ryan 2001) software.
Results
Water condition: Temperature (H = 16.5, p < 0.0001), dissolved oxygen (H = 16.5, p < 0.0001), phosphate content (H = 16.5, p < 0.0001) and nitrate concentration (H = 14.73, p < 0.0001) were significantly higher in the Pilcomayo than in the Paraná floodplain (Table 1). Although the differences in pH between both floodplains were significant with the test used (H = 9.60, p = 0.0019), the values found were close to neutrality. Transparency did not show significant differences (H = 1.74, p = 0.1862) between both floodplains. Electrical conductivity (Table 1) was significantly higher in the Paraná than in the Pilcomayo floodplain (H= 15, p = 0.0001). The mean chlorophyll a (Table 1) was lower than 1.7 µg.l-1.
TABLE 1 Traits of E. crassipes stands, water condition and overall macroinvertebrate abundance in the floating meadows of the Paraná and Pilcomayo floodplain between October and December 2012
Paraná | Pilcomayo | |
Traits of E. crassipes | ||
Leaf density per m2 | 290 ± 39.6 | 270 ± 28.5 |
Leaf biomass per m2 | 817 ± 110 | 1 100 ± 335 |
Mean leaf height (cm) | 68 ± 12 | 89 ± 26 |
Mean root length (cm) | 74 ± 10 | 42 ± 18 |
Mean root biomass g.m2 | 450 ± 69 | 379 ± 41 |
Water condition | ||
Temperature (°C) | 23.5 ± 0.7 | 30.4 ± 2.4 |
Transparency (cm) | 47.6 ± 18.0 | 27 ± 11.4 |
Conductivity (µS.cm-1) | 262 ± 36.2 | 169.3 ± 28 |
Dissolved oxygen (mg.l-1) | 2.8 ± 1.1 | 6.6 ± 0.96 |
pH | 6.5 ± 0.3 | 7.2 ± 0.8 |
NO3-N (µg.l-1) | Bdl | 76.6 ± 53.1 |
NH4-N (µg.l-1) | 70 ± 19.7 | 78.3 ± 14.2 |
PO4-P (µg.l-1) | 24.2 ± 24.7 | 343.3 ± 105.2 |
Chlorophylla (µg.l-1) | 1.7±0.47 | Bdl |
Macroinvertebrate abundance | ||
Number of Individuals per 1 000 g roots dry weight | 17 009 ± 5 981 | 24 580 ± 3 752 |
Number of Individuals per m2 | 6 244 ± 1 654 | 4 198 ± 1 213 |
Bdl= below the detection limit.
Plant traits and biomass: Leaf density was significantly higher in the Paraná than in the Pilcomayo floodplain (H = 4.92, p = 0.0265). However, the green leaf biomass (H = 15.25, p = 0.0001) and height (H = 18.72, p < 0.0001) were higher in the Pilcomayo (Table 1). Finally, the root biomass (H= 17.16, p < 0.0001) and the root length (H = 19.5, p < 0.0001) were higher in the Paraná, where they reached a maximum length of 84 cm (Table 1).
Abundance and richness of macroinvertebrate assemblages: The number of macroinvertebrates per 1 000 g roots dry weight (H = 7.9, p = 0.005) and per square meter (H = 8.56, p = 0.0034) differed significantly between the floodplains (Table 1). Note that the number of macroinvertebrates per 1 000 g roots dry weight was higher in the Pilcomayo floodplain than in the Paraná floodplain due to the lower root weight for the same surface unit. A total of 44 families of macroinvertebrates (34 in the Paraná and 33 in the Pilcomayo floodplain) from 11 major groups were recorded (Table 2), with 25 families in common.
TABLE 2 Comparison of the mean relative abundance of macroinvertebrates associated with E. crassipes between Laguna Blanca (Pilcomayo River floodplain) and El Puente oxbow (Paraná River floodplain). References: + presence, - absence.
Pilcomayo | Paraná | |
OLIGOCHAETA | ||
Naididae | 4.7 | 19.4 |
Lumbricumorpha | - | + |
OSTRACODA | 3.90 | 16.5 |
Limnocytheridae, Cytheridella ilosvayi (Daday) | + | + |
HIRUDINEA | ||
Glossiphoniidae | 0.14 | 4.74 |
CONCHOSTRACA | ||
Cyclestheriidae, Cyclestheria hislopii (Baird) | 4.30 | 0.09 |
CLADOCERA | 3.28 | 5.70 |
Daphnidae, Simocephalus vetulus (Müller) | + | - |
Macrothricidae, Grimaldina brazzai (Richard) | - | + |
Chydoridae, Euryalona occidentalis (Sars) | - | + |
COPEPODA | 0.42 | 1.96 |
Microcyclops anceps (Ricard) | + | + |
Macrocyclops albidus (Jurine) | + | + |
AMPHIPODA | ||
Hyalellidae, Hyalella curvispina (Shoemaker) | 9.06 | 1.93 |
DECAPODA | ||
Trichodactylidae, Trichodactylus borellianus (Nobili) | 0.14 | - |
Palaemonidae | 1.93 | - |
Macrobrachium jelskii (Miers) | + | - |
Macrobrachium amazonicum (Heller) | + | - |
INSECTA | ||
HEMIPTERA Adults | ||
Belostomatidae, Belostoma micantulum (Stal) | 0.26 | 0.03 |
Pleidae, Neoplea maculosa (Berg) | 0.16 | 0.33 |
Naucoridae, Pelocoris sp. | - | 0.19 |
ODONATA larvae | ||
Coenagrionidae | 1.74 | 1.36 |
Libellulidae | 1.43 | 0.77 |
Aeshnidae | - | 0.03 |
EPHEMEROPTERA larvae | ||
Caenidae, Caenis sp. | 0.37 | - |
Baetidae, Callibaetis sp. | 0.05 | - |
DIPTERA larvae and pupae | ||
Culicidae, Mansonia sp. | 2.36 | 1.38 |
Chironomidae | 21.09 | 12.29 |
Tipulidae | 0.21 | - |
Tabanidae | 0.06 | 1.2 |
Ephydridae | 0.44 | 0.36 |
Stratiomyidae | - | 1.05 |
Syrphidae, Eristalis sp. | - | 0.49 |
Ceratopogonidae, | 0.34 | 1.61 |
Forcipomyia sp. and Bezzia sp. | + | + |
COLEOPTERA adults and larvae | ||
Noteridae, Hydrocanthus sp. | 1.09 | 1.89 |
Scirtidae, Scirtes sp. | 0.07 | 1.15 |
Dytiscidae | 2.84 | 10.95 |
Laccophillus sp. and Desmopachria sp. | + | + |
Curculionidae, Neochetina bruchi(Hustache) and N. eichhorniae (Warner) | 0.84 | 1.12 |
Hydrophilidae | 3.27 | 4.60 |
Hydrochus richteri (Bruch) | + | + |
Derallus sp. | + | + |
Enochrus sp. | + | + |
Helochares sp. | + | + |
Berosus sp. | + | + |
Pselaphidae | - | 0.24 |
Staphylinidae | - | 0.54 |
Lampyridae | 0.2 | - |
LEPIDOPTERA larvae and pupae | 0.8 | 0.39 |
MOLLUSCA | ||
Planorbidae, Drepanotrema sp. | 1.2 | 2.21 |
Ampullariidae, Pomacea canaliculata (Lamark) | - | 0.24 |
Hydrobiidae, Heleobia parchappii (d’Orbigny) | 30.5 | 1.43 |
Ancylidae | 2.02 | - |
Piscidae | 0.26 | - |
HIDRACNIDIA | 0.53 | 3.83 |
Total number of families | 33 | 34 |
The most abundant taxonomic groups on water hyacinth roots (Fig. 2) in the Paraná River floodplain were oligochaetes (Naididae, 19.7 %), ostracods (Limnocytheridae mainly Cytheridella ilosvayi, 16.67 %) and larvae of non biting midges (Chironomidae, 12.59 %). Two families Hydrobiidae (Heleobia parchappii) and Chironomidae (larvae and pupae) account for 49.3 % of the total abundance in the Pilcomayo floodplain.
The differences between both floodplains were significantly for Naididae (H = 13.11, p = 0.0003), Limnocytheridae (H = 17.16, p = 0.0001) and Hydrobiidae (H = 16.5, p = 0.0001).
Amphipods (Hyalella curvispina) were significantly more abundant (H = 6.50, p = 0.01) in the Pilcomayo floodplain and, Glossiphoniidae (H = 17.16, p = 0.0001) and Dytiscidae (H = 14.18, p = 0.0002) in the Paraná floodplain. Palaemonidae (Macrobrachium jelskii and M. amazonicum) had a mean abundance of 77 ± 46 ind.m2 in the Pilcomayo floodplain, and they were not found in the Paraná floodplain (Fig. 2).
Euryalona occidentalis (Chydoridae) and Grimaldina brazzai (Macrothricidae) were the dominant cladocerans associated with E. crassipes in the Paraná River floodplain. Simocephalus vetulus (Daphnidae) was a more frequent cladoceran in the Pilcomayo floodplain, but the counts were made at the Order level (Table 2). The two species of weevils (Neochetina bruchi and N. eichhorniae) feed on the leaves but during the day were found near the stems. Its abundance reached 176 ind.m2 in the Pilcomayo floodplain. The other taxa had low relative abundance (Table 2).
The NMDS results reflected the differences in the abundances of macroinvertebrate taxa (mostly at family-level) between both floodplains (ANOSIM: R = 0.93, p = 0.0001) and the ordination had low final stress (9.2 %). The NMDS ordination separated the abundances of macroinvertebrate taxa found in the Pilcomayo and Paraná floodplains, which are grouped on the right and left of the first axis, respectively (Fig. 3). When environmental variables were analyzed, the NMDS ordination showed that some of these variables were highly correlated with the gradients in macroinvertebrate taxa abundance (Fig. 3). Nitrate (0.96), dissolved oxygen (0.93), electrical conductivity (-0.92), roots dry weight (-0.88), temperature (0.88), roots length (-0.85) and phosphate (0.86) had the highest correlation values, whereas pH had the lowest one (0.62).
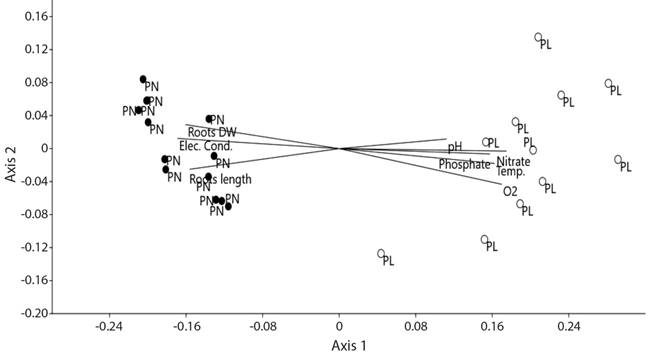
Fig. 3 NMDS ordination of environmental variables and the macroinvertebrate abundance (Ind.m2) in both floodplains. The proximity of symbols indicates a higher degree of similarities. Black and empty circles represent the samples collected in the Paraná and Pilcomayo floodplains, respectively. PN= Paraná, PL= Pilcomayo, Elec. Cond.= electrical conductivity, Roots DW= roots dry weight, Temp.= temperature.
The proportion of the variance represented by the first and second NMDS axis was 93 % and 0.82 %, respectively.
Discussion
Our results indicated that E. crassipes growing in the Pilcomayo floodplain had longer leaves and less root biomass than those found in the Paraná floodplain, which is limited by nutrients. Previous studies carried out in floodplain lakes demonstrated that leaf height, leaf biomass, and root biomass respond to the influx of nutrients during the prolonged hydrologic connection with the Paraná River (Neiff, Casco, & Poi, 2008). The density of the root in the Paraná floodplain influence oxygen concentration in the root zone by trapping organic matter and by preventing the re-aeration on the surface of the water. The low dissolved oxygen content within the floating meadows was attributable to intense heterotrophic activity in the E. crassipes roots (Carignan & Neiff 1992). The position of Laguna Blanca (Pilcomayo floodplain) respect to the wind action and the short root system enable the mixture of the water and the oxygen dissolution.
The mean number of macroinvertebrates per square meter found in both floodplain was in the range observed previously in studies conducted with the same methods in the Paraná floodplain (Poi de Neiff & Carignan 1997). Most of the registered taxa were common to both plains, but the abundance of some of them differed significantly.
The results of NMDS indicate that water conditions (temperature, dissolved oxygen, electrical conductivity, phosphate and nitrate content) of the studied floodplains, and roots biomass and length (which affect dissolved oxygen content in the floating meadows), are determinant of the composition of the macroinvertebrate assemblages. Phosphate and nitrate contents have a positive effect on the macroinvertebrate assemblages in the Pilcomayo floodplain because the highest concentration of nutrients encourages the trophic web that supports the macroinvertebrates.
The greater abundance of Naididae in the Paraná compared to the Pilcomayo River floodplain was expected because these oligochaetes are detritivores on the organic matter retain in the dense root systems and tolerant to low oxygen content. Oligochaeta dominated the macroinvertebrate assemblages during the decomposition of the leaves in the Paraná River floodplain (Poi de Neiff, Galassi, & Franceschini 2009). Rocha-Ramirez, Ramirez-Rojas, Chavez-Lopez, and Alcocer (2007) attributed the abundance of Oligochaeta to detritus retention within the E. crassipes roots. Several families of beetles and bugs can live with low oxygen content by swimming to the surface to renew the air supply carried with them.
Rocha-Ramirez, Ramirez-Rojas, Chavez-Lopez & Alcocer (2007) found a positive correlation between the density of amphipods and dissolved oxygen within the water hyacinth roots. Heleobia parchappii is an opportunistic species that can live in a wide range of salinities, except in anoxia conditions (Tietze 2011). Cyclestheria hislopii is a typical inhabitant of vegetation in shallow and permanent waters and many factors (habitat type, size of the water body, temperature and biotic conditions such as vegetation presence, competition and predation affect the population abundance (Maeda-Martínez, Belk, Barboza, & Dumont 1997).
The prawns M. jelskii and M. amazonicum, collected in the Pilcomayo floodplain, were not found either in the present study or previous studies in the Paraná floodplain (Poi de Neiff & Carignan 1997). Collins (2000) documented the occurrence of M. jelskii in Laguna Blanca, and extends its known range considerably southwards. Thus, the complete absence of these shrimps in the Paraná floodplain is related to its range of distribution rather than water conditions within the floating meadows. Montoya (2003) registered M. jelskii and M. amazonicum in E. crassipes roots growing in the Orinoco delta, with a mean density of 15.34 ± 7.75 ind.m2. The Amazon River prawn M. amazonicum is endemic to South America, where the species extends from Venezuela to Argentina, where isolated inland populations in the Upper Paraná and Paraguay Rivers were found (Pantaleão, Hirose, & Costa 2014).
The species of Macrobrachium sp. are an important component of the food chain in limnetic environments, feeding on detritus, insect larvae, and plant fragments. In wetlands of the northeast of Argentina, H. curvispina acts as collector-gatherer and shredder, consuming detritus (the item with greatest proportion and frequency) and vegetal tissue (Poi, Galassi, Carnevali, & Gallardo 2017).
The littoral cladoceran S. vetulus can evolutionarily adapt to quickly increased temperature (above 4 °C and 8 °C according IPCC scenario A2) in mesocosms experiment with more survival than those reared on cool water (Moss 2010). This species was cited by Heckman (2013) for the Pantanal de Poconé (Cuiaba, Basil) in warm waters.
The differences in the water condition between both floodplains and the root dry weight affect the composition and the dominance of macroinvertebrate assemblages. These differences affect the overall abundance, but not the taxa richness. E. crassipes forms larger floating meadows than other plants in the floodplain area with frequent horizontal flows from/to the river course and provides a unique habitat that contributes to overall biotic diversity, which must be considered in management strategies.
Our results indicate that the roots of the same floating plant (E. crassipes) growing under different environmental conditions may have different assemblages of macroinvertebrates. Forecasts for different Climate Change scenarios in the NE region of Argentina indicate that during this century a 2-3 degree increase in maximum temperatures is expected (Neiff & Neiff 2013). The waters of Laguna Blanca, due to its proximity to the Tropic, were warmer, with little seasonal variability and difference of seven degrees Celsius respect to El Puente oxbow. However the higher temperature of the Pilcomayo floodplain has not a negative impact on the overall abundance per area and species richness of the macroinvertebrates, although affects the composition of the assemblages. According to Moss (2010), some of the change that might be occur as temperature increased, could be in relative abundance of species already present. Exist also the probability of evolutionary change in some organisms, especially the smallest, to the new conditions.
Ethical statement: authors declare that they all agree with this publication and made significant contributions; that there is no conflict of interest of any kind; and that we followed all pertinent ethical and legal procedures and requirements. All financial sources are fully and clearly stated in the acknowledgements section. A signed document has been filed in the journal archives.