Introducción
Oceanic islands are recognized for their great value in developing human understanding of evolutionary processes in marine environments, acting as small “living laboratories” where species adapt and diversify. Where suitable protection measures exist, such islands are also important refuges against biodiversity loss from overexploitation (Robertson, 2001; Losos & Ricklefs, 2009). Island diversity is controlled by the equilibrium between migration and extinction, as well as by speciation rates. These processes are linked to the size of the island and the proximity of the island to the biodiversity source (such as the continent) (Whittaker, Triantis, & Ladle, 2008). Others factors that can influence the species richness of islands are the heterogeneity of the terrain, oceanographic processes, and geological history (Robertson, 2001; Hachich et al., 2015). Gene flow restriction is an important mechanism for the formation and maintenance of reef fish endemism on islands, where the degree of isolation of the island from the main source of diversity input, as well as the age of the island, are key factors in the number of endemic species (Hachich et al., 2015). It is expected that older islands will have more endemic species compared to younger islands, as a result of a longer time over which speciation processes may have acted on them (Emerson & Kolm, 2005).
Isla del Coco is located 600 kilometers South of Puntarenas, Costa Rica and 630 km to the Northeast of the Galapagos Islands of Ecuador; is part of the biogeographic region of the Eastern Tropical Pacific (ETP) (Garrison, 2000; Robertson & Cramer, 2009). Isla del Coco is the largest national park in Costa Rica (Cortés, 2008). Research on the island’s ichthyofauna has focused mainly on reef shark species and species of commercial interest (Cortés, 2012; Cortés et al., 2012; Gonzáles-Andrés, Lopes, Cortés, J., Sánchez-Lizaso, & Pennino, 2016; Fourriére, Alvarado, Ayala-Bocos, & Cortés, 2017). Cryptobenthic fishes are a component of marine ichthyofauna that has been poorly studied due to the difficulties associated with observation, sampling, and identification (Willis, 2001; Ackerman & Bellwood, 2002; Depczynski & Bellwood, 2003). The use of different substance like rotenone, quinaldine or clove oil to sampling cryptobenthic fish reveals species usually undetected with visual methods or other collection techniques; and the richness and diversity is underestimate (Ackerman & Bellwood, 2000; Robertson & Smith-Vaniz, 2008). Cryptobenthic fish have an important role in the reef ecosystem, cycling trophic energy from microscopic or detrital prey items to larger consumers and they can be important indicators to changes in environmental conditions (Bellwood et al., 2012; Brandl, Goatley, Bellwood, & Tornabene, 2018).
The family Gobiesocidae (clingfishes), are small cryptobenthic fishes adapted to live in intertidal zones or rocky coasts. Clingfish are found in marine and freshwater habitats, in temperate and tropical areas (Thomson, Findley, & Kerstitch, 2000). Clingfish are best representative in the ETP with 31 endemic species that Greater Caribbean with 27 species (Robertson & Allen, 2015).
Gobiesox adustus Jordan & Gilbert, 1882 is distributed from the Gulf of California to Ecuador, including the islands Revillagigedo and Marias (México), and Isla del Coco (Costa Rica) (Robertson & Allen, 2015). We evaluated for the first time, variation in the mitochondrial marker cytochrome oxidase sub-unit 1 (cox1) for the Gobiesox adustus population of Isla del Coco and continental populations from Costa Rica and Ecuador. The cox1 gene is widely used for the identification of the species through the International Barcode of Life project (iBOL) (Hebert, Cywinska, Ball, & deWaard, 2003; Hebert, Ratnasingham, S., & deWaard, 2003; Hollingsworth, Graham, & Little, 2011; Al-Rshaidat et al., 2016; Andújar, Arribas, Yu, Vogler, & Emerson, 2018). The sequencing of the cox1 gene has played an important role in the identification of cryptic species and the increase in the rate of new species discovery (Hebert et al., 2003a; Ward, Zemlak, Innes, Last, & Hebert, 2005; Sáenz, 2009; Valdéz-Moreno, Vásquez-Yeomans, Elías-Guitiérrez, Ivanova, & Hebert, 2010). More recently, this marker has been used in a phylogeographic framework to investigate the genetic connectivity or fragmentation along the distribution range of a species (Briggs & Bowen, 2012), to investigate the evolutionary history of species and the current dynamics of the populations. The objectives of the present work were: i) to elucidate if the isolation effect could be affecting the genetic structure of G. adustus and ii) to determine the evolutionary histories of G. adustus from Isla del Coco and southern continental populations and their conservation implications.
Materials and methods
Specimen collection and sampling: Sampling was carried out in rocky intertidal pools on Isla del Coco and in continental sampling locations from Costa Rica and Ecuador (Fig. 1; Table 1). Clove oil and hand nets were used following the protocol of Torres-Hernández et al. (2016). Tissue samples (fin clips) were obtained from each organism, preserved in absolute ethanol, and stored at -75°C. Voucher specimens were deposited in the ichthyological collection of the ‘Universidad Michoacana de San Nicolás de Hidalgo’, Mexico (CPUM, SEMARNAT registration number MICH.PEC-227-07-09) (Table 1).
TABLE 1 Accession numbers for GenBank of each sequence for cox1. Collection information: tissue ID, CPUM ID, sampling location, coordinates and country
Specie | Tissue ID | Collection ID (CPUM) | GenBank access cox1 | Sampling location | Coordinates | Country |
Gobiesox adustus | 21551 | n/d | MN539444 | Pool in Reserva San Miguel, Cabo Blanco, Puntarenas | 9º34’47.6”N, 85º8 ‘12.9”W | Costa Rica |
47056 | 12760 | MN539445 | Tidepool Bahía Wafer, Isla del Coco | 5º32’46.15”N, 87º3 ‘26.69”W | Costa Rica | |
47128 | n/d | MN539446 | ||||
47643- 47660 | n/d | MN539447- MN539464 | ||||
47671 | n/d | MN539465 | ||||
47672 | n/d | MN539466 | ||||
47673 | n/d | MN539467 | ||||
47677 | n/d | MN539468 | ||||
47678 | n/d | MN539469 | ||||
47679 | n/d | MN539470 | ||||
48368 | n/d | MN539471 | ||||
48489- 48511 | n/d | MN539472- MN539494 | ||||
48556- 48563 | n/d | MN539495- MN539502 | ||||
51501 | 12638 | MN539503 | Ligüiqui (Tidepool), Manabi | 0º55’38.2”N, 80º44 ‘25.4”W | Ecuador | |
51503 | MN539504 | |||||
51525 | n/d | MN539505 | ||||
51526 | n/d | MN539506 | ||||
51532 | 12638 | MN539507 | ||||
57338 | n/d | MN539508 | ||||
57339 | n/d | MN539509 | ||||
57340 | n/d | MN539510 | ||||
57363 | n/d | MN539511 | ||||
57369 | n/d | MN539512 |
DNA extraction, amplification, and sequencing: DNA extraction was carried out using the proteinase K and phenol-chloroform protocol (Sambrook, Fritsch, & Maniatis, 1989). The mitochondrial cytochrome oxidase sub-unit 1 gene (cox1: 621 base pair) was amplified with the primers Fish1F/Fish1R (Ward et al., 2005) and LCO1490F/HCO2198R (Folmer, Black, Hoeh, Lutz, & Vrijenhoek, 1994), by means of polymerase chain reactions (PCRs), in 12.5 μl volume reaction containing: 1.25 μl of the buffer (buffer Tris-HCL 10X with MgCl2), 0.3125 μl MgCl2 (50 mM), 0.5 μl of each primer (10 mM), 0.5 μl of dNTPs (10 mM), 0.125 U/μl of Taq DNA polymerase (Invitrogen) and 1 μl (10-60 ng) of DNA template. The thermocycling conditions for Fish1F/Fish1R consisted of an initial denaturation step at 94°C (5 min) followed by 36 cycles at 94°C (1 min) for DNA denaturation, 52°C (1 min) for annealing, and 72°C (1 min) for synthesis, with a final extension at 72°C (10 min). For LCO/HCO we followed Conway et al., (2017). The PCR products were purified with Exo-SAP-IT (USB Corp. Cleveland, OH, USA) and submitted to Macrogen Inc. (Korea) for sequencing. Sequences were manually aligned and examined using chromatograms in MEGA v7 (Kumar, Stecher, & Tamura, 2016). The sequences obtained were deposited in the GenBank database (http://www.ncbi.nlm.nih.gov/gengank/) under accession numbers shown in Table 1. The best-fit model for the data set was calculated in jModeltest v (Posada, 2008) based on the corrected Aikake Information Criterion (AIC).
Haplotype network and genetic diversity: Intraspecific relationships among the samples were inferred through a haplotype network constructed in PopArt v.1.7 (http://popart.otago.ac.nz) applying the Median-Joining method (Bandelt, Forster, & Röhl, 2000). Genetic diversity was assessed by means of the following measures: 1) haplotype diversity (h), 2) nucleotide diversity (π), and 3) segregating sites (S) using DnaSP v5.10 (Librado & Rozas, 2009).
Genetic distance and structure: Levels of genetic differentiation and structure among haplogroups of Gobiesox adustus were estimated using uncorrected pairwise genetic distances (p-distance) calculated in MEGA v.7 with 1 000 bootstrap replicates and using the statistic ΦST (as an alternative fixation index used for haplotype data) by means of pairwise comparisons and analysis of molecular variance (Weir & Cockerham, 1984; Excoffier, Smouse & Quattro, 1992; AMOVA; Dupanloup, Schneider, & Excoffier, 2002) with a significance level of α=0.05 and 1 000 random permutations implemented in the software Arlequin v.3.5.1.2 (Excoffier & Lischer, 2010).
Historical demography: The historical demography of Isla del Coco samples was assessed with the method Bayesian skyline plot (Drummond, Rambaut, Shapiro, & Phybus, 2005)This coalecsent-based methods provide a robust framework to infer past population dynamics through time from a DNA sequences without dependence on a prespecified parametric model of demographic history implemented in BEAST2 (Bouckaert et al., 2014). The analysis was carried out using an uncorrelated lognormal relaxed clock model. Since no mutation rate for cox1 in Gobiesocidae is available, we use the mutation rate available for Gobiidae, a close related group that is part of the Gobiesociformes, of 1.81 to 2.04 % widely used for reef fish species (Keith et al., 2010), using a uniform prior (lower = 0.009 and upper = 0.01 substitutions/site/million year). The webserver CIPRES Science Gateway v. 3.3 (Miller, Pfeiffer, & Schwartz, 2010) was implemented, using the Markov Chain Monte Carlo algorithm (MCMC) run for 50 million generations sampled every 1000 generations. The Bayesian reconstruction graph was carried out in Tracer software v1.4 (Rambaut & Drummond, 2007).
Results
We obtained a partial fragment of the cox1 mitochondrial gene for 69 individuals of Gobiesox adustus from Costa Rica (n=1), Ecuador (n=10) and Isla del Coco (n=58). The length of the alignment was 621 base pairs, of which 566 were invariable, 34 variable, and 27 parsimony informative. The best-fit substitution model for the aligned data was TrN+I.
Haplotype network: The haplotype network resulted in 31 haplotypes divided into three haplogroups (Fig. 2). The individuals of Isla del Coco appear segregated into one haplogroup (haplogroup 3) and did not share haplotypes with samples from the continental coast (Ecuador and Costa Rica). Haplogroup 3 is separated by 11 mutational steps from haplogroup 1, which is formed by samples from Ecuador. Haplogroup 2 is formed by the samples collected in the mainland of Costa Rican and two samples from Ecuador, and is separated by seven mutational steps from the Isla del Coco (haplogroup 3) and 11 mutational steps from haplogroup 2 (Fig. 2). The genetic diversity by haplogroup is shown in Table 2. The genetic diversity was high and the nucleotide diversity was low for the three haplogroups.
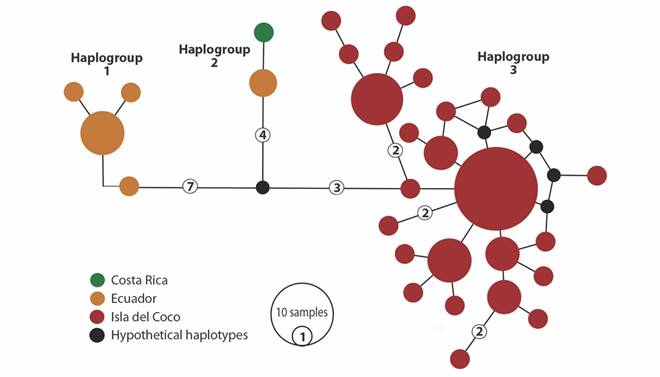
Fig. 2 Haplotype network of Gobiesox adustus based on a partial fragment of the cox1 gene. The size of the circles is proportional to the frequency of each haplotype and colors correspond to sampled areas as show in figure 1. Numbers inside circles correspond to mutation steps, when more than one. Black circles correspond to hypothetical haplotypes.
TABLE 2 Genetic diversity of the three haplogroups found in the network of Gobiesox adustus based on cox1 mitochondrial gene
N | S | nH | h | π | |
Haplogroup 1 (Ecuador) | 8 | 3 | 4 | 0.643 ± 0.033 | 0.00125 ± 0.00044 |
Haplogroup 2 (Costa Rica-Ecuador) | 3 | 1 | 2 | 0.667 ± 0.098 | 0.00322 ± 0.00152 |
Haplogroup 3 (Isla del Coco) | 58 | 21 | 25 | 0.883 ± 0.033 | 0.00443 ± 0.0000002 |
All samples | 69 | 34 | 31 | 0.913± 0.0006 | 0.00883 ± 0.00106 |
N, number of samples; S, polymorphic sites; nH, number of haplotypes; h, haplotype diversity; π, nucleotide diversity.
Genetic differentiation and structure: Pairwise ΦST values among the three haplogroups of Gobiesox adustus showed high and significant values (Table 3). The highest value was between haplogroup 1 (Ecuador) and haplogroup 2 (Costa Rica-Ecuador) (ΦST=0.941). The uncorrected mean genetic distances (p-distances) calculated between haplogroups were also high (Table 3). The p-distance for haplogroup 3 (Isla del Coco) vs haplogroup 1 and 2 was calculated as 2.1%, for haplogroup 3 vs 2 was 1.6%. The AMOVA show significant values for the three haplogroups (ΦST=0.688 P=0.016), and most of the variation were observed among groups (68.84%).
TABLE 3 Differentiation parameters for Gobiesox adustus cox1 haplogruoups. Above diagonal: uncorrected p genetic distances. Below diagonal: genetic differentiation using pairwise ΦST among recovered haplogroups. *Significance value (p < 0.05).
Haplogroup 1 (Ecuador) | Haplogroup 2 (Costa Rica-Ecuador) | Haplogroup 3 (Isla del Coco) | |
Haplogroup 1 (Ecuador) | 2.1% | 2.1% | |
Haplogroup 2 (Costa Rica-Ecuador) | 0.941* | 1.6% | |
Haplogroup 3 (Isla del Coco) | 0.811* | 0.732* |
Historical demography: The Bayesian skyline reconstruction showed a population expansion in the Isla del Coco population of Gobiesox adustus ca. 200 000-150 000 years ago (HDP 95 %) (Fig. 3).
Discussion
Data on the genetic structure of widely distributed fish populations in the Eastern Tropical Pacific (ETP) is scarce and the role played by the oceanic islands of the ETP in this structuring of populations remains poorly studied. In the studies that do exist (i.e., Stethojulis bandaenensis, Porites lobata), genetic differentiation of populations from islands such as Clipperton and Isla del Coco has been recorded (Lessios & Robertson, 2006; Baums, Boulay, Polato, & Hellberg, 2012; Lessios & Baums, 2016). A phylogeographic approach to investigate the evolution of Gobiesox adustus in the ETP with a focus in Isla del Coco is presented herein. This is the first study of the genetic diversity of clingfish from Isla del Coco and to evaluates the time of population expansion in fish species that habitat Isla del Coco.
Independence of evolutionary lineage from Isla del Coco. The genetic structure, high and significant ΦST values, AMOVA results, the presence of unique haplotypes and high uncorrected genetic distances of 1.6 to 2.1% between the Gobiesox adustus from Isla del Coco haplogroup (haplogroup 3) vs haplogroups 1 and 2, show that the Isla el Coco haplogroup is a well-differentiated lineage (Table 3). The consensus genetic divergences value of cox1 widely used to suggest the existence of independent evolutionary units (Blaxter et al., 2005; Domínguez-Domínguez & Vázquez-Domínguez, 2009) and detect cryptic species have been placed at 2.0% (Steinke, Zemlak, & Herbert, 2009; Asgharian, Sahafi, Ardalan, Shekarriz, & Elahi, 2011; April, Hanner, Dion-Côté, & Bernatchez, 2012; Winterbottom, Hanner, Burridge, & Zur, 2014). In this case, the population of G. adustus from Isla del Coco, suggests an independent evolutionary unit and could represent a discreet speciation process. Similar genetic differentiation values were found for several populations in marine fish species as in Chysiptera (Steinke et al., 2009), Trimma (Winterbottom et al., 2014), Bathybogius (Tornabene, Baldwin, Weigt, & Pezold, 2010), Macrourus (Smith et al., 2011), Inegocia (Puckridge, Andreakis, Appleyard, & Ward, 2013) were divergent haplogroups were recognized as provisional candidate species based in the >2% differences in the cox1 gene; in some cases the genetic results were consistent with minor taxonomic distinctions based on morphology (Quattro et al., 2006; Puckridge et al., 2013; Winterbottom et al., 2014) and even some well recognized marine fish species have genetic divergences lower than 2% (Ward et al., 2005, Lowenstein, Amato, & Kolokotronis, 2009) or different lineages Argyrops spinifer (Asgharian et al., 2011). As such, we propose that Gobiesox adustus population from Isla del Coco must to be considered as independent evolutionary linage pending of more deep study including more samples along the distribution range and integrative taxonomic analyses.
Significant differences in mtDNA frequencies between populations of Gobiesox adustus could be produced by a combination of the life history traits and oceanographic conditions. Data on the life history of G. adustus, such as its reproductive period, or the pelagic larval duration (PLD) are unknown. In general, a short PLD has been estimated for clingfish (i.e., 13-14 days) (Beldade, Pedro, & Gonçalves, 2007). Several studies have shown that PLD has a positive correlation with the range of distribution of some species (Riginos & Victor, 2001; Robertson, 2001; Macpherson & Raventos, 2006; Kelly & Palumbi, 2010). However, for other species this relationship is not as clear (Victor & Wellington, 2000). Some studies in the Gulf of California show all stages of developing clingfish larvae can be found near reefs, indicating strong larval retention in and around the reef habitat (Brogan, 1994; Beldade et al., 2007). These characteristics of clingfish can maintain self-recruitment and, as a result, promote genetic differentiation in oceanic island populations, as in the case of G. adustus from Isla del Coco presented herein.
Another factor that could affect clingfish populations are the oceanographic processes that may reduce gene flow, generating significant genetic differentiation in populations. Oceanographic processes (i.e., currents and cyclonic/anticyclonic gyres) have a great influence on the marine ecosystems of the ETP and can promote retention or concentration of pelagic larvae, affecting the dispersal and the recruitment of fish species (Aceves-Medina et al., 2004; Contreras-Catala, Sánchez-Velasco, Lavín, & Godínez, 2012; Pantoja, Marinone, Parés-Sierra, & Gómez-Valdivia, 2012). Isla del Coco is influenced by the North Equatorial Countercurrent (NECC), the dynamic processes of the Costa Rica Dome (CRD), the Peruvian upwelling, and an anticyclonic circulation to the south of the island (Lizano, 2008). Accordingly, the short larval duration, the isolation of the oceanic Isla del Coco and the abovementioned oceanographic conditions, may restrict gene flow between of populations from Isla del Coco and the continent (Fig. 2), by limiting G. adustus dispersal.
All these processes play a fundamental role in the genetic structure of species associated with rocky reefs in the ETP (Hastings, 2000; Sandoval-Huerta et al., 2018), and particularly in oceanic island species, as is the case of Epinephelus labriformis and E. clippertonensis were private haplotypes of the Clipperton Athol and Alijos rocks populations indicate gene flow restriction between Clipperton Atoll, Alijos Rocks and the mainland due to oceanographic conditions, concluding that the formation of this sister species is a case of incipient speciation in the marine environment (Craig, Hastings, Pondella, Robertson, & Rosales-Casián, 2006). Similar scenarios are present in the marine populations of other islands (Cunha et al., 2011) and Cape Mendocino, US, were the south-flowing California current, strong upwelling jets and seasonal offshore currents may form a barrier that has a structuring effect on some marine populations (Kelly & Palumbi, 2010).
Historical demography: The changes in climate that occurred in the Pleistocene directly influenced changes of sea levels (Shen, Jamandre, Hsu, Tzeng, & Durand, 2011), and this changes had a great influence on the contemporary distribution, abundance and evolutionary histories of a large number of species, including fish (Grant & Bowen, 1998; Thacker, 2004; Barber & Bellwood, 2005; Floeter et al., 2008; Kašparová, Van de Putte, Marshall, & Janko, 2015), generating a genetic footprint on affected species (Hewitt, 2000; Avise, 2001). In Gobiesox adustus of Isla del Coco, the star-like haplotype network, high haplotype diversity, and low nucleotide diversity support a population expansion scenario, with, expansion dates calculated by the Bayesian Skyline Plot analysis at 200 000-150 000 years ago (95%HPD) (Fig. 3). This period corresponds to the Middle Pleistocene, a time marked by the beginning of an interglacial period (240-190 kya), followed by the Illinoian glacial period (190-125 kya), with sea levels as low as -130m below present during Illinoian (Rohling et al., 1998). During periods of falling sea level, coastline geomorphology is modified and islands increase in area (Graham, Dayton, & Erlandson, 2003) providing extra habitats for benthic species, which frequently promote the increase in effective population size in marine species (Marko et al., 2010; Cunha et al., 2011). For Isla del Coco have been estimated that the actual shallow habitat (<50 m deep) is 14 km2, being much higher during periods of falling sea level (110 Km2) (Robertson, 2001). Accordingly, since the estimation of the demographic expansion in G. adustus from Isla del Coco coincide with the Illinoian glacial period with sea levels as low as -130m below present, we expect a scenario were the habitat availability in the island increase considerably, favoring the population expansion of G. adustus populations.
Conservation implications: The knowledge about the natural populations, at different levels, from the genetic diversity of the populations to the specific richness of an ecosystem, should be an important part of the decision making process for the protection and conservation of species and areas, moreover in populations living in natural protected areas. Conservation efforts should not be limited to species of commercial interest; it is also necessary to include highly diverse ecosystems, such as reefs or intertidal zones (Perkins, Ng, Dudgeon, Bonebrake, & Leung, 2015). The information presented herein, of an evolutionary distinct population of G. adustus from Isla del Coco should be taken in consideration in the conservation desertions for Isla del Coco National Park, specifically for the importance of the conservation of intertidal zone to maintain the entire diversity of the park (Rocha, Craig, & Bowen, 2007; Domínguez-Domínguez & Vázquez-Domínguez, 2009). Robertson (2001) proposed that small oceanic islands (like Isla del Coco) be considered as small reserves as they represent hotspots of diversity and endemism, in this case, the use of genetic information, such as that presented herein, allows the identification and help in the establishment of marine reserves and protection priorities of endemism and diversity hotspots (Jones, Srinivasan, & Almany, 2007; Almany et al., 2009; Planes, Jones, & Thorrold, 2009; Drew & Barber, 2012).
The strong population differentiation for Gobiesox adustus in Isla del Coco is influenced by life history traits and the oceanographic conditions. Further study and conservation of the G. adustus population of Isla del Coco will be important as part of conserving the biodiversity of the oceanic islands of the ETP. There is still a lot of work to be done, with respect to the populations of G. adustus, in particular it is recommended to perform integrative taxonomic study in order to elucidate the distinctiveness of this population.
Ethical statement: authors declare that they all agree with this publication and made significant contributions; that there is no conflict of interest of any kind; and that we followed all pertinent ethical and legal procedures and requirements. All financial sources are fully and clearly stated in the acknowledgements section. A signed document has been filed in the journal archives.