Introduction
Seed dispersal by animals (zoochory) is an important interaction that have a fundamental role in the life cycle of plants, influencing their biology, ecology, genetics and evolution (Herrera, 2002; Correa, Winemiller, López-Fernández, & Galetti, 2007; Pollux, 2011) and contribute to the biological and functional diversity of plant communities (Aslan, Zavaleta, Tershy, & Croll, 2013), especially in those dominated by animal-mediated dispersal (Schupp, Jordano, & Gómez, 2010). This interaction can be disrupted or affected by habitat loss (Goulding, Smith, & Mahar, 1996), high deforestation rates, as occurs in the Amazon basin (Armenteras, Rudas, Rodríguez, Sua, & Romero, 2006) and land use changes (Murcia et al., 2014), since the loss of natural vegetation reduces the food supply for these animals, which in turn reduces the possibility of seed dispersal.
Fish were probably one of the first agents to disperse seeds, a phenomenon that possible started in the Carboniferous about 300 million years ago (Tiffney, 1984). Up to date, over 270 species of fish have been reported to consume fruits and seeds (Horn et al., 2011; Beaune et al., 2013; Correa et al., 2016), most of these inhabiting the floodplains of the Neotropics (Horn et al., 2011) and feeding on more than 150 plant species (Correa et al., 2007). In a recent report, Correa, Costa-Pereira, Fleming, Goulding, & Anderson (2015a) increase this diversity of florae whose fruits and seeds are consumed by Neotropical fish, to at least 566 plant species. Since an enormous amount of seeds from a high diversity of plants have been encountered in the stomachs of frugivorous fish, it is thought that ichthyochory (seed dispersal by fish) is paramount for seasonally flooded forest regeneration (Goulding, 1980; Anderson, Rojas, & Flecker, 2009). Some research has shown that larger fish increase the effectivity of seed dispersal and viability and disperse seeds farther from their origin due to long retention times in their digestive tracts (Galetti, Donatti, Pizo, & Giacomini, 2008; Anderson et al., 2009; Anderson et al., 2011), but also because they consume more seeds in terms of diversity, number and biomass (Galetti et al., 2008; Anderson et al., 2009; Correa et al., 2015a; Correa et al., 2015b; Correa et al., 2016). However, several germination experiments of seed ingested by fish have given contrasting results regarding seed germination enhancement (Pollux, 2011) and in part this could be attributed to large variation in the experimental design and protocols (Costa-Pereira, 2017).
Colossoma macropomum Cuvier, 1818 is widely distributed throughout the Amazon and Orinoco Basins (Mago-Leccia, 1970; Mojica, Usma, Álvarez, & Lasso, 2012) and present morphological and behavioral adaptations for fruit and seed consumption (i.e. molar-like teeth, long intestines and extended retention times) (Goulding, 1980; Correa et al., 2007; Anderson et al., 2011). These species feeding and its migratory behavior are closely related to the flooding season (Oliveira, Martinelli, Moreira, Soares, & Cyrino, 2006). When water levels are high, adults move to smaller tributaries and floodplains to consume a large number of seeds and fruits of almost a hundred plant species (Goulding, 1980; Kubitzki & Ziburski, 1994; Araujo-Lima & Goulding, 1997; Lucas, 2008; Correa et al., 2015a) allowing fat accumulation (Junk, Soares, & Saint-Paul, 1997), which is essential for subsequent reproduction of Colossoma (De Almeida, Avilez, Honorato, Hori, & Moraes, 2010). Despite its key ecological function, C. macropomum has been underlined as keystone species in commercial and artisanal fisheries in the Amazon basin. However, over the last decades stocks have declined by 90 %, yielding significantly smaller fish and altering structure of populations (IBAMA, 2005; Santos, Ruffino, & Farias, 2007). Costa-Pereira, Lucas, Crossa, Anderson, Weiss et al. (2018) have hypothesized that the C. macropomun’s key ecological function as a seed disperser has decreased in the Manaus region (Brazilian Amazon) by the striking fisheries-induced downsize. In Colombia, this species is classified as Near Threatened (NT) (Mojica et al., 2012) and regardless its biological, economic and social importance there are no data on the level of consumption and commerce of this fish species in the Colombian Amazon basin (Agudelo et al., 2011).
Cecropia latiloba Miq. and Cecropia membranacea Trécul. (Urticaceae) are broadly distributed in the northern parts of South America, especially along the floodplains of white-water rivers of the Amazon and Orinoco basins (Franco-Rosselli, & Berg, 1997; Parolin, 2002; Berg, Franco-Rosselli, & Davidson, 2005). Cecropia species are recognized as pioneers in the dynamics of tropical forests, since they have an abundant and almost continuous seed production, their seeds retain a high latency and have a very rapid growth (Hartshorn, 1980). Cecropia spp. trees colonize different habitats that are generated by the erosional and depositional activity of rivers (Salo et al., 1986) and natural forest gaps (Parolin, 2002; Parolin, 2003; Parolin, Oliveira, Piedade, Wittman, & Junk, 2002), thereby initiating natural succession leading to forest diversity (Worbes, Klinge, Revilla, & Martius, 1992; Parolin, 2002). In the Amazonas, Mesquita et al. (2001) points out that natural disturbance, like flooding of upland low terrains, can result in communities dominated by pioneers such as Vismia or Cecropia; these authors add that successional stages dominated by Cecropia can result in forest mosaics with greater age heterogeneity and a more continuous spatial distribution. Additionally, Cecropia fruits have been reported as part of the diet of C. macropomum (Kubitzki & Ziburski, 1994; Anderson et al., 2009, 2011; Weiss, Zuanon, & Piedade, 2016). C. latiloba and C. membranacea have their peaks of flowering and fruiting in the flooded period, but fruits are mature mainly at the end of the high-water phase, in July and August (Kubitzki & Ziburski, 1994; Parolin, 2002).
To increase our understanding of the role of C. macropomum as a seed disperser of two pioneer Amazonian plant species, we conducted a series of controlled experiments to test the hypothesis that the passage of C. latiloba and C. membranacea seeds through the digestive tract of C. macropomum enhances their germination. Specifically, we wanted to determine: 1) how the germination variables of the seed were affected by passing through the digestive system of the fish, and 2) changes in the growth presented by the seedlings from the germinated seeds of each treatment.
Materials and methods
Source of seeds: Cecropia fruits were obtained along the lakeshore of the Yahuarcaca lake system (4°11'16" S - 69°58'16" W) at 82 m in elevation. This lake system has 17 lagoons that are connected by natural channels. It is located 2 km west of Leticia, the capital of Amazonas Department in Colombia, and has a direct and constant connection with the Amazon River that particularly influences the lake system from December to April during the season of high-water flooding (Andrade-Sossa, García-Folleco, Rodríguez-Munar, Duque, & Realpe, 2011; Salcedo-Hernández et al., 2012). C. macropomum occurs naturally in this area and feeds on Cecropia and other fruits (Mojica et al., 2012). Mature fruits of both Cecropia species were collected manually. Specialists at the Instituto Amazónico de Investigaciones Científicas (SINCHI) identified the species.
Feeding trials: live specimens of C. macropomum were bought at a local fishery located 10 km North of Leticia. They were between 29 and 43 cm total length and we placed them in 10 l plastic bags for transportation to the “Laboratorio de Semillas y Recursos Naturales” (Seeds and Natural Resources Laboratory) at the Universidad Nacional del Colombia, Leticia (96 m altitude, 26 °C mean air temperature), where we conducted the experiments. The fish did not remain in the bags more than 40 min and there was no mortality during this process. We carried out trials between March and July of 2015.
In total 60 fish were used, 30 for seeds of each Cecropia species. The seeds of each plant taxon were subjected to four treatments: 1) “fish”: seeds underwent a complete passage through the digestive tract, 2) “stomach”: fruits were consumed but seeds did not have a complete passage through the digestive tract, and were removed directly from the stomachs of sacrificed fish, 3) “washed”: the fruits were washed to remove the pulp from the seeds through mechanical action, helping the process of scarification, and 4) “pulp”: the seeds were taken directly from the fruit with the pulp intact (3 and 4 were control treatments). For treatments 1 (defecated seeds) and 2 (seeds extracted from the digestive system) the same fish were utilized. For this set of the two treatments three replicates were made (three trials), in each of which 10 individuals of C. macropomun were used. For treatments 3 and 4 (without fish) three replications of the germination tests were also made.
For each trial of 10 fish, we introduced them into a 4 000 l PVC tank (INTEX, China). Two tanks were used (one for C. latiloba and another for C. membranacea), each equipped with a heating system composed of four heaters (SOBO H300 300W, China) set at 23 °C and a continual water circulation system using two 1 000 l h-1 pumps (RESUN sp-1800, China). Water was constantly oxygenated using a 280 l h-1 air pump (ACTIVEAQUA AAPA70L, China), and two 360 l h-1 filters (AQUEON 06090, China). Every two days, the pH was measured with indicator bands (Merck McolorpHast, USA) that showed neutral conditions (pH ≈ 7) throughout the experiments. Temperature was monitored periodically at 9:00 and 19:00 every day with two thermometers (LongQi LQ-08, China) at the tank’s bottom and surface. Mean water temperature was 23 ± 2.8 °C, very similar between surface (23.8 °C) and bottom (23.04 °C). Both tanks were covered with nets to prevent predation and human intervention. After the fish were introduced, every four days 500 l were renewed to maintain optimal quality.
Before start the experiments, feeding of the fish was suspended for four days to ensure complete evacuation of the digestive tract and to promote feeding activity. On the fifth day, the feeding began with abundant quantities of fruits of the corresponding Cecropia species. After eight days of constant feeding, the C. macropomum specimens were removed from the tanks and sacrificed with thermal shocks by placing them in a refrigerator at 4 °C. For each fish the standard length (SL), total length (TL), intestine length (IL) and total weight (W) were measured. Digestive tracts were removed with caution to avoid damage. Stomach and intestinal contents were analyzed using a stereomicroscope to check for possible damage to the seeds. Water was totally replaced before the beginning of the replications for each experiment.
Defecated seeds lost their capacity for buoyancy and were collected at the bottom of the pool while uneaten seeds and fruits kept floating. Bottom and floating seeds were collected daily with a small net and transported to the laboratory. Before the germination trials we measured seed lengths and widths with a Vernier caliper in order to contrast the size of seeds with the size of fish. All applicable institutional and national guidelines for the care and use of animals were followed. All mandatory laboratory health and safety procedures were followed during the course of conducting the experiments.
Germination: seeds collected from the trials were placed in Petri dishes, on moist absorbent paper and exposed to sunlight. Each Petri dish held a maximum of 30 seeds, some Petri dishes held less seeds whenever the maximum number could not be completed. Every two days we applied a commercial 1 % chlorine solution to control the appearance of fungi, following the procedure performed by Naranjo, Rengifo, & Soriano (2003). We inspected each Petri dish daily over a minimum of four consecutive days to detect germinated seeds. We counted and retrieved these until there was no further germination (Naranjo et al., 2003). We defined “germination” as the emergence of the radicle through the seed coat (Salisbury & Ross, 1992). A stereomicroscope was used to observe the emergence of radicles. A total of 10 214 seeds were used, distributed per treatment as shown in Table 1.
TABLE 1 Number of seeds of the Cecropia species used in the germination treatments
Plant species | Replica | Treatments | |||
Washed | Pulp | Fish | Stomach | ||
C. latiloba | 1 | 210 | 210 | 192 | 484 |
2 | 140 | 420 | 1 600 | 72 | |
3 | 700 | 630 | 1000 | 63 | |
Total | 1 050 | 1 260 | 2 762 | 619 | |
C. membranacea | 1 | 450 | 400 | 560 | 160 |
2 | 240 | 500 | 400 | 210 | |
3 | 450 | 400 | 610 | 143 | |
Total | 1 140 | 1 300 | 1 570 | 513 |
Seeds of treatments “Washed” an “Pulp” did not have transit through the digestive system of the fish
We assessed five parameters: 1) germination capacity (GC), namely the percentage of germinated seeds under each treatment, 2) minimum imbibition time (Tmi), that is the minimum time in days required for the first seed to start germinating, 3) time in days necessary for reaching 50 % germination capacity or mean germination time (T50) (Naranjo et al., 2003), 4) emergence velocity index (EVI) in days, which is the average number of days it takes the seeds to germinate after sowing, and 5) germination mean time (GMT) in days, that is the average number of days it takes the seeds to germinate since the beginning of germination (Ruiz & Terenti, 2012).
Seedling emergence percentage: we selected five germinated seeds from each treatment to be sown in plastic cups with soil taken from the farm at the Universidad Nacional de Colombia, Leticia. These were watered every three days and the seedlings growth was measured every five days for two months using a Vernier caliper. We considered the seedling emergence percentage and the seedlings growth as measures of seeds capacity to establish, continued to grow after emergence, and developed into plants (Hanson, 1985).
Statistical analysis: we calculated means and standard deviations for the morphometric parameters of the C. macropomum specimens and for the seed measurements and heights reached by the seedlings of both Cecropia species. The normality of germination data (GC, EVI and GMT) was tested with a Shapiro - Wilk analysis; the percentage values of germination capacity were previously transformed using the arcsine function. We carried out one-way analysis of variance (ANOVA) in order to evaluate the significance of the differences between the germination treatments. Non-parametric Kruskal-Wallis analysis was realized to evaluate the significance of the differences in sizes reached by the seedlings. Statistical analysis and figures were made with R 3.1 (R Core Team, 2015).
Results
Both the averages of standard length and total length of the fish were similar in the treatments of the two plant species, with standard deviations of a few centimeters. Fish intestine length was almost two times the standard length (Table 2). Seeds of C. latiloba tended to be more elongated (3.25 x 1.89 cm) than those of C. membranacea (2.87 x 2.01 cm); dimensions of the seeds were within narrow ranges of variation (Table 2). The measurements of the employed fish are presented in a supplementary file.
TABLE 2 Morphometric parameters of Colossoma macropomum individuals and seed length and width (mean ± SD) of Cecropia species used for feeding trials
Plant species | n | Fish | Seeds | |||||
SL range | SL | Tl | IL | W | Length | Width | ||
(cm) | (cm) | (cm) | (cm) | (kg) | (mm) | (mm) | ||
C. latiloba | 30 | 29 - 38 | 32.9 ±2.41 | 37.73 ± 2.72 | 68.26 ± 4.68 | 2.48 ± 0.32 | 3.25 ± 0.388 | 1.89 ± 0.268 |
C. membranacea | 30 | 30 - 43 | 34.83 ± 3.39 | 39.93 ± 3.76 | 75.36 ± 11.34 | 2.67 ± 0.46 | 2.87 ± 0.213 | 2.01 ± 0.234 |
Thirty individuals of C. colossoma were used in the trials of each Cecropia species.
n= number of fish, SL= standard length, TL= Total length, IL= intestine length, W= Weight of entire fish.
Germination: the germination capacity for C. latiloba seeds varied for each treatment. Seeds with the higher values were those completing digestive tract transit and those that retained the pulp (Table 3, Fig. 1). Seeds submitted to mechanical treatment showed medium germination values and those retrieved directly from the stomach had greater reduction in the third replication (Fig. 1). Seeds that passed completely through the digestive tract had the highest accumulative percentages, with a mean of 86.12 % (Table 3). The control treatments (seeds with pulp and without pulp by mechanical washing) showed similar values for the first and second replications, but on the third one the accumulative percentage of germination decreased in washed seeds (Fig. 1). The ANOVA of the germination capacity showed no statistical differences between treatments (P = 0.0916). Minimum imbibition time and germination mean time in defecated seeds of C. latiloba had similar values to the other treatments (Table 3). Seeds extracted from the stomachs germinated less and slower. Although seeds of fish-treatment showed higher values of the emergence velocity index (5.7) and of the germination mean time (3.96), these differences were not significant (pevi = 0.292; pgmt = 0.63).
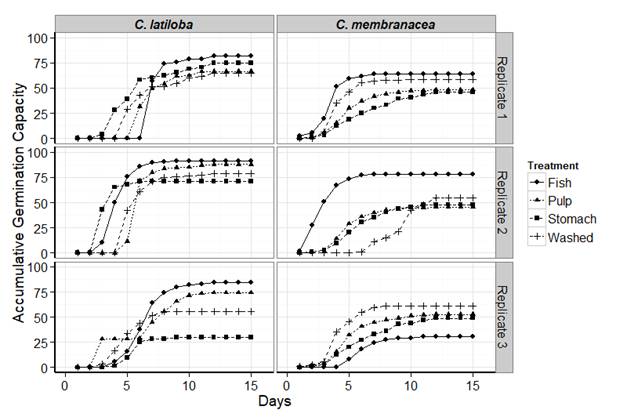
Fig. 1 Accumulated germination capacity (percentage) of Cecropia latiloba (left) and Cecropia membranacea (right) seeds under the different treatments.
TABLE 3 Means and standard deviations of the seed germination analysis for both Cecropia species and for each treatment
Treatment | ANOVA TEST | ||||||||
Species | Parameter | n | Washed | Pulp | Fish | Stomach | F-values | df | P-value |
GC - % | 3 | 67.14 ± 12.22 | 77.45 ± 9.78 | 86.12 ± 4.71(*) | 58.71 ± 24.82 | 3.059 | 3 | 0.0916 | |
Tmi - days | 3 | 4 | 4 | 4 | 3 | ||||
C. latiloba | T50 - days | 3 | 5 | 6 | 6 | 4 | |||
EVI - days | 3 | 4.48 ± 2.84 | 5.46 ± 1.17 | 5.7 ± 0.66 | 2.67 ± 2.33 | 1.481 | 3 | 0.292 | |
GMT - days | 3 | 2.48 ± 1.3 | 2.44 ± 1.86 | 3.96 ± 1.6 | 2.58 ± 1.66 | 0.606 | 3 | 0.63 | |
GC - % | 3 | 58.05 ± 3.36 | 48.83 ± 3.66 | 57.74 ± 24.15 | 47.92 ± 1.46 | 0.655 | 3 | 0.602 | |
Tmi - days | 3 | 4 | 3 | 3 | 4 | ||||
C. membranacea | T50 - days | 3 | 6 | 5 | 4 | 6 | |||
EVI - days | 3 | 2.58 ± 1.03 | 3.13 ± 0.18 | 3.33 ± 2.35 | 2.91 ± 0.29 | 0.182 | 3 | 0.906 | |
GMT - days | 3 | 2.28 ± 0.85 | 1.46 ± 0.11 | 1.46 ± 0.16 | 3.14 ± 2.08 | 1.526 | 3 | 0.281 |
Seed coming from the C. macropomum feeding treatments were “Fish” and “Stomach”. Seeds of treatments “Washed” an “Pulp” did not have transit through the digestive system of the fish.
GC = germination capacity, Tmi= minimum imbibition time, T50= time necessary for reaching 50 % of germination capacity, EVI = emergence velocity index, GMT= germination mean time.
For C. membranacea, the highest germination capacity was for the washed seeds (58.05 %) and the second percentage (57.74 %) was for the evacuated seeds (Table 3). Seeds collected from the stomachs had lower germination capacity, similar to those of seeds with pulp (Table 3). The accumulative germination capacity of the seeds with complete digestive tract transit displayed an improvement (70 %) in the two first replications, despite a clear decrease in the third one (Fig. 1). Meanwhile, the seeds taken directly from the stomach showed values close to 45 % in the three replications (Fig. 1). Among the treatments without digestive tract transit, the washed seeds had an accumulative germination capacity of 60 %, higher than seeds with pulp (48 %) (Fig. 1). However, the ANOVA showed no statistical differences (P = 0.602). The emergence velocity index of evacuated seeds of C. membranacea was also higher (3.33), but the germination mean time (1.46) was lower, as compared to the other treatments. The minimum imbibition time was similar for all treatments, although seeds evacuated by fish and seeds with pulp increased their germination velocity (Table 3). Despite the fact that the seeds collected from the fish’s feces had a higher emergence velocity index and required less time to germinate (Table 3), the differences with the control treatments were not significant (pevi = 0.906, pgmt = 0.281).
Seedling emergence percentage: for both plant species, there was 100 % of seedling emergence. The seedlings from C. latiloba prevenient of seeds with complete digestive transit were more elongated 65 days after sowing (Table 4, Fig. 2). The Kruskal-Wallis test showed significant differences (H = 13.98, P < 0.0001). The seedlings from the C. latiloba seeds without pulp (washed) reached a greater height with respect to the seeds with pulp, as confirmed by the Kruskal-Wallis test (H = 14.06, P < 0.0001). Similarly, seedlings of C. membranacea from seeds with partial or total digestive transit reached greater heights after 65 days (H = 13.06, P < 0.0001) (Table 4). Of the two treatments without seed ingestion, seedling from seeds with pulp removing grew more (H = 13.02, P < 0.0001).
TABLE 4 Mean length (mm) and standard deviations of seedlings for both Cecropia species at each treatment
Species | Treatments | Kruskal-Wallis test | ||||||
n | Washed | Pulp | Fish | Stomach | Х 2 | df | P-value | |
C. latiloba | 5 | 103. 93 ± 5.44 | 87 ± 4.61 | 109.12 ± 6.57 | 109. 73 ± 7.65 | 13.98 | 4 | p<0.0001 |
C. membranacea | 5 | 98.61 ± 8.54 | 100.93 ± 13.01 | 109.93 ± 7.04 | 108.44 ± 6.01 | 13.06 | 4 | p<0.0001 |
Seed coming from the C. macropomum feeding treatments were “Fish” and “Stomach”. Seeds of treatments “Washed” an “Pulp” did not have transit through the digestive system of the fish.
Discussion
Our study shows that C. macropomum juveniles can potentially play a role in the dispersal of Cecropia spp. seeds. However, to what extent this role is important or not is currently unknown and will require further investigation. Hitherto, our results showed certain positive tendency in germination enhancement without loss of germination potential during digestive tract retention, and developed seedlings from defecated seed attained longer lengths in comparison with seeds under other treatments. Moreover, seeds did not suffer damage in the stomach and they were viable after intestinal evacuation of the fish. Nonetheless, the real effectiveness of these juvenile fish as dispersers will require repetitive studies with a greater number of specimens.
It is expected that the fish used in our experiments had digestive tracts shorter than adults, so that the retention time of the seeds could be lower, compared to grown-up fish that can reach over 90 cm (Mojica et al., 2012). Longer intestines allow better seed coat scarification and increase pulp removal due to longer retention times (Mannheimer, Bevilacqua, Caramaschi, & Rubio-Scarano, 2003; Galetti et al., 2008; Anderson et al., 2009, 2011; Correa et al., 2015a; Correa et al., 2015b). The results of our germination experiments did not show any statistical differences between the treatments, but it is not unreasonable to assume that the passage through the digestive tract, even in juvenile fish, could stimulate the end of dormancy, eliminate germination inhibitors in seed pulp and promote the scarification of the seeds. These effects should allow a rapid absorption of water by the seeds that achieve an expeditious development of the embryo, consequently enhancing germination.
Our germination results were similar to those of Horn (1997), Pilati, Andrian, & Carneiro (1999), Da Silva, Pereira-Filho, & De Oliveira-Pereira (2003) and Mannheimer et al. (2003), who used alike conditions as our study. Although they evaluated different species, it is important to contrast the patterns in the responses of germination of seeds consumed by fish. First, seeds retained their germination viability after passing through the digestive tract of fish, similarly to what happened with the seeds of C. latiloba and C. membranacea consumed by C. macropomum. Second, the seeds of Cecropia spp. extracted from stomachs showed no significant difference in germination rates of those seeds remove from the posterior portion of the intestine, a result like that found in our study. However, the effects appear to be more pronounced in the final portions of the intestine (Pilati et al., 1999), which could indicate that the passage through the entire tract favors a greater scarification of the seeds.
C. latiloba and C. membranacea produce a great number of seeds, have a wide distributional pattern, frequently bear fruits (Franco-Rosselli, & Berg, 1997; Parolin, 2002; Berg et al., 2005), and have been reported in high numbers in the C. macropomum diet (Goulding, 1980; Kubitzki & Ziburski, 1994; Anderson et al., 2011). In some cases, Cecropia seeds are retained in the stomach for periods longer than 150 hours and fish movements along the longitudinal and lateral gradient exceed 200 m (Anderson et al., 2009; Anderson et al., 2011). As in our germination trials, Goulding (1980), Mannheimer et al., (2003) and Anderson et al., (2009); Anderson et al., (2011) found that seeds of different Cecropia species can germinate after consumption by C. macropomum (and other fish species), which emphasizes that seed survival is not affected when consumed by this fish. Given their long retention times, the number of seeds consumed, the viability of the seeds after the immersion periods and the distances traveled, adults of C. macropomum act as effective seed dispersers for these plant species (Kubitzki & Ziburski, 1994; Araujo-Lima & Goulding, 1997; Da Silva et al., 2003; Anderson et al., 2011; Correa et al., 2015a). For juvenile fish like we used, it is likely that this dispersive function is also fulfilled, at least partially.
According to Pollux (2011), the causes of the contrasting germination results in the experiments with seeds ingested by fish are not clear, but it possibly could be attributed to the low number of replicas, small sample size, and the source of the seeds, seed storage and morphological and physiological features of fish. These aspects should be explored in greater detail in future studies. With a greater number of data, a logistic regression could be performed, which would allow modeling the probability of seed germination as a function of treatments. The juvenile state of the fish can also influence, as previously mentioned. Although these might be some of the reasons for the lack of significant differences among our treatments, we believe that juveniles may have some role in seed dispersal. The function of juveniles could be even more relevant, since populations of C. macropomum have been reported to have been overexploited and declining for more than 30 years (Petrere, 1983; Santos et al., 2007), probably longer since the decline of the rubber bloom at the beginning of the 20th century and the consolidation of fisheries as replacement activity in the Amazon basin (Araujo-Lima & Goulding, 1997; Lasso et al., 2011). According to Garcez & Freitas (2011) and Campos, Costa Sousa, Catarino, De Albuquerque Costa, & Freitas (2015), most of the catches of C. macropomum unloaded by commercial fisheries correspond mainly to young individuals; therefore, the range of sizes of the fish used in our experiments resembles what is currently found in overexploited areas. This aspect raises important conservation implications for the future of this species and its biological function in Neotropical ecosystems.
In summary we observed that complete transit through the digestive tract of juveniles of C. macropomum does not negatively affect the germination of Cecropia seeds. Although we did not detect significant differences in the success of germination and the speed of emergence between the seeds consumed or not by C. macropomum, it is likely that this species could contribute to the dissemination of Cecropia seeds. Seeds evacuated in fish feces may have the ability to settle along the banks of rivers and flooded forests, where they could germinate in forest gaps stimulating colonization of new areas near water bodies. This can stimulate the succession, regeneration, conservation and maintenance of the forest. We know that larger fish can disperse seeds over longer distances (Anderson et al., 2011), so the reduction in the average size of overfished stocks can diminish the effectiveness of this seed dispersal. Overexploitation of natural populations of C. macropomum could have some indirect effect on the establishment of the plants dispersed by this fish, as in the case of Cecropia spp.; however, it is probable that seed consumption of these plants by other fish, bats, monkeys and bird species might complement the dispersal role of C. macropomum, since these plant species are known as foods of these vertebrates (Lobova, Mori, Blanchard, Peckham, & Charles-Dominique, 2003; Russo, Campbell, Dew, Stevenson, & Suarez, 2005). In that case, ecosystems may be resilient to the loss of a particular frugivorous, but since large-bodied fishes have a greater range of seed dispersal, this resilience may be reduced if such larger fishes are overfished (Correa et al., 2016). For this reason, further studies on C. macropomum populations are required and better measures and conservation policies to protect this important fish species should be implemented.