Introduction
Numerous sticky plants have glandular trichomes (GTs) that secrete viscous-adhesive fluids to entrap insects and other arthropods (Wheeler & Krimmel, 2015). These structures are epidermal hairs with the capacity to secrete or store secondary metabolites and other compounds (Huchelmann, Boutry, & Hachez, 2017). Leaves, stems, and flowers of many vascular plants exhibit GTs and a variety of adaptive functions have been postulated, including solar UV A/B protection, toxin secretion, and anti-herbivore defense (Wagner, Wang, & Shepherd, 2004; Glas, Schimmel, Alba, Escobar-Bravo, Schuurink, & Kant, 2012). Typically, GTs are multicellular structures with differentiated basal, stalk and apical cells. The production and storage compartments are usually located at the tip of the hair and are part of one or more glandular cells (Tissier, 2012). GTs may release the stored exudate by physical rupture or secrete it through the tip opening, causing the plant surfaces to become sticky (Falara & Pichersky, 2012). Exudates are chemically diverse and include terpenoids, flavonoids, phenylpropanoids, alkaloids, fatty acid derivatives and acylated sugars which can confer mucilaginous or resinous properties (Werker, 2000). The effectiveness of GTs in plant defense against herbivory notwithstanding (Levin, 1973; Wagner, 1991; Peiffer, Tooker, Luthe, & Felton, 2009; Tian, Tooker, Peiffer, Chung, & Felton, 2012), there are specialist arthropods which can move across the sticky plant surfaces without becoming entrapped (Voigt & Gorb, 2008, 2010). Species of spiders, plant bugs and stilt bugs are examples of invertebrates that colonize sticky plants for consuming both living and dead prey caught by the GTs (Sugiura & Yamazaki, 2006; Romero, Souza & Vasconcellos-Neto, 2008; Krimmel & Pearse, 2013).
Resin bugs (Hemiptera: Reduviidae) collect viscous substances (Roepke, 1932; Schuh & Slater, 1995; Gil-Santana, Costa, Forero, & Zeraik, 2003; Forero, Choe, & Weirauch, 2011; Zhang, Weirauch, Zhang, & Forero, 2015) which assist them in prey capture and protect their eggs against predators (Choe & Rust 2007). To date, the morphological base of the ability of these insects to collect and store fluids from a trichome-bearing plant has not been studied in the Neotropics.
Recently we described the natural history and the resin-collecting behavior of the assassin bug Heniartes stali Wygodzinsky 1953 (Hemiptera: Reduviidae: Harpactorinae) in association with Rubus adenotrichos (Schltdl.) (Rosaceae), an Andean wild blackberry whose stems and buds are covered by a densely arranged glandular sticky trichomes. We collected immature and adults of both sexes of H. stali on this plant as they harvested the adhesive GT secretion with the forelegs and stored it on the hindlegs (Avila-Núñez, Naya, Otero, & Alonso-Amelot, 2016). This exudate was an effective tool for prey capture (Avila-Núñez, Naya, Otero, & Alonso-Amelot, 2017). We also found H. stali on Melinis minutiflora grass, which was also covered with a thick layer of GTs. The association of Heniartes bugs and sticky trichome plants is not a rare phenomenon, since other as yet undetermined bug species have been observed in the field on such plants (Zhang & Weirauch, 2011; Zhang et al., 2015). Thus, H. stali is an excellent model for studying this exploitation of plant resource by insects. Our study was focused on three issues: 1. Morphology of the GTs of R. adenotrichos; 2. Chemical composition of the exudate of GT, and 3. Functional morphology of H. stali legs involved in the collection and storing of the exudate from GTs of R. adenotrichos.
Materials and methods
Plant and insect material: Stems of juvenile and mature R. adenotrichos individuals were collected in October 2015 growing in wild thickets along Monterrey road (2 515 m ), near Merida city, Merida State, Venezuela (8º40’43’’ N & 71º6’51’’ W) marked by anthropogenic fragments of shrubs, ferns, grasses and arboreal plants. Rainfall and average annual temperature is 2 560 mm and 13 ºC, respectively, with a bimodal March-May and August-November rain regime (Acevedo & Ataroff, 2012).
For the morpho-functional study, we examined the right legs (fore and hind legs) of six female adults of H. stali collected in La Llanada (2 150 m), near to Merida city, Venezuela (8º35’55’’N & 71º10’56’’ W). Voucher specimens of R. adenotrichos were deposited in the herbarium of the Facultad de Ciencias Ambientales y Forestales (No. 054882 MER) and H. stali in the insect collection of the Laboratorio de Ecología de Insectos (No. ED201 CLEI-ULA) of the Universidad de Los Andes, Mérida, Venezuela.
Morphology of GT of R. adenotrichos: Fresh stem samples from juvenile (N = 6) and mature (N = 6) individuals of R. adenotrichos were examined using a Kyowa SDZ-TR-PL stereo microscope. We also recorded the morphology and distribution of GT in leaves and inflorescences.
The density and length of GTs on the juvenile stems samples were measured. Small pieces of epidermal stripes (0.5 cm2) were prepared macerating sections of fresh juvenile stems (1 cm long) in 10 % KOH solution during 24 h at room temperature (modified from Kiger, 1971). Samples were then washed with distilled water and embedded in a glycerine drop on microscopic slide. The number of GTs per unit area was estimated at 20x magnification by counting the trichomes in randomly selected samples of stems (N = 15) from five juvenile plants (Gravano, Tani, Bennici, & Gucci, 1998). For measurements, GTs (N = 32) from 10 randomly selected epidermal stripes from five juvenile plants were removed using a fine point pincer. The distance from the middle point of the base to the vertex of the head of each GT was measured using ToupView®3.2 Image Software.
For the histological analysis, five stem sections (0.5 cm long) from juvenile plants were fixed in FAA 50 for 48 hours, followed by dehydration in an ethanol series, stored in 100 % ethanol, and embedded in paraffin wax (Paraplast®Plus) (Johansen, 1940). Serial sections (10 μm thick) were obtained on a rotator microtome, stained with astra blue and basic fuchsin (Kraus et al., 1998), and mounted in Canada balsam on glass slides.
Chemical composition of the exudate from R. adenotrichos
Microscopic analysis/Staining: The Prussian blue method (Price & Butler, 1977) for phenolics and the Dragendorff assay for alkaloids (Furr & Mahlberg, 1981) were employed in histochemical tests. Twelve fresh GTs were pulled off with a fine point pincer. Six GTs were soaked with a distilled water drop and, at 1 min intervals, one drop of FeNH4(SO4)2 solution was added, followed by another drop of a K3Fe(CN)6 solution 20 min after. One drop of Dragendorff reagent was added to six GTs. After exposing GTs to these reagents color changes were observed in the light microscope.
Gas Chromatography-Mass Spectrometry (GC-MS): The GT exudate was selectively extracted following the procedure described by Calcagno-Pissarelli, Alonso-Amelot, Mora, Rodríguez, and Avila-Núñez (2010). Removing previously the leaves, fresh sections (30 cm long) of juvenile stems (311 g) were immersed in dichloromethane (10 mL/g fresh weight) in an ultrasound bath, 3 times for 30 s followed by dehydration with anhydrous sodium sulfate, filtered, and evaporated to dryness at reduced pressure a 30 ºC, yielding a crude residue (1.61 g; 0.5 % of fresh weight). This material was flash-chromatographed through silica gel and eluted with a hexane-dichloromethane-ethyl acetate-methanol gradient into four fractions (F1, 0.014 g; F2, 0.149 g; F3, 0.409 g, and F4, 0.770 g). F1 and F2 were pooled and all fractions were taken up in acetone (5 mg/mL) for GC-MS analysis (Hewlett-Packard GC-quadrupole MS Model 5973). We used a Hewlett-Packard HP-MS fused silica column (30 m x 0.25 mm (inner diameter), film thickness, 0.25 µm; injection volume, 1.0 µL. The transfer line temperature was programmed from 100 to 300 ºC (5 ºC/min); source temperature, 230 ºC; quadrupole temperature, 150 ºC. The carrier gas was helium, adjusted to a linear velocity of 34 cm/s; scan range, 40-700 amu; 3.9 scans/s. The identity of components was established by matching against Mass Spectra Wiley library (6th edition) and those reported in the literature (Adams, 2007).
Morphology of H. stali legs: Our previous observations indicated that forelegs and hind legs were used by H. stali for collecting and storing the exudate from GT (Avila-Núñez et al., 2016). For LM, legs (N= 3) were excised, macerated in 10 % KOH for 48 h and rinsed with 70 % ethanol following the procedure of Zhang & Weirauch (2011). Subsequently, they were immersed in a glycerol drop on a microscope slide. For scanning electron microscopy (SEM), excised legs (N = 3) were immersed in dichloromethane for 30 s to remove the sticky exudate and dehydrated in a series of ethanol dilutions (30 - 100% in 10 % steps) and then one step in 100 % acetone. The samples were mounted on metallic stubs and using a Hitachi HUS-5GB vacuum evaporator, the drying was completed for 5 s. Finally, the samples were sputter-coated with a 200 nm-thick layer of gold for 300 s, at 8 mA current using Ion Coater 1B-2 (Hitachi High-Technologies Corporation, Japan). Micrographs were obtained in a Hitachi S-2500 scanning electron microscope (Hitachi High-Technologies Corporation, Japan).
Leg and GT morphology was studied with macrograph and micrograph images obtained using T-10 (Sony Cybershot) and FMA050 (AmScope Co) digital cameras, respectively, both for leg´s and GT morphology. Insect structures were termed as in Forero et al. (2011).
Results
GT of R. adenotrichos: SM showed the presence of trichomes on stems, petioles, young leaves and inflorescences, while on mature leaves trichomes were located primarily on veins and margins of the abaxial side (Fig. 1A). We observed two trichome types: non-glandular trichomes (NGTs) and GTs (Fig. 1B, Fig. 1C). The NGTs were unbranched, tapered, having a translucent appearance. GTs were unbranched, multicellulary stalked, capitate, occurring with a mean of 44.13 GT per cm2 of stem sections (Standard Deviation, SD = 9.58) and a mean of 1.83 mm (SD = 0.99) in length. They had a characteristic dark red color although they were less pigmented in those plant parts less exposed to sunlight (Fig.1B). A yellowish secretion was released by the calyx-shaped head as individual droplets (Fig. 1D) which eventually collapsed and smeared the GT stalk (Fig. 1C). Stem surfaces turned to gummy traps for wandering insects as recognized in our field observations (Fig. 1B).
LM images revealed that GT stalk cells were arranged in multiple rows, while the cells of the glandular head containing red-stained vacuoles were radially organized (Fig. 1E, Fig. 1F). The outer cell walls of the latter were greatly thickened and heavily cutinized. Additionally to trichomes, we also could distinguish the typical tissues of a dicotyledonous stem (Fig. 1E).
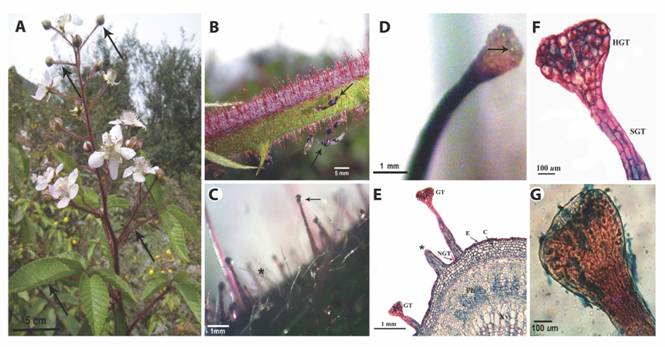
Fig. 1 The plant surface of R. adenotrichos. A. Aspect of the whole plant showing parts bearing trichomes (arrows). B. Trichomes perpendicularly aligned to the stem surface. Some trapped insects can be seen on these sticky structures (arrows). C. Stereoscopic view of glandular trichomes (arrow) and non-glandular, filiform trichomes (asterisk) in a stem surface. Arrow points exudate at the tip of the trichome. D. A calyx-shaped head of a glandular trichome showing the secretion of tiny drops (arrow) of the exudate. E. Light microscopy micrograph (LM) of a stem cross-section. Glandular trichome (GT), Non-glandular trichome (NGT), Epidermal (E), chlorenchyma (C), phloema (Ph) and xylema (Xy) tissues can be viewed. Asterisk indicates a ruptured GT. F. Detail of GT obtained with LM. Glandular head (HGT) and multicellular stalk (SGT) G. LM image showing residual exudate in the head of glandular trichome upon staining with blue.
Chemical composition of the exudate of GT from R. adenotrichos: The intense blue coloration upon exposure of GT to the Prussian blue reagent suggested the presence of phenolic compounds in the trichome secretion (Fig. 1G), whereas alkaloids were absent (Dragendorff reagent).
Twelve major compounds, chiefly terpenoids were identified by GC-MS analyses of the exudate of R. adenotrichos (Table 1, Fig. 2). The waxes nanocosane and hentriacontane were the most abundant constituents in fraction F2, in addition to heptacosane and nanocosane in F3 and F4. These cuticular hydrocarbons were probably co-extracted with dichloromethane, i.e., artefacts which do not belong to the exudate compounds. F3 predominantly contained lupeol, and a few other sesquiterpenes. A greater molecular diversity was identified in F4, including blue-reactive phenolic compound eugenol and hydroxy chromene (Table 1).
TABLE 1 Major compounds identified by GC-MS from column chromatography fractions of the glandular exudate of R. adenotrichos (see Fig. 2 for further information)
Fraction | Nº | Compound | Class | Tr (min) | % ** |
2 | 1 | Heneicosane | h | 27.506 | 0.5 |
2 | Pentacosane | h | 30.715 | 0.8 | |
3 | Heptacosane | h | 33.695 | 1.3 | |
4 | Nonacosane | h | 36.476 | 3.9 | |
5 | Hentriacontane | h | 39.074 | 2.3 | |
3 | 1 | Caryophyllene oxide | s | 13.990 | 0.5 |
2 | Spathulenol | s | 14.884 | 1.3 | |
3 | Germacra-4-(15),5,10,(14)-trien-1α-ol | s | 16.144 | 1.4 | |
4 | Heptacosane | h | 33.683 | 2.8 | |
5 | Nonacosane | h | 36.476 | 1.4 | |
6 | Lupeol | t | 42.585 | 12.6 | |
4 | 1 | Eugenol | php | 8.927 | 4.17 |
2 | Caryophyllene oxide | s | 13.975 | 2.0 | |
3 | Spathulenol | s | 14.880 | 0.4 | |
4 | trans-Caryophyllene | s | 16.150 | 0.6 | |
5 | Germacra-4-(15),5,10,(14)-trien-1α-ol | s | 16.140 | 2.6 | |
6 | Heptacosane | h | 33.695 | 5.5 | |
7 | Nonacosane | h | 36.487 | 2.8 | |
8 | 2-(3,4-dimethoxyphenyl)-7-hydroxi-3-Methoxy-4H-chromen | c | 37.530 | 7.6 | |
9 | Lupeol | t | 42.591 | 20.4 | |
Total | 74.87 |
Tr = retention time in minutes; ** percentage relative to the total of identified compounds; h = long-chain linear hydrocarbons, s = sesquiterpene, t = triterpene, php = phenylpropene, c = chromen.
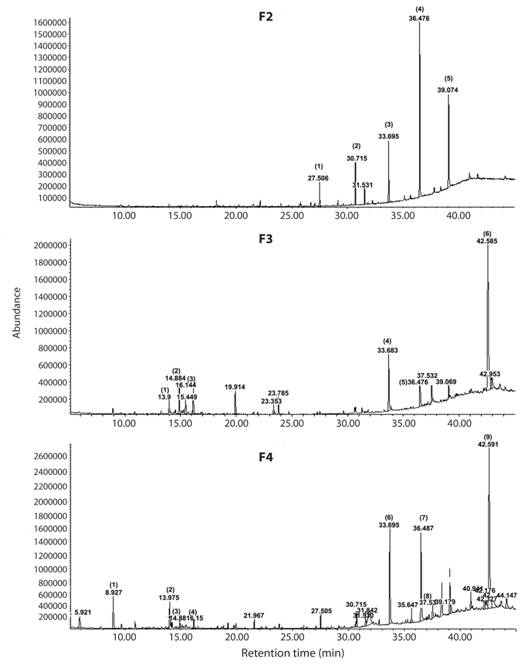
Fig. 2 Spectra obtained from GC-MS analysis column chromatography, demonstrating F2, F3, F4 of the plant extract prepared from R. adenotrichos. Numbers at peaks correspond to their retention times. Numbers in parentheses indicate the identified compound (for details see table 1). The peaks were identified according to the Mass Spectra library (6th edition) and Adams (2007).
Leg morphology of H. stali: The apex of the protibia was directly involved in the collection of resin from GT heads (Fig. 3A). We could identify two areas with different vestiture on the ventroapical surface (Fig. 3B, Fig. 3C). In the distal area the setation was less dense, with short setae and scattered long setae. The proximal area was covered with a brush-like structure with very dense vestiture composed by long, wide and concave setae. A short row of straight setae protruded from the center of the upper edge.
Hind legs: The harvested GT exudate was stored on the metatibiae (Fig. 3D) whose most conspicuous characteristic were long, brush-like and pointed setae (Fig. 3E) either erect or procumbent, and some of them longer than the tibia’s width. Although setae were observed on the entire metatibia surface, they were more abundant on middle section. Another remarkable feature was the abundance of small ovoid protuberances with irregular-shaped openings distributed on the entire metatibial cuticle (Fig. 3E, Fig. 3F).
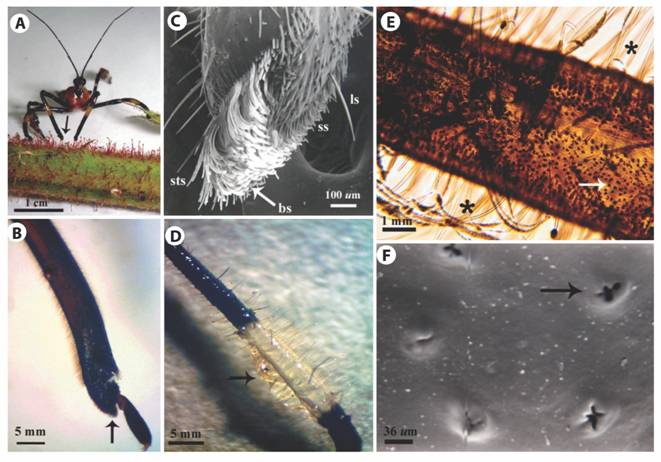
Fig. 3 Morphology of H. stali legs. A. A female on a stem of R. adenotrichos while gathering the sticky exudate from the trichome heads employing the foreleg end (arrow). B. LM image of the lateral view of a foreleg showing a brush-like structure in the protibia tip (arrow). C. SEM image of protibia. Short setae (ss), long setae (ls), brush-like structure (bs), short straight setae (sts). D. Metatibia covered with secretion of the plant trichomes adhering to the bulk of setae (arrow). E. LM image of a metatibia showing long setae (asterisks) and high number of pores dispersed over the cuticle (arrow). F. SEM image of ornamented pore in the cuticle of metatibia (arrow).
Discussion
The presence and abundance of GTs secreting a sticky fluid was a relevant attribute of R. adenotrichos aerial parts. These GTs showed typical multicellular structures in the base, long stalk and glandular head (Werker, 2000). However, the sticky fluid did not accumulate within a subcuticular space in the glandular tip as in other plants bearing GT with a similar basic morphology, nor was this fluid released by the rupture of the cuticle (Gregory, Ave, Bouthyette, & Tingey, 1986; Pichersky & Gershenzon, 2002; Peiffer et al., 2009). In R adenotrichos, the GT heads appeared as secreting organs which exported the sticky fluid possibly through cuticular pores (Wagner et al., 2004; Sousa, 2016). Once on the outside, the secretion was dispersed throughout the GT stalk.
Although the origin, cell development, and properties of prickles and GT in species of Rubus and other Rosaceae have been investigated (Kellogg, Branaman, Jones, Little, & Swanson, 2011; Gallenmüller, Feus, Fiedler, & Speck, 2015), several studies describe the morphology and anatomy of their GTs. Sulborska and Weryszko-Chmielewska (2014) described the secretory structures in the flowers of Rosa rugosa Thunb. as GTs having the features of colleters, with stalk and glandular head whose cells were radially arranged in a globular contour. While studied the raspberries Rubus phoenicolasius Maxim. and R. odoratus L. Pohl (2009) and Roshchina (2014) characterized these GTs as multi-cellular glands constituted by a stalk and glandular head with morphology and anatomy very similar to R. adenotrichos’s GTs
Our chemical analysis of the R. adenotrichos GT exudate let assume a lipophilic mix mainly of terpenes, phenolic compounds and others. Many of these compounds are bioactive and/or physiologically important in GT-bearing plants (Schilmiller, Last, & Pichersky, 2008; Schnetzler, Teixeira & Ribeiro-Marinho, 2017; Ribeiro-Marinho, Poletti, Gobbo-Neto & Pádua, 2018). Among Rosaceae, the GT of Rosa rugosa secrete a viscous fluid in which the sesquiterpenes rugosal and others related to carotane, are predominant constituents (Hashidoko, Satushi, & Junya, 1992; Hashidoko, Endoh, Kudo, & Tahara, 2001). In Rubus, studies on the chemical constituents have been focused on leaves and fruits from non-glandular species. Numerous diterpenes and triterpenes have been isolated (Patel, Rojas-Vera, Dacke, 2004), some of them with taxonomic significance such as rubusoside and suavioside (Seto, Tanaka, & Tanaka, 1984). Also it is known Rubus contains a number of phenolic compounds, the prevailing being anthocyanins and ellagitannins (Mullen et al., 2002; Maatta-Riihinen, Kamal-Eldin, & Torronen, 2004).
In our study, lupeol, a common triterpene of higher plants, was the major compound identified in the R. adenotrichos exudate, in consonance with the GT sticky secretion of certain insect-trapping plants (Simoneit, Medeiros, & Wollenweber, 2008; Frenzke et al., 2016). Lupeol biotransformation, mechanism of action, and biological activities are on record (Gallo & Sarachine, 2009).
Triterpenes and phenolics present in the exudate of R. adenotrichos could explain the adhesive properties. It is known natural adhesives do not consist of one compound only but are chemical mixtures (Betz, 2010). Triterpenes, as pure natural products, are crystalline solids, but as a mixture they form sticky syrup (Simoneit et al., 2008). Additionally, polyphenolics are one class of chemical compounds which are frequently found in bioadhesives (Rischka et al., 2010).
During our analysis of the dichloromethane-soluble fraction, although stem sections were briefly immersed in the solvent to dissolve the exudate, this wash might have included more compounds than only those of the exudate. Chances are, however, that these are cuticular substances and probably not cell vacuole substances from stem cuticle or parenchyma. Additionally, some peaks in the GC trace escaped mass spectral characterization. Compound richness could be enhanced by more exhaustive extraction procedures using other solvents. We are currently expanding our studies in this regard.
The morphology of H. stali´s female legs suggests the evolution of morpho-functional traits to gather and store viscous exudates from sticky plants. Two areas of vestiture and modified setae on fore and hind legs support this contention. The brush-like structure on the protibia tip of H. stali suggests an adaptation for collecting efficiently the tiny drops on the glandular head of trichomes. Bugs extended their forelegs to browse the GTs heads repeatedly to load up the hairy tips of protibia with resin. An expansion of the apex of the protibia area has also been identified in the resin bug Heniartes flavicans Fabricius 1798 (Forero et al., 2011), another harvesting species of GT sticky exudate (Zhang et al., 2015). However, apical setae in H. flavicans forelegs are more discrete than the brushy setation of H stali.
The resin-storing area in the hind legs which was characterized by long and thin setae was a prominent trait in H. stali. Setae appeared to operate as a multifunctional brush to collect and store temporarily the exudate being transferred from the forelegs after harvesting from GTs. In turn, the stored resin was reclaimed by the forelegs as required for prey trapping (Avila-Nuñez et al., 2016). By contrast, the vestiture on the anterior surface of H. flavican´s metatibiae showed only sparse and relatively short setae (Forero et al., 2011).
The abundant ovoid protuberances on the cuticle of H. stali metatibiae were another interesting finding. Catalá and Scholfield (1994) recorded similar structures in antennal sensilla of hematophagous bug Rhodnius (Reduviidae: Triatominae) as highly ornamented pores with 5-6 inner extensions. Although pores in the metatibiae have been reported in other Apiomerini species (Forero et al. 2011), ours is the first record of ornamented pores in this group. The function of these openings in H. stali is still uncertain. According to Weirauch (2008), who identified similar pores in Triatominae as outlets of dermal glands, these structures in Apiomerini might produce secretions to prevent the hardening of resin stored in the hairy structures and maintain the insect ability to manipulate it (Avila-Nuñez et al. 2016). This hypothesis has also been proposed for Apiomerus females that store plant resins on their abdominal surface where gland structures have been observed (Forero et al., 2011), but this is as yet untested.
Our results suggest that the structural modifications in the fore and hind legs constitute morphological traits to exploit plants with resinous trichomes. These findings contribute to the considerations of the sticky plant trichome usage in assassin bugs, which evolved as an exogenous source of adhesive fluids in sticky trap strategy (Zhang et al. 2015). These results have also revealed new directions toward a more profound study of this fascinating interaction. Our ongoing research aims to associate the antioxidant properties of the exudate and the stability of its sticky properties upon air contact after harvesting by H. stali and the purported role of the insect’s secretions to prevent the collected exudate hardening.