Phyllomedusa (Anura: Hylidae) is a frog genus, composed by 32 species and presents unique characteristics that differentiate it from other frogs, like green color in the dorsal body region, pupils located in the vertical fends, slow locomotion and arboreal life style (Caramaschi & Cruz, 2002; Frost, 2017). This genus is recognized for possessing four phenotypic groups where most species are alocated: P. burmeisteri group, P. perinesos group, P. tarsius group and P. hypochondrialis group. This last one receives more attention, essentially because it exhibits a great diversity of described species (Faivovich et al., 2010). The species P. azurea was revalidated and included in the P. hypochondriallis group (Caramaschi, 2006), despite a study based on an integrative approach highlighted the need for a revision on the diagnostic features used for the identification of this species in this group (Bruschi, Busin, Toledo, Vasconcellos, Strussmann, Weber, Lima, Lima, & Reco-Pimentel, 2013). Morphologically, the male adult has a medium size of 43 mm and 42.3-44 mm for the female, green skin color in their dorsal region, white in the ventral and lateral regions besides an orange colour with black strips in internal parts of anterior and posterior members. Concerning its geographic range, P. azurea inhabits open formations in South America and has been recorded in Paraguay, east of Bolivia, north of Argentina, and in the Brazilian Pantanal and Cerrado with its activity restricted to months with greater humidity and precipitation levels (Freitas, Carvalho, Faria, Batista, Batista, Coelho, & Bocchiglier, 2008; Prado, Borges, Silva, Tognolo, & Rossa-Feres, 2010; Lucas, Fortes, & Garcia, 2010; AmphibiaWeb, 2018).
The amphibian cutaneous apparatus plays a crucial role in several homeostatic processes, as respiration, water and solute transport, osmotic regulation, body temperature control and evolved to defense against predators and pathogenic microorganisms. A broad range of biologically active compounds have been characterized from these secretions including alkaloids, steroids, bioorganic amines, peptides and proteins (Clarke, 1997; Xi, Li, Chen, & Kwok, 2015). Particularly for those animals, antimicrobial peptides (“AMPs”) produced by epithelial cells and secreted by granular glands located in the skin surface are predominant and essentially has as function to prevent or avoid the colonization of microbial pathogens as part of their innate immune system (Rinaldi, 2002; Conlon, 2011), complementing this role also performed by their skin associated microbiota (Assis, Barreto, & Navas, 2017). Several AMPs isolated from these animals acts primarily through binding to negatively-charged molecules and/or other membrane lipids, disturbing the bacterial envelope structure what leads to cell lysis (Rollins-Smith, Reinert, O’Leary, Houston, & Woodhams, 2005). Members from the subfamily Phyllomedusinae stands out about production of AMPs, with more than 80 known AMPs, which are divided in six main classes and similar in relation to the primary structure and action spectrum: dermaseptins, phyloseptins, plasticins, dermatoxins, phylloxins and hypoxins. Regarding P. azurea, more than 40 bioactive peptides have been isolated to date with attention to phylloseptins with antibacterial, antiprotozoal activities and low-hemolytic action (Leite et al., 2005; Conceição et al., 2006; Kückelhaus, Leite, Muniz-Junqueira, Sampaio, Bloch Jr., & Tosta, 2009; Calderon, Silva, Ciancaglini, & Stábeli, 2011).
The increasing threat of multi-resistant bacterial infections for human health poses a major challenge in the discovery of new drugs which has considerably declined in the last decades. With this scenario, the intensive search for effective molecules against these pathogens is indispensable (Wright, 2016). The biological and pharmacological properties of amphibious skin secretion attract more attention from the antimicrobial drug discovery research, since the isolated AMPs show effectiveness in the bacterial infection treatment, precisely due to their action on membrane what hampers the development of resistance by the target microorganisms, and that do not cause any deleterious consequence on the mammalian cells (Cardoso et al., 2014; Ladram & Nicolas, 2016). Therefore, amphibian skin peptides must be considered as a source for alternative antimicrobial drugs once a plenitude of molecules remains to be exploited, especially for the genus Phyllomedusa (Conlon & Sonnevend, 2011; Neiva, Vargas, Conceição, Rádis-Baptista, Assakura, Jared, & Hayashi, 2013). Up to the present, the last work involving the evaluation of bioactivity of peptides extracted specifically from P. azurea was from Kückelhaus et al. (2009) where the authors confirmed activity against Plasmodium falciparum and Leishmania amazonensis of a synthetic amidated-phylloseptin-1 with low toxicity against human macrophages, opening up this molecule as a candidate for the treatment of these tropical protozoal diseases. In this context, our goal was to investigate the antimicrobial activity of the skin secretion of P. azurea from Brazilian Cerrado by determination of the minimum inhibitory concentration (MIC) against different reference bacterial strains and to evaluate of its capacity in affecting the bacterial cellular envelop permeability.
Materials and methods
Animals specimens: Amphibians used in the present study were collected in different points of central Cerrado in rainy seasons and given in by the Toxinology Laboratory (Department of Physiological Sciences, University of Brasília, Brasília, DF) under the “Chico Mendes Institute for Biodiversity Conservation” (ICMBio) license number 41555-1. A total of five specimens were kept in captivity between 7 and 10 days in a terrarium under controlled conditions of temperature and humidity at 28 °C and ٦5 %, respectively.
Skin secretion sampling and preparation: The crude extract was obtained by mild electrical stimulation (approximately 9 V and 500 mA) on the dorsal skin of the animals. The recovered material was washed and homogenized from the skin with ultrapure water, sterilized by filtration and, immediately, submitted to lyophilizing. Lyophilizate was then stored at 4 °C for further experiments. For antimicrobial assays, a total of 1.0 mg of lyophilizate were weighted and diluted in 1.0 mL of ultrapure water under aseptic conditions, to achieve an initial concentration of 1.0 mg/mL. The experimental protocol was approved by the Ethics Committee on Animal Use at the Pontifical Catholic University of Goiás (PUC-GO, opinion number 0008/1). Additionally, every effort was made to avoid unnecessary stress and pain to the animals.
Bacterial strains and culture conditions: Control ATCC (American Type Culture Collection) strains were provided by the Environmental Microbiology and Biotechnology Laboratory from the Institute of Tropical Pathology and Health Public of the Federal University of Goiás. It was used a total of four indicator strains: two Gram-positive, Staphylococcus aureus ATCC 25923 and Bacilus subtilis ATCC 6633; and two Gram-negative, Escherichia coli ATCC 25922 and Pseudomonas aeruginosa ATCC 27853. Brain Heart Infusion (BHI, Difco™) medium was used for bacterial reactivation and culture for experiments, with the incubation at 37 °C for 24 h.
Determination of antimicrobial activity by the skin secretion: Aiming to evaluate the antibacterial activity of the skin secretion recovered from P. azurea, it was carried out the MIC determination of crude extract. The broth microdilution test was executed in 96-well microtiter plates to stablish the bacterial susceptibility to different concentrations of the crude extract in accordance with the Clinical and Laboratory Standards Institute (CLSI) instructions (CLSI, 2015). Firstly, the indicator bacterial strains cultivated in BHI were diluted sequentially in saline solution at 0.85 % (m/v) to reach the proper 107 UFC/mL cell concentration. Next, serial dilutions of the crude extract (500-0.49 μg/mL) in the twice-concentrated Mueller-Hinton (MH) broth (HiMedia, India) were prepared in the microtiter plates. Posteriorly, a volume of 10 μL of the diluted bacterial suspensions at the wells. Three controls were prepared for the experiment: (1) MH broth control contamination, with only the MH broth; (2) microbial growth control, with the microbial dilution suspended in the MH broth; (3) crude extract control, with the skin secretion extract homogenized in the MH broth. Finally, the material was incubated at 37 °C for 18-24 h.
The confirmation of the bacterial growth inhibition by the crude extract was accomplished with the addition of a 3-(4,5-dimethylthiazol-2-yl)-2,5- diphenyltetrazolium bromide (MTT, Merck, Kenilworth, NJ, EUA) solution at 0.5 % (m/v) in the wells to check cell viability. After the incubation with this reagent at 37 °C for 35 min, the plates were carried to a spectrophotometer (TP Reader Basic, Thermo Plate) and the absorbance was readed at 450 nm. Inhibition was calculated as percentage of growth when compared to the growth in the absence of any inhibitor. The experiment was done in triplicate. The results were analyzed by comparing two groups applying the t-test with a significance level of 0.05 (Microsoft Excel 2016). The minimum bactericidal concentration (MBC), the one which inhibits 99,9 % of bacterial growth (Isenberg, 1992), was confirmed through plating of 10 μL-aliquots from the wells on BHI Agar before adding MTT and inspecting the presence or absence of bacterial growth after incubation at 37 °C for 18 h.
Crystal violet assay: Considering that most of AMPs isolated from Phyllomedusinae frogs act at the plasma membrane (Wan et al., 2015), the crystal violet assay was chosen as an initial screening test to assess any level of disturbance in the bacterial cell envelope permeability caused by the cutaneous secretion components (Devi, Nisha, Sakthivel, & Pandian, 2010). The standard strain of S. aureus ATCC 25923 was selected as indicator for this test because the probable greater susceptibility of Gram-positive bacteria to the AMPs present in the crude extract. Thus, a suspension of the bacterial strain was prepared in BHI broth and subsequently transferred to a phosphate buffer solution (PBS) 1x (pH 7.4) with the turbidity adjusted to the MacFarland standard No. 0.5. The suspension was centrifuged at 4.500 g during 5 min at 4 °C, with the supernatant discarded. Then, it was added 1.5 mL of PBS to the pellet, with a new centrifugation step. This washing procedure was repeated once more. Afterwards, a total volume of 250 μL of the bacterial suspension was added to eppendorfs microtubes. At each one of these microtubes was also added a volume of 250 μL of the following solutions: an equivalent to the MIC of the secretion from P. azurea, twice concentrated and four times concentrated MIC, EDTA (Synth, Diadema, SP, Brazil) at 0.25 M (positive control) and PBS 1x (negative control).
The tubes were incubated at 37 °C for 30 min. Next, a new centrifugation was carried out at ٩.500 g for 5 min. The supernatant was discarded, and it was added 500 μL of PBS supplemented with crystal violet (Synth) at 10 μg/mL. Subsequently, the solution was incubated at 37 °C for 10 min and centrifuged at 13.400 g for 15 min. A volume of 100 μL of the supernatant was added in a microtiter plate well and the absorbance was readed at 595 nm. The percentage of crystal violet cell uptake was calculated by the formula: [(Sample absorbance/Crystal violet solution absorbance) X 100)]. All the tests were run in triplicate.
Results
Determination of antimicrobial activity by the skin secretion: Overall, the amphibian skin secretion presented inhibitory activity against all indicator strains (Figure 1). In relation to S. aureus ATCC 25923 and E. coli ATCC 25922, no bacterial growth was observed for the following secretion concentrations: 500 μg/mL, 250 μg/mL, 125 μg/mL, 62.5 μg/mL and 31.25 μg/mL. Regarding to the B. subtilis ATCC 6633, the secretion inhibited its growth at concentrations ranging from 500 μg/mL to 125 μg/mL. It was verified a higher degree of inhibition (growth > 10 %) of P. aeruginosaATCC 27853 only at 500 μg/mL and 250 μg/mL dilutions. Thus, it was possible to determine the MIC for each tested strain: 31.25 μg/mL for S. aureus ATCC 25923 and E. coli ATCC 25922, 125 μg/mL for B. cereus ATCC 6633 and 250 μg/mL for P. aeruginosa ATCC 27853. All the results were statistically significant (p < 0.05) ( Figure 1 A, Figure 1B, Figure 1C and Figure 1D). The MBC values were 31.25 μg/mL, 62.5 μg/mL, 250 μg/mL and 500 μg/mL for S. aureus ATCC 25923, E. coli ATCC 25922, P. aeruginosa ATCC 27853 and B. cereus ATCC 6633, respectively.
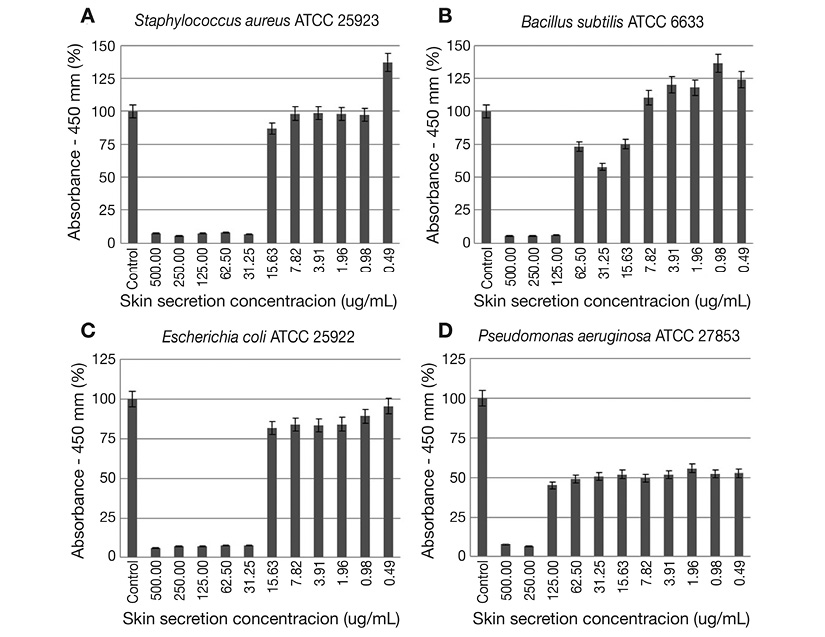
Figure 1 Microdilution broth assay results with: A. S. aureus ATCC 25923; B. B. subtilis ATCC 6633; C. E. coli ATCC 25922; and D. P. aeruginosa ATCC 27853 as test organisms. Represented data were calculated from the average absorbance values. The control consists in the bacterial growth in the absence of treatment with the skin secretion.
Crystal violet assay: The skin secretion exhibited activity on the cell envelope permeability of the S. aureus ATCC 25923, with this efficacy changing according to the evaluated concentration: 31.25 μg/mL (MIC), 62.5 μg/mL (MICx2) and 125 μg/mL (MICx4). The major percentage of crystal violet pickup was at 125 μg/mL, in other words, this concentration caused the greatest change in the cell envelope permeability of S. aureus ATCC 25923 compared to the other concentration values. In the absence of exposure to the skin secretion, the crystal violet absorption was 53.1 %. The treatment with EDTA resulted in an increasing of the absorption (97.7 %), when compared with the skin secretion treatment. The crystal violet absorption by S. aureus in presence of MIC was 72.3 %, rising progressively, 73.8 % for MICx2 and 83.8 % for MICx4 (Figure 2).
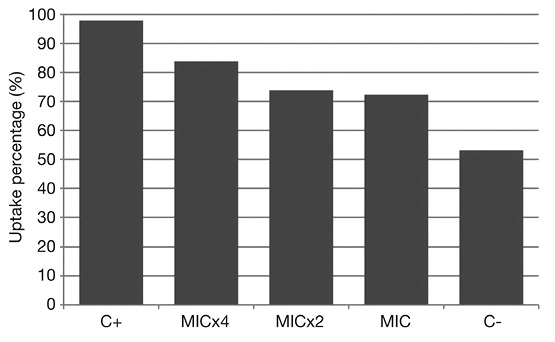
Figure 2 Crystal violet assay results with S. aureus ATCC 25923 as indicator strain. C-: negative control (bacterial cells treated only with PBS); C+: positive control (bacterial cells treated with EDTA 0.25 M); MIC, MICx2, MICx4: skin secretion dilutions, respectively, 31.25 μg/mL, 62.5 μg/mL and 125 μg/mL.
Discussion
The skin secretion of frogs from the family Phyllomedusinae consists in an abounding source of AMPs, particularly from the dermaseptin and phylloseptin families (Brand et al., 2013). According to Calderon et al. (2011), the compounds from these two families encountered in the skin secretion of P. azurea have lytic activity against Gram-positive bacteria (Corynebacterium glutamicum, Enterococcus faecalis, Klebsiella pneumoniae, Micrococcus luteus, Nocardia spp., Nocardia brasiliensis, S. aureus, Streptococcus agalactiae, Streptococcus dysgalactiae), Gram-negative bacteria (Acholeplasma laidlawii, Acinetobacter calcoaceticus, E. coli, Neisseria gonorrhoeae, P. aeruginosa), fungus (Candida albicans, C. tropicalis, C. guilliermondiie) and even protozoa (L. major, L. mexicana, L. amazonensis, L. chagasi, P. falciparum, Trypanosoma cruzi). Except for the genomic/proteomics guided studies from the same research group (Thompson, Bjourson, Orr, Shaw, & McClean, 2007a; Thompson, Bjourson, Orr, Shaw, & McClean, 2007b), there is a scarcity of literature focusing on the antimicrobial activity of peptides and compounds of other nature in P. azureaskin secretion, specifically against bacterial pathogens.
Conlon et al. (2007) evaluated the antimicrobial potential of the skin secretion of Phyllomedusa lemus, which was tested against S. aureus, E. coli and the fungus Batrachochytrium dendrobatidis, responsible for chytridiomycosis, a common amphibian skin infection. It was found peptides belonging to these two classes of AMPs and they distinguished from each other regarding to the antimicrobial activity spectrum. Dermaseptin-L1 inhibited the growth of E. coli and B. dendrobatidis and did not affect the S. aureus strain. On the other hand, phylloseptin-L1 presented activity against S. aureus and B. dendrobatidis and not against E. coli. In our study, the MIC values for S. aureus and E. coli strains were equal, and it is possible to infer the presence of AMPs from at least these two families, dermaseptins and phylloseptins, probably in different proportions what could explain the broad activity of the crude extract. This is expected once various peptides may have special targets in the interaction with the complex cell wall and membrane of microorganisms and provides an extra layer to the natural defense role of these molecules to the animal (Shi et al., 2016).
In a study focused on the structural and functional characterization of phyllospetin-PBa from P. baltea, Wan et al. (2015) found a higher MIC value for E. coli (128 mg/L) than for S. aureus (8.0 mg/mL), and the authors attribute this weaker antibacterial activity against Gram-negative to the presence of a single bilayer lipid membrane in Gram-positive bacteria and rich acidic membrane that reduces the charge on their surfaces. Our study evidenced not only this commonly found susceptibility among Gram-positive strains for these antimicrobial compounds in amphibian cutaneous secretion, but also in Gram-negative indicator bacteria, with the same MIC value observed for S. aureus and E. coli as mentioned above. An elevation of MBC values may indicate that the bioactive molecules are in a low concentration in the crude extract, and the need to purify them to improve the observable antibacterial profile. Nevertheless, it was observed that the broad activity spectrum is still maintained for S. aureus and E. coli. Indeed, the crude extract exhibited MIC of 125 μg/mL in preliminary microdilution tests carried out with a hospital methicillin-resistant S. aureus (MRSA) strain (data not shown) and furthers tests are being conducted with other resistant Gram-positive cocci strains.
For Bacillus subtilis ATCC 6633 strain, it was verified (Figure 1D) that the estimated growth in almost half of evaluated concentrations was superior than that found on positive control. This is probably a result of the production of endospores by this genus, which may have germinated during incubation and contributed to the increasing of observed spectrophotometric values. It is worth to point out the growth inhibition of P. aeruginosa ATCC 27853 around 50 % between the concentration values of 62.5 μg/mL and 0.49 μg/mL, even with the high MBC value (500 μg/mL). Possible explanations for this result are: (i) the onset of tolerance or persistence development in microbial cells, what could have not been clear due to the presence of various compounds in the frog skin secretion; (ii) a cellular accumulation of these AMPs and other possible bioactive compounds or a delay in its elimination or turnover in this Gram-negative strain leading to an extension of the inhibitory effect.
With the crystal violet assay, it was evidenced the capacity of the frog skin secretion in changing the plasma cell membrane integrity of the S. aureus ATCC 25923. From the tested secretion dilution, 31.25 μg/mL (MIC), it was detected a dose dependent increase in the crystal violet absorption by the bacterial cell. Despite their diversity, most AMPs act directly against microbes by membrane disruption and pore formation, causing death by osmotic imbalance and/or efflux of ions and nutrients (Epand & Epand, 2010; Peters, Shirtliff, & Jabra-Rizk, 2010). For frog skin AMPs, the Shai-Matsuzaki-Huang model postulates that these peptides first bind to the membrane surface up to a critical concentration and then pass through the membrane’s interior, which is an interesting mechanism of action since theoretically prevents the immediate development of resistance to the peptide (Zairi, Tangy, Bouassida, & Hani 2009). This model agrees with the present results in the crystal violet assay since the dye absorption was directly proportional with the increase of the secretion concentration. In other words, there was more peptide compounds to act on the cell envelope along with a feasible increased absorption of them, resulting in alteration of membrane permeability. Considering the interest in the action in this cell target, the crystal violet assay is also useful not merely to have a first glimpse of the action on membrane but as well to assess in parallel with determination of MIC if this antibacterial activity endure as the purification and characterization of active fractions is carried out, as previously planned in our experimental design in case we have more cutaneous secretion available or when more skin secretion is obtained.
Raja et al. (2013) characterized the structure, antimicrobial activity and mechanism of action of five new phylloseptins from P. sauvagii. Widely, the authors observed a higher activity against Gram-positive strains in comparison with the Gram-negative ones, what was not exactly found in the microdilution tests of the present our study. Additionally, it was evaluated a direct action of phylloseptins against the cytoplasmic membrane of S. aureus and E. coli strains with a different assay, with confirmation by differential scanning calorimetry. Recently, Gao et al. (2017) isolated and synthesized analogs of a medusin from P. tarsuis culminating in an enhancement of its antimicrobial activity expressed by the low MIC values against S. aureus and MRSA besides the analogs inhibited considerably the S. aureus biofilm when compared with the natural peptide. What is interesting about this work is that the authors pointed out that even with the original molecule, alterations are valid not only to increase its bioactivity without affect the low cytotoxicity as also to its stability, beyond being a peptide from a recently-characterized AMP family derived from Phyllomedusa genus.
One major obstacle for the development of the study was the insufficient quantity of the lyophilized secretion due to climate change that affected the sampling process. Once this problem is totally solved, the future natural steps is: determination of MIC for other microbial indicator strains, including fungi and focusing on multi-drug resistant strains; hemolytic assays which are presently in the standardization phase; and, finally, purification and structural characterization of bioactive peptides from the skin secretion followed by antimicrobial and cytotoxic tests with the purified molecules.
In conclusion, the P. azurea skin secretion exhibited antibacterial activity against Gram-positive and Gram-negative strains in microdilution plate tests, with S. aureus ATCC 25923 and E. coli ATCC 25922 (MIC = 31.25 μg/mL) being the indicator strains with the most inhibited growth under the frog secretion action. Furthermore, the secretion increased the crystal violet uptake by staphylococcal treated cells suggesting the cell envelope as the target of the present bioactive compounds. The results confirm the presence of antimicrobial molecules, probably from peptide nature, in the P. azureaskin secretion with a broad-spectrum action. This reinforces the view of this amphibian species as a profitable reservoir of bioactive compounds, highlighting the need to sample more specimens to expand the current, and still restricted, knowledge about its skin secretome.
Ethical statement: authors declare that they all agree with this publication and made significant contributions; that there is no conflict of interest of any kind; and that we followed all pertinent ethical and legal procedures and requirements. A signed document has been filed in the journal archives.