The poor dispersal capabilities of diatoms represent a strong constraint to their spatial distribution along the longitudinal dimension of rivers (Kristiansen, 1996). Given the unidirectional nature of rivers, sessile microorganisms - such as diatoms - tend to be continuously dislodged from upstream to downstream sections of the river (Liu, Soininen, Han, & Declerck, 2013). In order to offset individual losses and maintain viable populations in upstream sections, diatoms have a wide variety of strategies, such as increasing the number of generations per year, developing attachment mechanisms, and growing on animals which can carry them to upstream sections (Peterson & Stevenson, 1989; Kristiansen, 1996; Tiffany, 2011). One such animal dispersal vector could be the turtle, for turtle carapaces provide a suitable substrate for diatoms, while turtles themselves travel long distances in the upstream direction (Fachín-Terán, Vogt, & Thorbjarnarson, 2006; Souza, Raizer, Da Costa, & Martins, 2008; Frick & Pfaller, 2013; Ersanli & Gönulol, 2015).
While the study of epizoic diatoms growing on the carapaces of marine turtles has been advanced considerably in recent years (Frick & Pfaller, 2013; Majewska, Santoro, Bolaños, Chaves, & De Stefano, 2015), such studies for freshwater turtles have remained scarce, and even more so in the Neotropical region, where epizoic diatoms in aquatic ecosystems have only been studied by Wetzel, van de Vijuer, Cox, and Ector (2010); Wetzel, van de Vijuer, Cox, Bicudo, and Ector (2012). The Wetzel studies described two new species of epizoic diatoms in the Amazon Basin - Luticola deniseae (Wetzel et al., 2010) and Tursiocola podocnemicola (Wetzel et al., 2012) - and hypothesized that the presence of both these species in freshwater ecosystems is closely linked to the presence of both turtles and river dolphins, as these animals play a key role both as substrates and as dispersal vectors. However, how assemblage composition may vary among turtle species, has hardly been explored.
Habitat fragmentation, illegal trafficking, and hunting currently pose a great threat to the continued existence and livelihood of Neotropical freshwater-turtles (Rhodin et al., 2011; Páez, Morales-B, Lasso, Castaño, & Bock, 2012). Gaining knowledge of these turtles’ ecology is, moreover, crucial for designing effective measures to ensure their conservation. A first step in this direction is characterizing the epizoic assemblages inhabiting their carapaces, since species composition might be related not to only environmental conditions but also the autoecology of the turtles (Soylu, Gönulol, Sukatar, Ayaza, & Tok, 2006). Majewska et al. (2015), for instance, found that some diatom traits served as bio-indicators for marine turtle foraging sites. Furthermore, Soylu et al. (2006) suggested that the abundance of some epibiont species is explained by the feeding behaviour of some freshwater turtle species. These autoecological aspects of turtles may contribute to the estimation of their home range, which is a relevant factor in delineating conservation areas (Stamps & Swaisgood, 2007).
The aim of this study was to assess and compare diatom assemblages inhabiting carapaces of some of the endangered turtle species in the Neotropical region. Diatom assemblages were sampled from turtles kept in captivity and cared for by the specialists at the Roberto Franco Tropical Station (Villavicencio, Colombia). Although kept in one place, characterizing diatom assemblages from carapaces different in form and size represents a great opportunity (1) to study the degree of association between diatom assemblages and turtle species and (2) to evaluate if various turtle species could disperse diatoms equally as well. If there are specific associations between turtle and diatom species, characterizing diatom assemblages would be useful for improving turtle conservation plans. Specific associations could serve as proxies of turtle’s feeding behaviour and foraging sites, or population’s connectedness, which are important elements for delineating species home range.
Materials and methods
Study site: The study was carried out at the Roberto Franco Station for Tropical Biology (Villavicencio, Colombia). As a part of a conservation and rehabilitation program, the station cares for reptile species recovered from illegal traffickers. Freshwater turtles are maintained in artificial ponds with a surface area of 9 m2 and a maximum depth of approximately 0.6 m. The pond water is renewed with river or ground water every 15 d. Water quality parameters varied only slightly among ponds. The average water temperature was 25.9 ºC (Standard Error from the Mean - ‘SE’ = 0.45, n = 7); pH 6.19 (SE = 0.09); and dissolved oxygen concentration 4.9 mg/L (SE = 0.21). The average conductivity was 106.3 µs/cm (SE = 0.75); nitrate concentration 0.24 mg/L (SE = 0.08); phosphate concentration 1.2 mg/L (SE = 0.25); and ammonium concentration 1.5 mg/L (SE = 0.3).
Each pond contained between three and 15 individuals of the same species, which were fed according to their natural diets. Turtle carapaces of seven native species were sampled on December 1st, 2015: Rhinoclemmys melanosterna (Gray, 1981) or “Palmera” (n = 3); Rhinoclemmys diademata (Mertens, 1954) or “Inguensa” (n = 3); Kinosternon dunni (Schmidt, 1947) or “Morrocoy de Dunn” (n = 3); Podocnemis lewyana (Duméril, 1852), o “Tortuga de río” (n = 3); Podocnemis unifilis (Troschel, 1848) or “Terecay” (n = 3); Podocnemis vogli (Müller, 1835) or “Galápago” (n = 3); and, Podocnemis expansa (Schweigger, 1812) or “Charapa” (n = 3). At the time of sampling, the carapaces of all turtle species had not been cleaned by station staff for about a month, thus allowing sufficient time for diatom colonization (Stevenson, Bothwell, Lowe, & Thorp, 1996). See Páez et al. (2012) for details about species biology and conservation status.
Diatom sampling and processing: For each species, three individuals were randomly selected for study. The total area of each carapace was measured by tracing the carapace onto paper with a known weight per area, and then weighing the paper. For each carapace, an area of approximately 19.2 cm2 (divided into three sections by a slide frame) was brushed with a tooth brush. The samples thus obtained were preserved in 4 % formalin. In the laboratory, samples were oxidized using 30 % hydrogen peroxide and mounted on slides using Naphrax® following the protocol described in Bellinger and Sigee (2015). A total of 400 valves were counted for each sample, except those from P. unifilis and P. vogli, for which only 100 valves were counted owing to their low abundance on slides. Diatoms were identified to the lowest taxonomic-level possible following specialized keys (Krammer & Lange-Bertalot, 1986, 1991; Metzeltin & Lange-Bertalot, 1998, 2007; Rumrich, LangeBertalot, & Rumrich, 2000).
Data analysis: The structure and composition of diatom assemblages were characterized using taxonomical and trait-based approaches. Taxa richness and Simpson indices were used to compare diatom diversity among turtle species. Comparisons were made using pairwise T-tests adjusted by the Bonferroni correction. A generalized model was used to test the relationship between carapace area and diatom richness, while a linear model was used to investigate the connection between carapace area and diatom diversity. An ANOSIM analysis (Clarke, 1993) was used to compare assemblage composition among turtle species, and non-Metrical multidimensional scaling (‘nMDS’; Kruskal, 1964) was used to explore how the taxonomical composition varied among turtle species. The Horn Index was selected as the distance index for this ordination, since it does not give so much weight to rare taxa (Jost, Chao, & Chazdon, 2011). A posterior ANOSIM analysis was performed to evaluate if the groups of turtles drawn by the nMDS were different in terms of diatom species composition.
Three traits - diatom biovolume, life-form, and the type of attachment - were used to assess the functional composition of the diatom assemblages. Each species or genus was assigned to one trait category following Lange et al. (2016). According to these authors, valve biovolume has five categories: nano (5 ≤ 100 µm3), micro (100 ≤ 300 µm3), meso (300 ≤ 600 µm3), macro (600 ≤ 1 500 µm3), and large (> 1 500 µm3). Life-form has two categories: ‘colonial’ or ‘solitary’. And, type of attachment has three categories: ‘tightly-attached’, ‘attached’, and ‘not-attached’. Trait data were obtained from existing trait-databases (Lange et al., 2016) and complemented by observations in the laboratory, e.g. diatom biovolume being identified to the genus-level. For each turtle species, the relative abundance of diatoms possessing a specific trait category was presented in the form of stacked bar graphs. Trait and ordination analyses were performed using the relative abundances of the traits. All analyses and figures were done in R language (R Core Team, 2016) using the package ‘Vegan’ (Oksanen et al., 2013).
Results
A total of 45 diatom species were found among all carapaces sampled from the turtle seven species (Appendix), and diatom species composition differed among the turtle species (ANOSIM R = 0.665, P < 0.001, permutations = 9 999). In fact, three distinct groups of turtle species (Fig. 1) can be defined, each with a significantly different diatom species composition (ANOSIM R = 0.965, P < 0.001, permutations = 9 999). The first group (Group 1) consisted of turtle species whose carapaces’ diatom assemblages were dominated by Navicula sp., namely R. melanosterna, R. diademata, and P. lewyana. The second group (Group 2) included the other four turtle species (K. dunni, P. unifilis, P. vogli, and P. expansa) and was characterized by highly diverse diatom compositions. The third group (Group 3) consisted of just one turtle, which as one of the three individuals from the species P. vogli and differed from the members of Group 2 in terms of its composition. Certain diatom species were only found on the carapace of this one individual. These species included Brachysira serians, Aulacoseira sp., Eunotia exigua, Gomphonema insigne, and Stauroneis anceps.
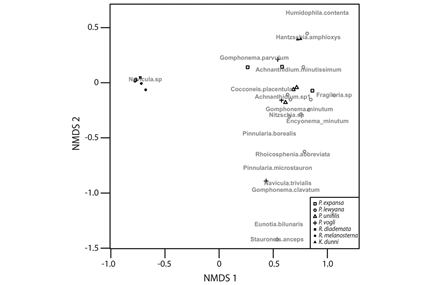
Fig. 1 Non-metrical multidimensional scaling (nMDS) plot using the Horn-distance index. Turtle species are ordered according to the composition of diatom assemblages inhabiting their carapaces (Stress = 0.019).
Regression models showed that carapace area was neither a good descriptor of species richness nor of species diversity among the epibiont diatom assemblages (Fig. 2), for the greatest richness and diversity were observed in four species with significantly different carapace areas: P. expansa, P. unifilis, K. dunni and P. vogli; Kruskal-Wallis test: H-value = 9, df = 3, P = 0.03. (Fig. 3A). T-test pairwise comparisons showed that richness and diversity were not statistically different among these four species (P > 0.05), but that there was a statistically significant difference between those four species and R. melanosterna, R. diademata, and P. lewyana (P < 0.01). Consequently, in terms of richness and diversity, it can be said that there were two groups of turtles: one with diverse diatoms assemblages and another with assemblages composed of five or less diatoms species (Fig. 3).
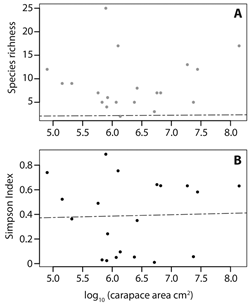
Fig. 2 Species richness (A.) and Simpson index (B.) regressed against turtles’ carapace area (log-transformed). Dotted lines represent the line of best fit for each model.
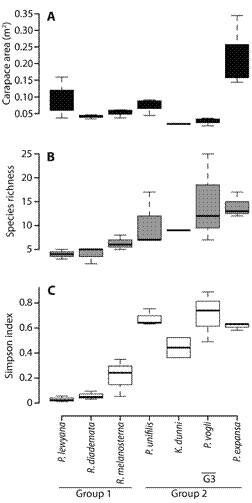
Fig. 3 Box-and-whiskers plot of carapace size (A.), species richness (B.), and diversity in terms of Simpson index (C.) of diatom assemblages inhabiting the different turtle species. Turtles have been ordered along the x-axis from lowest to highest species richness.
The diatom assemblages of these three groups also differed in terms of their trait composition (Fig. 4). As regards diatom attachment, Group 1 mainly consisted of nonattached forms such as Navicula sp., while Groups 2 and 3 consisted of tightly-attached and attached forms (Fig. 4A). In terms of diatom life-forms, Group 1 was mainly composed of solitary life-forms, whereas Groups 2 and 3 were principally populated by colonial lifeforms (Fig. 4B). As for biovolumme, all Group 1 diatoms were in the “micro” category, with the exception of two Achnanthidium species which were in the “nano” category. In contrast, Groups 2 and 3 mainly consisted of “nano” diatoms, although some diatoms belonging to other size categories were also present (Fig. 4C). The relative abundance of biovolume categories varied among the turtle species, with the greatest diversity in biovolume occurring in P. vogli. For this reason, the difference between P. vogli individual from Groups 2 and 3 was further investigated (Fig. 5). The Group 3 individual was found to differ from those in Group 2 on account of a greater abundance of larger diatoms (categories defined by sizes > 300 µm3), which were tightly-attached and/or had colonial life-forms.
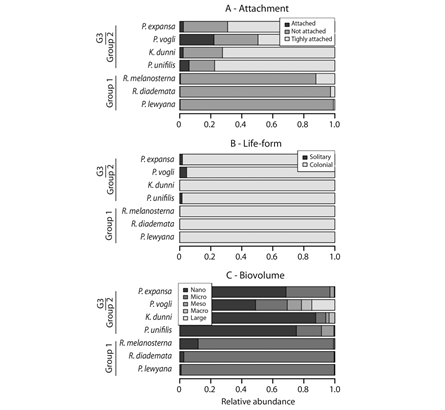
Fig. 4 Trait composition of diatom assemblages inhabiting turtle carapaces. Turtle species are ordered, from bottom to top, according to increasing diatom species richness.
Discussion
This study is one of the first to assess and compare the association between turtle carapaces and the diatom life-forms inhabiting them, in the Neotropical region. Although it is a small-scale study carried out on turtles in captivity, its findings show that turtle carapaces are not uniformly colonized by the same diatom species. Two general groups of turtles, defined by the structure and composition of their diatom assemblages, emerged: One whose carapaces were colonized by only one or two diatom species (e.g. Navicula sp. or Achnanthidium sp.) and another whose carapaces were colonized by a diversity of diatom species with diverse traits. Given that the artificial ponds were similar to each other in terms of water quality and physical disturbance, diatom assemblage structure and composition on turtle carapaces might be dependent on several extrinsic and intrinsic factors, such as carapace size, physical stress or competence, and the regional pool and successional stage of assemblages (Tumlison & Clark, 1996; Soylu et al., 2006; Wu & Bergey, 2017). This study’s results suggest that carapace size is not the best descriptor of diatom richness and diversity. Although carapace roughness and successional stage were not directly measured, the analysis of assemblage trait compositions suggests that these two factors could explain the observed differences between carapaces in terms of species richness, composition, and diversity.
As regards carapace roughness, the attachment point theory (Scardino, Harvey, & De Nys, 2006; Scardino, Guenther, & De Nys, 2008) states that substrate roughness constrains the presence of diatoms, depending on their valve size or attachment form. Hence, since only small diatoms with smaller points of attachment (“non-attached” category), such as Navicula sp., are capable of inhabiting rough substrates (Sweat & Johnson, 2013), it can be reasoned that the turtle carapaces with less diatom diversity (e.g. carapaces of R. melanosterna, R. diademata and P. lewyana) were rougher. In contrast, bigger diatoms with larger points of attachment are capable of colonizing smooth substrates (Sweat & Johnson, 2013). From this, it follows that the turtles species inhabited by larger and attached or highly-attached diatoms (such as Cocconeis placentula, Gomphonema parvulum, Placoneis elginensis) had smooth carapaces. Because these latter diatom species were common on the carapaces of K. dunni, P. vogli, P. unifilis, and P. expansa, it is likely that these turtle species had smooth carapaces. The foregoing implies that carapace roughness might be an important factor in determining the structure of epizoic assemblages. Nonetheless, actual measurements of carapace roughness are needed in order to confirm this hypothesis.
The successional trajectories of each diatom assemblage could also explain the three groups of turtles defined in the nMDS. The P. vogli individual, which constituted Group 3, had a greater richness of unique species than the turtles in Group 2. These unique species were mainly unattached diatoms of larger size (meso, macro, and larger categories). Diatoms with these traits (e.g. Eunotia binularis, Gomphonema insigne, Gomphonema clavatum, Pinnularia microstauron) are characteristic of late-successional stages (Stenvenson et al., 1996 and references therein), suggesting that the assemblage of the P. vogli individual had reached a more advanced successional stage at the time of the sampling. Considering that all the carapaces had not been cleaned for about a month, the early-successional assemblages observed in Group 1 appear to be more linked to carapace roughness, as discussed above, or other factors inhibiting assemblage development, as will be described below.
This study’s results may also have been influenced by a number of unmeasured factors, such as: (1) the overall “regional” pool of diatom species in the ponds, (2) the time that the turtles spent outside the water, and (3) biofilm thickness. The regional diatom species pool can be a major explanatory factor of diatom richness on turtle carapaces. Wu and Bergey (2017), for instance, showed that biogeographical patterns in diatom species explained the species composition on the carapaces of turtles from different regions of the United States. Consequently, characterizing the available diatom species pool in turtle habitats could improve our understanding of the relationship between the “regional” and local species pools inhabiting carapaces. On the other hand, Tumlison and Clark (1996) stated that the movement behaviour of turtles affects carapace appearance (clean, muddy or mossy), which, in turn, constrains the richness of epibiont species. R. melanosterna and R. diademata are turtles that spend a significant amount of time outside the water, that is to say, they are “semiaquatic turtles” (Páez et al., 2012). Their time outside the water could be a stress factor affecting biofilm thickness and, consequently, the composition of epibiont diatoms on their carapaces. Species capable of colonizing carapace crevices might be more tolerant to this stressor (Bergey 1999, 2005) and, hence more likely to populate the carapaces of semiaquatic turtles. In light of the foregoing, measuring the overall “regional” pool of diatom species, the time that the turtles spend outside the water, and biofilm thickness appear be essential for determining what actually gives rise to the structure of epibiont communities in aquatic ecosystems.
Several studies have demonstrated that characterizing epibiont assemblages give some clues as to the auto-ecology of turtles, which is of great importance in effectively delineating conservation areas based on feeding behaviour (Soylu et al., 2006), foraging sites (Majewska et al., 2015), and/or the degree of connectivity between populations of the same species (Caine, 1986). Our results give a first indication 1) as to which diatom species might be prone to inhabit the carapaces of a certain turtle species and 2) as to why this might be the case. While carapace roughness emerged as the most plausible hypothesis for explaining this relation, other species-specific behavioral factors, such as feeding behavior and time spent outside the water, might be also relevant.
Further research, both in captivity and in natural conditions, is needed to establish which of these factors give rise to the structure of diatom assemblages. Once the relationship between these factors and diatom assemblage structure has been established, the latter could serve as a proxy for species auto-ecology and be used for designing conservation plans. An example of this is Caine (1986), who, by assessing epibiont communities, found that conservation plans for loggerhead sea turtles required a reappraisal because of the disconnection between populations along the Atlantic coast of the USA.
Characterizing epibiont assemblages on turtle carapaces can also contribute to our understanding of diatom distribution on larger scales. Unidirectional streams and rivers restrict diatom recolonization in upstream zones (Kristiansen, 1996). It has been suggested that non-attached diatoms are more abundant in low zones of a river network, as they are less resistant to flow-induced stress (Liu et al., 2013). Thus, these diatoms should have a mechanism to persist and recolonize upstream zones (Kristiansen, 1996). Our findings show that non-attached diatoms can colonize turtle carapaces, suggesting that turtles could be vectors moving non-attached diatoms to the rivers’ upstream sections. Nevertheless, further research is needed to establish what the potential role of turtles is in the recolonization of diatom species in upstream zones.