The ecological and ethological aspects in the larval feeding of fishes have received attention from aquatic ecologists and fishery biologists (Lazarro, 1987; Rao, 2003). Larval feeding for carnivorous fishes is one of the key aspects for the commercial aquaculture and one of the greatest challenges in accomplishing fish production in controlled culture systems (Zambonino & Cahu, 2007; Nguyen, Reinertsen, Wold, Tran, & Kjørsvik, 2010). In North America, Central America and Cuba, the overfishing of gars is one of the main factors related to its population decline (Mendoza, Aguilera, & Ferrara, 2008). The increasing demand among gar producers, local fisheries and aquarists, requires improved food supply for fish larvae in controlled culture systems for exploitable native species. For instance, in Southeastern Mexico, an increasing demand for the tropical gar, Atractosteus tropicus (Gill, 1863), due to a high local consumption, sport fishing, handicrafts and cultural value, requires improvement in ex situ production (Mendoza, Aguilera, Rodriguez, González, & Castro, 2002). Unfortunately, in Mexico, aquaculture techniques have been introduced to the country to cultivate mainly non-native species (e.g. Oreochromis spp., Micropterus salmoides, Cyprinus carpio), which in some cases pose a potential risk for native aquatic fauna through predation, competition and niche overlap (Zambrano, Perrow, Aguirre-Hidalgo, & Macías-García, 1999; Tapia & Zambrano, 2003; Domínguez-Domínguez, Pérez-Ponce de León, Martínez-Meyer, & Zambrano, 2006; Córdova-Tapia, Contreras, & Zambrano, 2014).
Recently, important efforts have been focused on developing biotechnology for the culture and reproduction in controlled conditions of A. tropicus in Southeast Mexico, not only for the mass production of food, but also for the conservation and reestablishment of wild populations by artificial introductions (Márquez-Couturier et al., 2006). Many commercially important fish are oviparous and the hatchlings are independent of external diet as long as the yolk persists; however, due to rapid growth and development in oviparous fish, this source of nutrition usually lasts only 2-4 days (Lazarro, 1987; Morales-Ventura, Nandini, & Sarma, 2004). For effective feeding by larval fish, zooplankton prey should be available at a certain critical density and size, in order to promote survivorship and growth at early stages (Juanes, 1994; Cunha & Planas, 1999). This can vary depending on the larval age and the natural feeding habits of the species in turn (Laurel, Brown, & Anderson, 2001). In this context, ecological and ethological aspects of fish feeding are useful in preventing larval mortality, permitting an increase in growth rate, reducing cannibalism and increasing the survivorship of the larvae in reintroduction programs (Colgan, Brown, & Orsatti, 1986; Katavic, Jug-Dujakovic, & Glamuzina, 1989; Atencio-García & Zaniboni, 2006). Although food and feeding aspects of many important fish in aquaculture such as catfish, carps and trouts have received considerable attention and information about feeding habits and feeding behavior of larvae of commercial carnivorous native fishes, is unknown or less available (Jhingran, 1991; Dutta, 1996).
The tropical gar presents carnivorous larval stages and feeding and nutrition in controlled conditions is critical to prevent high mortalities (Márquez-Couturier et al., 2006). The use of zooplankton as live food increases and improves fish production (Katavic et al., 1989; Atencio-García & Zaniboni, 2006); however, even when some ethological aspects of feeding behaviour are well-documented, the supply of adequate live food could be difficult and expensive (Sarma, López-Rómulo, & Nandini, 2003). Thus, to develop proper diets considering feeding aspects, especially during the early stages of larval development, can reduce mortality, enhance growth of fish larvae and reduce production costs in the culture of carnivorous tropical native fish. Therefore, the goal of the present work was to determine the optimal prey species and densities for rearing the tropical gar A. tropicus in the early larval stages (first 8 weeks), quantifying the prey selection and determining the feeding behavior and functional response using three zooplankton species as prey; following the hypotheses: i) A. tropicus will show a carnivorous functional response since external feeding is present; ii) the prey selection of this fish species is related not only to prey species but also to differences in prey densities in the environment; and iii) fish will handle and feed on prey more successfully with increasing age.
Material and methods
Experimental conditions: Fish eggs were donated from the Aqua-Pet fish farm located in the State of Tabasco, Mexico. The spawning was not induced and 98 % of eggs were fertilized obtaining 125 eggs of A. tropicus which were maintained in two aquaria of 50 L (62-63 eggs per aquarium) with constant aeration and temperature controlled by 300 W heaters (AQUATOP, Model GH-300, Brea, CA, USA). Experimental conditions and results are presented as mean ± standard error. Daily, temperature (27 ± 0.18 ºC), dissolved oxygen (7.5 ± 0.05 mgL-1) and pH (8.2 ± 0.05, N = 10 aquaria) were measured in each aquarium using a laboratory thermometer, an oximeter (YSI, Model 51B, Yellow Springs, OH, USA) and a pH meter (CONDUCTRONIC, ModelPC18, Puebla, Puebla, Mexico); weekly, 20 % of the water in each aquarium was replaced using a syphon vacuum cleaner (PEN-PLAX, 16 inch gravel vac, Hauppauge,NY, USA). In all 106 hatched larvae were obtained and placed as stock in two aquaria of 250 L maintained under the same conditions as the eggs and subjected to 12:12 light:dark hours photoperiod using fluorescent lamps until complete yolk absorption and exogenous feeding was needed. In each stocking aquarium (N = 38), temperature (26.5 ± 0.2 ºC), pH (8.0 ± 0.01) and dissolved oxygen (7.1 ± 0.02 mgL-1) were measured every third day along the eight weeks of the experiment using the instruments mentioned above.
For experiments as well as for routine feeding of the larvae, we cultured separately three species of zooplankton (2 cladocerans and 1 branchiopod). Daphnia pulex (1 500 ± 0.65 µm, N = 80) and Moina macrocopa (1 000 ± 0.6 µm, N = 80) were cultured in 10 L transparent plastic bottles with EPA medium (Anonymous 1985) in 1 L of distilled water at 25 ºC ± 0.16 (N = 24) and pH 7.9 ± 0.02 (N = 24). Artemia franciscana 1 700 ± 0.62 µm, N = 80) was cultured in eight 200 L plastic pools, at salinity of 45 gL-1 , temperature 26.05 ± 0.34 ºC and pH 8.2 ± 0.03 (N = 24). Once a week, 10 individuals of each zooplankton species were randomly collected from the culture bottles to obtain the size (length) using a micrometer 10:100 (8 weeks x 10 individuals = N = 80 per species). The culture medium for cladocerans was replaced 100 % every three days, and for the branchiopod 20 % of the total water in each pool was replaced every week. For zooplankton culture, the single-celled green alga Chlorella vulgaris was cultured using Bold’s basal medium (Borowitzka & Borowitzka, 1988) in 10 transparent containers of 2 L maintained with continuous aeration and fluorescent illumination. Temperature and pH for this culture was measured once a week in each bottle (25 ºC ± 0.11, pH 7.9 ± 0.01, N = 80). The green alga was given at concentration of 0.6x106 cells mL-1 every day as food for zooplankton. For feeding zooplankton, we routinely harvested the log phase algae, centrifuged at 4 000 rpm for 5 minutes and re-suspended in moderately hardwater EPA medium. Algal density was quantified using a hemocytometer and diluted to the desired density for feeding zooplankton.
The experiments were conducted during the first eight weeks of larval stage, starting since the first exogenous feed (4-5 days after hatching) using fish larvae of known age. Prior to each experiment, all larvae were starved for 2 hours following Tilseth and Ellertsen (1984) in separated aquaria with pre-filtered water (50 µm mesh). In order to reduce stress on the fish larvae, for each experiment we filtered preconditioned (water in which A. tropicus individuals were maintained) water with a 50 µm mesh to use in the experimental vessels (150 mL plastic vessels; dimensions: length 8 cm, width 5.5 cm, height 3.5 cm) and filled with 25 mL of EPA and 25 mL of pre-filtered water and after 15 min, prey density was added according to the specific experiment. Temperature was measured in each vessel after each trial using a laboratory thermometer. Given the salinity conditions in the A. franciscana culture, this species was separated from the experimental containers 30 min before to start each experiment using plastic sieves (2 000 µm mesh size) and rinsed carefully with tap water to avoid salinity conditions in the experimental vessels. In all experiments, prey species were counted using a Pasteur micropipette until prey density was reached according each experimental design. All the different treatments for each experiment were conducted with five replicates and one fish per replicate. Standard length (Ls), gape width (GW) and gape length (GL) of fish were measured to the nearest mm weekly from 15-18 larvae (total N = 131) taken at random during the eight weeks of the experiment using a digital calliper (TRESNA® EC16; Guangxi, China); GW was measured every time at the half of the GL.
Functional response: In order to study the functional response of A. tropicus, we selected one fish larva at random into each of the experimental vessel; the branchiopod and cladoceran species were offered independently at six different densities (Table 1). The fish larvae were allowed to feed for 45 min and after the feeding period, were carefully removed and returned to the culture aquaria. The remaining zooplankton in the experimental vessels were fixed with 10 % formalin and quantified using a stereo microscope at 20X magnification. The number of prey consumed by each larva was determined as the difference between the initial and the final prey count.
Table 1: Experimental design used to determine the optimal prey for Atractosteus tropicus (Gill)
Experiment/trail time and Temperature | Prey species | Density (ind. mL-1) | Index / model for analyzes | Calculation | Factors | Interpretation |
Functional response 45 minutes 25.7 ºC ± 0.3 | A. franciscana D. pulex M. macrocopa | 0.2, 0.5, 1.0, 2.0, 4.0, 8.0 | Holling (1966) | F = (Fmax X C) (C + K) -1 | F = feeding rate (prey consumed/ unit of time) C = prey density Fmax = maximum consumption K = prey density at Fmax/2 | If the linear parameter estimate has/is: no significant slope = Type I significantly negative slope = Type II significantly positive and the quadratic estimate is significantly negative = Type III |
Functional curves (Lampert and Sommer, 1997) | y = ax/(b + x) | x = number of prey offered y = number of prey consumed a =intersection of y b = slope | ||||
Prey selection 45 minutes 25.5 ºC ± 0.4 | A. franciscana + D. pulex + M. macrocopa | i) 0.12 + 0.25 + 0.25 ii) 0.25 + 0.50 + 0.50 iii) 0.50 + 1.0+ 1.0 | Manly’s α (Krebs 1993) | α i = r i / n i (1/Σ(r j / n j )) | α i = Manly's alpha for prey type i, r i , r j = proportion of prey type i or j (i and j = 1, 2, 3 ..., m) n i , n j = proportion of prey type i or j in the environment m = number of prey types possible | When: α i = 1/ m, feeding is not selective α i > 1 /m, prey species i is selected α i < 1 /m, prey species i is avoided |
Feeding behavior 10 minutes 25.8 ºC ± 0.4 | A. franciscana D. pulex M. macrocopa | 1 | Differences in prey consumption among fish age | Sum of each event | Number of: Encounters, attacks, captures, ingestions, prey rejections and prey escapes | Changes in the number of events along the age of the fish larvae |
The functional response describes the prey consumption rate of a predator as a function of the density of a given prey. In biological terms, the functional response Type I is found in passive predators, where the number of prey caught is proportional to prey density, thus prey mortality is related to constant predation; in Type II the search rate of prey is constant, and prey mortality declines in relation to prey density; and Type III is related to predators that increase the search activity of prey related to the prey density (Krebs & Davies, 1993). Therefore, functional response curves were analyzed using the model of Holling (1966) and calculated by the equation in Lampert and Sommer (1997) described in Table 1.
To distinguish between Type I, II and III functional responses, we used the procedures described by Trexler, McCulloch, and Travis (1988), which involves the logistic regression of the proportion of prey eaten related to the density of prey offered (Table 1). We used Analyses of Variance (ANOVA) to test for differences between the means of prey consumption at the asymptote of the functional response curves and the maximum prey consumption (Fmax) in relation to the age of the fish larvae and prey species offered.
Prey selection: For determining prey selection, we used the zooplankton prey in three different densities based on Greene (1983), Nandini and Sarma (2000) and Gallardo-Alanis, Sarma, and Nandini (2009) (Table 1). The fish larvae were allowed to feed for 45 min and thereafter they were removed. The number of prey consumed by each larva was determined as the difference between the initial and the final prey count. We also randomly dissected out 2-3 guts per week of fish larvae to make sure that the number of prey consumed tallied with the counts. Prey selectivity was calculated following Manly’s α (Krebs & Davies, 1993) and Two-way ANOVA was performed to test for statistical differences among consumption of prey types (Factor A) and densities (Factor B), and post hoc analysis for paired comparisons was used (i.e. Tukey-Kramer HSD).
Feeding behaviour: For experiments of feeding behaviour, the density of prey used was 1 ind. mL-1 for cladocerans as well as for the branchiopod. We quantified the components of feeding behaviour (encounter, attack, capture, ingestion, prey rejection and prey escapes) based on 10 minutes of direct observation of a total of 15 fish larvae placed individually in the experimental vessels using a lamp with a magnifying glass and following Greene (1983), over the eight weeks of the experiment. We considered an encounter as the number of occasions in which the predator recognized its prey. This was evident by changes in the swimming behaviour and visual contact of the fish larvae and the prey. Attacks were the number of assaults made by predator towards the prey with intent to capture it. Capture is considered when the prey is in the mouth of predator; in this moment, the prey can be ingested or escape from the predator’s mouth. The ingestion and escape are easily differentiated by the fish and prey movements.
Differences in prey consumption were tested using ANOVA and post hoc analysis for paired comparisons (i.e. Tukey-Kramer HSD). Relations of the Ls to GW and GL were calculated using lineal regression models. Data analyses for all the experiments were performed with the libraries stats and MASS (Venables & Ripley, 2002) using the statistical computer environment R 3.2.0 (R development core team, www.r-project.org).
Results
Functional response: The functional response curves of A. tropicus larvae at the different ages showed an approximation to the asymptote when more than 4 ind. mL-1 (Figure 1, Figure 2 and Figure 3). Overall, a Type II functional response with any prey species offered was present as observed in the significantly negative slopes (Table 2). The average of the maximum consumption during the eight weeks of A. franciscana (105 ± 18.73) and M. macrocopa (105 ± 10.9) was higher than that for D. pulex (100 ± 20.85). However, the consumption of A. franciscana and D. pulex was similar during the first 4 weeks, but during the next weeks the consumption of D. pulex decreased in comparison to A. franciscana. The consumption of M. macrocopa was high during the 8 weeks; however no clear changes in the pattern of consumption were generated in relation to fish age when M. macrocopa was offered.
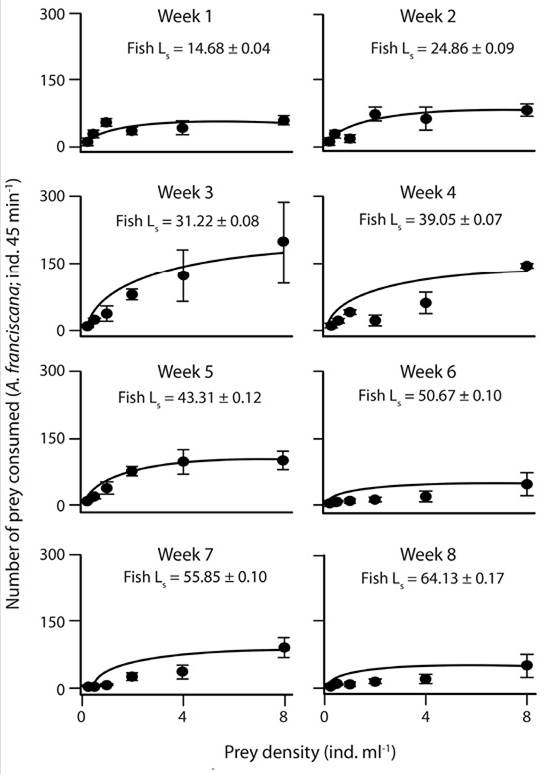
Figure 1 Functional response of larval Atractosteus tropicus (Gill) based on the model of Holling using A. franciscana as prey. Each point represents the mean ± SE, and Ls = fish standard length.
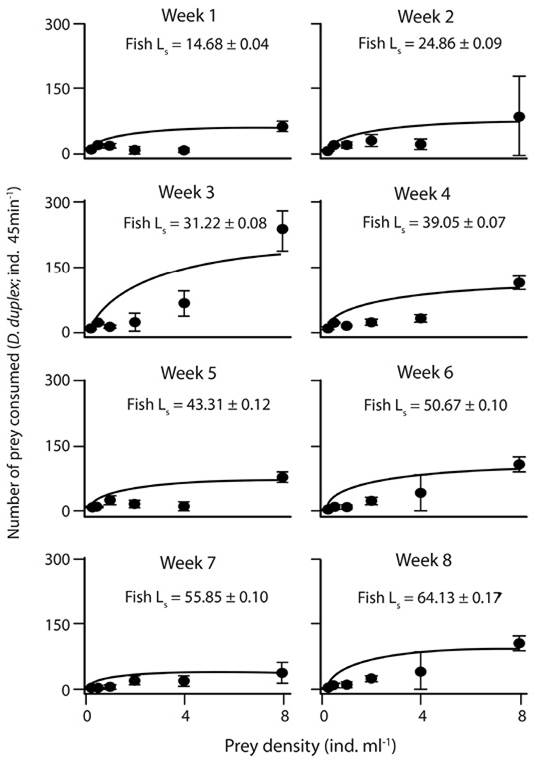
Figure 2 Functional response of larval A. tropicus based on the model of Holling using D. pulex as prey. Each point represents the mean ± SE, and Ls = fish standard length.
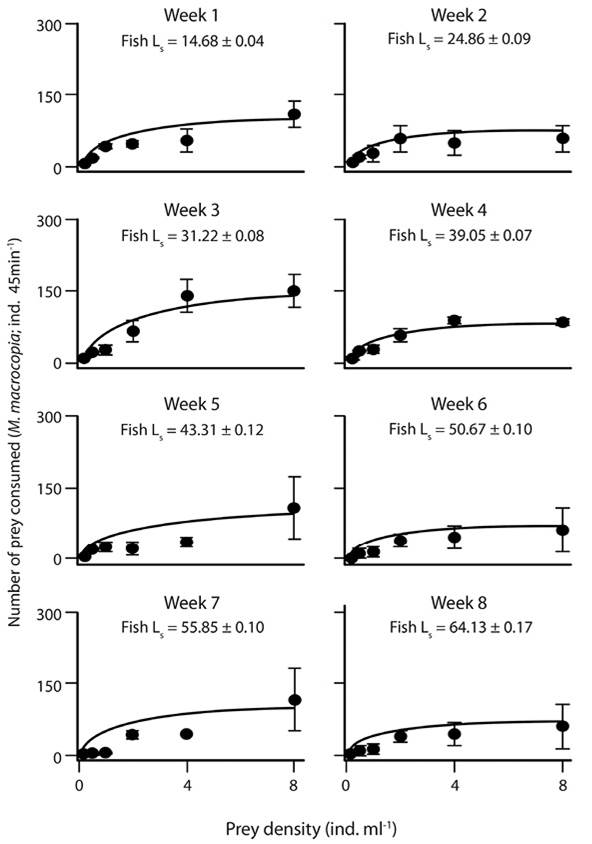
Figure 3 Functional response of larval A. tropicus based on the model of Holling using M. macrocopa as prey. Each point represents the mean ± SE, and Ls = fish standard length.
Table 2: P-value of the Slope, Fmax (mean ± SE), and Fmax vs. asymptote P-value regarding the functional response of A. tropicus using the three prey species
Prey species/fish age (weeks) and Ls | P-Slope | Fmax | P-Fmax vs asymptote |
A. franciscana | |||
1 (14.68 ± 0.04) | -0.007* | 59.75 ± 3.87 | 0.02 |
2 (24.86 ± 0.09) | -0.006* | 87 ± 6.29 | 0.23 |
3 (31.22 ± 0.08) | -0.004* | 212.25 ± 30.28 | 0.08 |
4 (39.05 ± 0.07) | -0.001* | 148 ± 2.80 | 0 |
5 (43.31 ± 0.12) | -0.007* | 117.75 ± 7.56 | 0.03 |
6 (50.67 ± 0.10) | -0.002* | 52.66 ± 8.31 | 0.09 |
7 (55.85 ± 0.10) | 0.000* | 91.66 ± 10.05 | 0.04 |
8 (64.13 ± 0.17) | -0.002* | 74 ± 10.45 | 0.15 |
D. pulex | |||
1 (14.68 ± 0.04) | -0.002* | 63.75 ± 5.19 | 0 |
2 (24.86 ± 0.09) | -0.002* | 87 ± 41.19 | 0.44 |
3 (31.22 ± 0.08) | 0.003* | 234 ± 20.32 | 0 |
4 (39.05 ± 0.07) | -0.001* | 113.75 ± 7.18 | 0 |
5 (43.31 ± 0.12) | -0.001* | 77.33 ± 5.22 | 0 |
6 (50.67 ± 0.10) | 0.001* | 106.33 ± 7.77 | 0.01 |
7 (55.85 ± 0.10) | -0.002* | 40.33 ± 9.48 | 0.34 |
8 (64.13 ± 0.17) | -0.003* | 74 ± 3.49 | 0.01 |
M. macrocopa | |||
1 (14.68 ± 0.04) | -0.004* | 114 ± 10.10 | 0.01 |
2 (24.86 ± 0.09) | -0.007* | 84 ± 4.05 | 0 |
3 (31.22 ± 0.08) | -0.004* | 170.5 ± 10.53 | 0 |
4 (39.05 ± 0.07) | -0.006* | 93 ± 1.09 | 0 |
5 (43.31 ± 0.12) | -0.001* | 107.66 ± 28.97 | 0.25 |
6 (50.67 ± 0.10) | -0.003* | 76.33 ± 15.07 | 0.25 |
7 (55.85 ± 0.10) | 0.001* | 117.66 ± 29.18 | 0.28 |
8 (64.13 ± 0.17) | -0.001* | 73.66 ± 3.38 | 0.09 |
Asterisk denotes P-value < 0.05 for the slope. Values in parenthesis denotes the mean ± SE of the Standard length (Ls, in mm) of the fish at the determined week of age Experiment was performed at 25.7 ºC ± 0.02.
Prey selectivity: The counts in the vessels matched with the dissected guts (± 2 ind.). The two-way ANOVA using Manly’s α showed statistical differences among the prey species selected (excluding the 3 and 5 week). Overall, no statistical differences where shown among prey densities (Table 3); the A. franciscana was selected in the three different densities during the first 3 weeks of age; after the third week, this prey species was only selected in the 4-5 week at density ii. After the third week of age of the fish larvae, M. macrocopa are selected during the next weeks until the 8 week; D. pulex was rarely selected throughout the experiment (Figure 4).
Table 3: Two-way ANOVAs between densities and prey species obtained from Manly’s alpha, considering A. tropicus larval age (weeks)
Source of Variation | DF | SS | MS | F |
Week 1 | ||||
Prey | 2 | 0.04 | 0.02 | 10.829* DM |
Density | 2 | 0 | 0 | 0.02 |
Prey x Density | 4 | 0.05 | 0.01 | 6.858* |
Week 2 | ||||
Prey | 2 | 0.07 | 0.03 | 4.427* AD, DM |
Density | 2 | 0 | 0 | 0.004 |
Prey x Density | 4 | 0.06 | 0.01 | 1.888 |
Week 3 | ||||
Prey | 2 | 0.02 | 0.01 | 1.649 |
Density | 2 | 0 | 0 | 0.007 |
Prey x Density | 4 | 0.02 | 0.01 | 1.172 |
Week 4 | ||||
Prey | 2 | 0.04 | 0.02 | 9.904* DM |
Density | 2 | 0 | 0 | 0.146 |
Prey x Density | 4 | 0.1 | 0.02 | 13.38* |
Week 5 | ||||
Prey | 2 | 0.02 | 0.01 | 2.387 |
Density | 2 | 0 | 0 | 0.012 |
Prey x Density | 4 | 0.01 | 0 | 0.591 |
Week 6 | ||||
Prey | 2 | 0.06 | 0.03 | 6.129* AD |
Density | 2 | 0 | 0 | 0.012 |
Prey x Density | 4 | 0.08 | 0.02 | 4.156* |
Week 7 | ||||
Prey | 2 | 0.21 | 0.1 | 44.981* AD, DM |
Density | 2 | 0 | 0 | 0.029 |
Prey x Density | 4 | 0.03 | 0.01 | 2.909* |
Week 8 | ||||
Prey | 2 | 0.1 | 0.05 | 21.943* AD, DM |
Density | 2 | 0 | 0 | 0.044 |
Prey x Density | 4 | 0.03 | 0.01 | 3.53* |
Asterisk denotes P-value < 0.05 and the concatenate superscript letters (A = A. fransiscana, D = D. pulex and M = M. macrocopa) after the F-value, indicate no differences in pair comparisons (Tukey-Kramer HSD). Experiment was performed at 25.5 ºC ± 0.03.
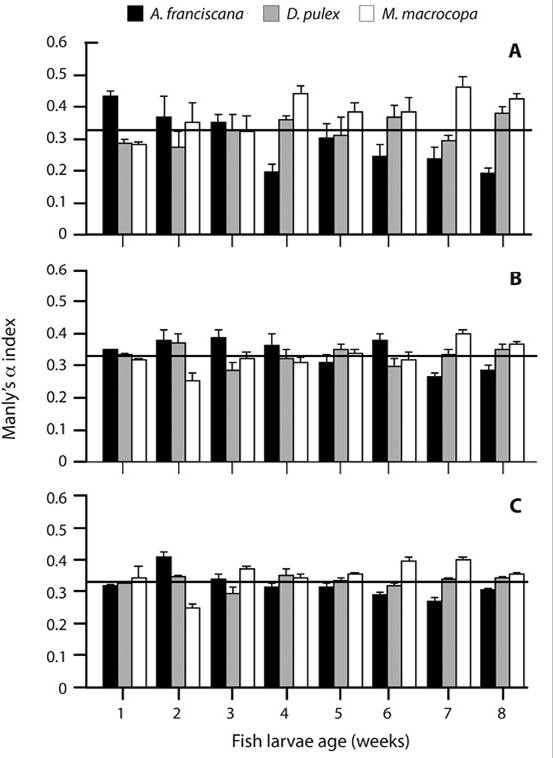
Figure 4 Prey selection based on Manly’s α using A. franciscana + D. pulex + M. macrocopa in a) 0.12 + 0.25 + 0.25 ind. mL-1, b) 0.25 + 0.50 + 0.50 ind. mL-1 and c) 0.5 + 1.0 + 1.0 ind. mL-1, densities along the first eight weeks of the larval stage of A. tropicus. Bars represent mean ± SE, values above the horizontal line (0.33) indicate positive selection.
Feeding behaviour: For the three different species of zooplankton, practically no rejection was registered and low escape values were found with A. fransiscana and D. pulex. Data on the increase of the Ls, GW and GL of A. tropicus during the first eight weeks of larval development showed a lineal relation (Figure 5). Regardless of the larval age, prey species were hardly rejected by A. tropicus. The trends for both cladoceran prey species were nearly similar with respect to encounters but with a less number of encounters of these prey species compared with A. fransiscana. Attack, capture and ingestion patterns for M. macrocopa decreased when larval age increased, and in the last five weeks these events were statistically low. Values for encounters for D. pulex were lower than for A. fransiscana but higher than for M. macrocopa and they were relatively constant during the eight weeks. Attack, capture and ingestion values for cladocerans were the lowest of the three prey species and were similar during the experiment (Table 4). Similar values obtained for the statistic F in captures and ingestions confirm that A. tropicus presents a high rate of success in capture and ingest all prey species used in the present study.
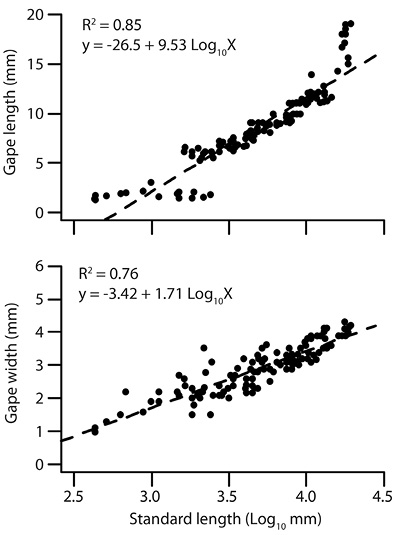
Figure 5 Linear relation between gape length (GL; upper plot), gape width (GW; lower plot) with standard body length (Ls).
Table 4: Events of feeding behavior (mean ± SE) at the different larval age of A. tropicus using the three prey species
Prey species | Fish age (weeks) | Encounters | Attacks | Captures | Ingestions | Rejections | Escapes |
A. franciscana | 1 | 7.4 ± 2.32 | 0.8 ± 0.49 | 0 ± 0 | 0 ± 0 | 0 ± 0 | 0 ± 0 |
2 | 31.6 ± 3.69 | 10.8 ± 3.05 | 9.8 ± 3.02 | 9.8 ± 3.02 | 0 ± 0 | 2 ± 0.32 | |
3 | 28.6 ± 4.87 | 7 ± 2.61 | 5.8 ± 2.4 | 5.8 ± 2.4 | 0 ± 0 | 1 ± 0.32 | |
4 | 24.6 ± 2.71 | 1.8 ± 1.2 | 1 ± 0.77 | 1 ± 0.77 | 0 ± 0 | 1 ± 0.63 | |
5 | 22.2 ± 2.13 | 3.6 ± 1.17 | 2.4 ± 1.03 | 2 ± 1.14 | 0 ± 0 | 1.2 ± 0.38 | |
6 | 26.4 ± 3.92 | 2.6 ± 0.93 | 2.2 ± 0.73 | 2.2 ± 0.73 | 0 ± 0 | 0.4 ± 0.25 | |
7 | 17.6 ± 2.11 | 1.6 ± 0.68 | 1.6 ± 0.68 | 1.4 ± 0.51 | 0.2 ± 0.2 | 0.2 ± 0.2 | |
8 | 15.4 ± 1.63 | 2.2 ± 0.58 | 2.2 ± 0.58 | 2.2 ± 0.58 | 0 ± 0 | 0 ± 0 | |
F7, 40 | 6.371* | 4.51* | 4.51* | 4.58* | 1 | 4.67* | |
D. pulex | 1 | 8.8 ± 2.06 | 5 ± 2.17 | 4 ± 2.51 | 4 ± 2.51 | 0 ± 0 | 0 ± 0 |
2 | 11.2 ± 2.75 | 1.8 ± 1.11 | 1.4 ± 1.17 | 0.2 ± 0.2 | 0.2 ± 0.2 | 0.4 ± 0.4 | |
3 | 13.2 ± 3.18 | 2.6 ± 0.6 | 1.2 ± 0.38 | 1.2 ± 0.38 | 0 ± 0 | 1.2 ± 0.58 | |
4 | 7.4 ± 2.2 | 0.6 ± 0.4 | 0 ± 0 | 0 ± 0 | 0 ± 0 | 0.6 ± 0.4 | |
5 | 17.4 ± 3.99 | 1.6 ± 0.25 | 1.2 ± 0.2 | 1.2 ± 0.2 | 0 ± 0 | 0.4 ± 0.25 | |
6 | 21.2 ± 1.53 | 1 ± 0.55 | 1 ± 0.55 | 1 ± 0.55 | 0 ± 0 | 0 ± 0 | |
7 | 13.6 ± 1.81 | 2.4 ± 0.87 | 1.8 ± 0.58 | 1.6 ± 0.6 | 0 ± 0 | 0.6 ± 0.4 | |
8 | 12 ± 0.71 | 3.2 ± 0.58 | 2.6 ± 0.51 | 2.6 ± 0.51 | 0 ± 0 | 0.6 ± 0.4 | |
F7, 40 | 1.13 | 1.95 | 1.31 | 1.81 | 1 | 1.13 | |
M. macrocopa | 1 | 18.6 ± 2.09 | 14.6 ± 2.04 | 11.6 ± 2.18 | 11.6 ± 2.18 | 0 ± 0 | 0 ± 0 |
2 | 21 ± 4.81 | 8.4 ± 3.81 | 7 ± 3.45 | 7 ± 3.45 | 0 ± 0 | 1.2 ± 0.58 | |
3 | 9.6 ± 2.07 | 3.6 ± 1.94 | 3.6 ± 1.94 | 3.6 ± 1.94 | 0 ± 0 | 0.2 ± 0.2 | |
4 | 6.6 ± 0.87 | 0 ± 0 | 0 ± 0 | 0 ± 0 | 0 ± 0 | 0 ± 0 | |
5 | 11.2 ± 3.1 | 0.4 ± 0.25 | 0.2 ± 0.2 | 0.2 ± 0.2 | 0 ± 0 | 0.2 ± 0.2 | |
6 | 17.8 ± 4.59 | 0 ± 0 | 0 ± 0 | 0 ± 0 | 0 ± 0 | 0 ± 0 | |
7 | 10 ± 1 | 0 ± 0 | 0 ± 0 | 0 ± 0 | 0 ± 0 | 0 ± 0 | |
8 | 6.8 ± 1.24 | 0.4 ± 0.25 | 0.4 ± 0.25 | 0.4 ± 0.25 | 0 ± 0 | 0 ± 0 | |
F7, 40 | 3.84* | 10.27* | 7.33* | 7.33* | 3.26* | ||
pair-comparisons (Tukey-Kramer HSD) | |||||||
A. franciscana - D. pulex | >0.05 | 0.09 | 0.09 | 0.06 | 1 | 0.22 | |
M. macrocopa-A. franciscana | >0.05 | 0.86 | 0.91 | 0.95 | 0.66 | >0.05 | |
M. macrocopa-D. pulex | >0.05 | 0.26 | 0.2 | 0.12 | 0.66 | 0.18 |
Asterisk denotes P-values < 0.05 for the comparisons among larval age. At the bottom of the table, paired comparisons (Tukey-Kramer HSD) are shown. Experiment was performed at 25.8 ºC ± 0.03.
Discussion
The consumption of live food depends on prey species size and gape size and most fish larvae of commercial importance usually have a gape size < 200 µm at early stages, restricting its diet to small prey, and fail to consume even the smaller cladocerans used in aquaculture such as Moina sp. and Ceriodaphnia sp. (Lubzens 1987; Khadka & Rao, 1986; Villegas, 1990; Gerking, 1994). In comparison to other gar fish species, A. tropicus presents high growth rate after hatching (Comabella, Hurtado & García-Galano, 2010); the smallest fish larvae at the first week of age used in the present study showed a GW of 1.0 mm and a GL of 1.3 mm, and consumed preys of sizes that range 1 500-1 000 µm, suggesting that the selectivity and feeding behaviour of this fish species could be related to the ability of handling prey successfully in the predation process. As well, the common pattern in functional response of fish larvae is highly related to prey size (Puvanendran, Salies, Laurel, & Brown, 2004; Peña-Aguado, Nandini, & Sarma, 2009); therefore, our results from the functional response experiments support the hypothesis that in the wild A. tropicus is an active predator presenting a functional response of a carnivorous fish (Montenegro-Guillen, Vammen, & Cisneros, 1991; Case, 1999; Jeschke, Kopp, & Tollrian, 2002; Mendoza et al., 2008).
The lineal relation between body size and gape size observed in A. tropicus, suggests that the inclusion of larger prey and prey switching occurs in relation with fish larval age (Murdoch & Bence 1987; Domínguez-Domínguez, Nandini, & Sarma, 2002). Even when we did not find differences among the three densities used in selectivity experiments, after the third week, selectivity for A. fransiscana shifted to M. macrocopa. This result suggests that the switching for prey species in A. tropicus, could be more related to behavioural changes during later larval stages of the fish larvae than to conspicuous prey size (Zaret, 1980). Also, the shift in prey selection in A. tropicus indicates that even at low prey availability, the carnivorous fish larvae are able to manipulate and feed on zooplankton of wide range in size, in contrast with herbivore fish (e.g. C. carpio), where a very high initial density of zooplanktonic prey is required (Khadka & Rao, 1986). Daphnia pulex was selected only in the 8th week, and had the ability to escape once captured. This could be associated to the presence of morphological defence structures (e.g. apical spines, helmets); in contrast, M. macrocopa lacks these structures, which makes this prey species easier to manipulate for the fish larvae (Dodson & Frey, 2001).
The ability of A. tropicus to manipulate prey at low densities is supported by the high capture success and high ratio of ingestion per capture, enhancing the larval survival. Our study indicates that A. tropicus larvae fed well on M. macrocopa and A. fransicana once the yolk was absorbed and until the first eight weeks of age. In general, rearing different freshwater larvae (e.g. fish, crustaceans) presents higher success in survivorship using a mix of zooplanktonic prey species (Gerking, 1994; Barros & Valenti, 2003; Martín, et al., 2006). For A. tropicusMárquez-Couturier et al. (2006) obtained the higher survivorship using a mix of D. pulex and A. franciscana, and the lowest survivorship using D. pulex as the only food source. Considering the nutritional quality of these prey species; the percentage for protein and lipids of Daphnia sp. is 30.80 - 61.00 % (Bogatova, Shcherbina, Ovinnikova, & Tagirova, 1971) and 11.90 - 12.10 % (Barata, Varo, Navarro, Arun & Porte, 2005), for Moina sp. 69.53 - 75.20 % and 9.94 - 11.51 % and, Artemia sp. 59.06 and 16.77 % (Alam, Ang, & Chea, 1993) respectively; therefore, we suggest that prey selection in A. tropicuscould be related to the content of protein in the prey; however, the evaluation of the relation of the optimal nutritional requirements and fish behavior and selectivity for fish larvae is still needed.
Larval feeding studies of both ornamental and consumable fish species are important for formulating successful management, and culture strategies for conservation purposes. The zooplankton species used in this study can be cultured in high densities (Bossuyt & Sorgeloos, 1979; Dodson & Frey, 2001), and regarding its protein/lipids content and our results, we suggest the use of a mix of A. franciscana and M. macrocopa to feed A. tropicus in culture systems in concentrations ≈ 2 ind. mL-1 during the first 3 weeks of age and then shift to M. macrocopa from the 4 week. The results obtained in this study can also be used in regional aquaculture for conservation efforts, enhancing this species growth at early stages and increasing the survivorship of adult and juvenile individual for further reintroduction or in situ management.
Directly, we did not test the survivorship of the larvae, however we used the three zooplankton species in routine feeding of the larvae and obtained 92 % of survivorship in the stocking density, suggesting that using a mix of these prey species can result in low fish larvae mortality. Our results, in conjunction with studies on the survivorship of the juveniles under natural conditions would aid in conservation efforts and improve the production of gars in aquaculture, mainly in North America, Central America and Cuba, where this species’ populations have declined and are used for human consumption.