Locusts are one of the most damaging pests worldwide. They undergo a typical gregarious phase characterized by an increase in population density and formation of swarms, and in turn moving and travelling hundreds or thousands of kilometers in search for suitable habitat, threatening agriculture and rural livelihood (Symmons & Cressman, 2001; Sword, Lecoq, & Simpson, 2010).
The Central American Locust, Schistocerca piceifrons piceifrons is a major plant pest in cropped and grazing areas in Southern Mexico and Central America (Harvey, 1983; Barrientos, Astacio, Álvarez, & Poot, 1992). In the Yucatán Península there is an important gregarious zone for S. p. piceifrons, where this species finds suitable vegetation and weather for breeding and growing (Magaña, Contreras & Álvarez, 2013; SENASICA-DGSV, 2016).
Plant community and weather characteristics have an influence on Orthoptera outbreaks and population maintenance (Bouaichi, Simpson, & Roessingh, 1996; Torrusio, Cigliano, & Wysiecki, 2002; Smith & Capinera, 2005). Vegetation plays a major role in providing food and shelter for locusts. Studies on the desert locust Schistocerca gregaria have shown that high population density is correlated with the abundance of a specific group of plants, like the Boraginaceae Heliotropium arbainense and the Poaceae Pennisetum typhodium, Aristida pungens and Panicum turgidum (Ould & Sword, 2004; Woldewahid, Van der Werf, Van Huis & Stein, 2004; Woldewahid et al., 2007; Sword et al., 2010). The stage of the vegetation has been also implicated in affecting locust biology, low cover and dry vegetation leading to a high probability of contact between individuals, triggering gregarization and swarm formation (Cisse et al., 2013).
Climate and soil characteristics play an important role on fluctuation of population density of various insect species (Vinatier, Tixier, Duyck, & Lescourret, 2011). In the case of locusts, temperature and soil moisture are highly related to egg laying and in turn to increases in locust population densities (Van der Werf, Woldewahid, Van Huis, Butrous, & Ykora, 2005; Xian-Lei, Xian-Hui, Hong-Fu, & Kang, 2007). Weather at the regional scale, particularly the distribution of precipitation, is also a critical factor that shapes the population density of locusts (Powell, Berg, Johnson & Warland, 2007; Wysiecki, Arturi, Torrusio, & Cigliano, 2011).
Understanding the influence of factors, such as vegetation, climate and soil, on locust population increase is critical not only for predicting outbreaks, but also for effective field surveys (Symmons, 1992; Van der Werf et al., 2005). In this regard, the present study aimed to characterize seasonal population fluctuation of S. p. piceifrons in a gregarious zone in the Yucatán Península, and to determine the association of population increase with the characteristics of vegetation, soil, and climate factors.
Materials and methods
Study site: The study was conducted in seven sites of the Yucatán Península, where S. p. piceifrons commonly occurs (see characteristics of each site in Table 1). The sites have been recognized as gregarious zones for S. p. piceifrons in Mexico (Barrientos et al., 1992). The Yucatán Península has a sub-humid tropical climate with three seasons during the year: the rainy season (July-October) is characterized by abundant precipitation (> 60 mm per month), with the highest precipitation (> 200 mm) in September; the north-winds season (November-February) has a moderate precipitation (< 60 mm per month) with brief rains or drizzles; and in the dry season (March-June) the precipitation is < 16 mm per month (Orellana, Islebe & Espadas, 2003; González-Moreno, Bordera, & Delfín-González, 2015). Vegetation is characterized by secondary plant communities produced by the abandonment of grazing livestock ranches. The survey was specifically directed toward sites where the presence of S. piceifrons has been documented by the locust campaign in Mexico (Magaña et al., 2013) (Table 1).
Table 1: Characteristics of the study sites where the S. p. piceifrons breeds and gregarize Yucatán Península, México (Orellana, Espadas & Nava, 2010; Bautista et al., 2011)
Site (Coordinate) | Weather factors | Edaphic conditions | ||||||||||||
Type | Max P (mm) | Max T (°C) | Isot (°C) | Isoh (mm) | Land use | Sand (%) | Silt (%) | Clay (%) | pH | OM (%) | AD (g cm-3) | FC (%) | PWP (%) | |
Maxcanu (20°56’09”N 89°95’77”W) | SHW 0 | 1 371 | 37.3 | 26.5 | 800 | NG | 28.32 | 58.22 | 13.45 | 7.43 | 6.95 | 0.92 | 44.92 | 24.8 |
Telchac Pueblo (21°25’28”N 89°26’76”W) | SHW0 | 526 | 36.11 | 25.7 | 900 | NG | 38.50 | 45.70 | 15.67 | 7.80 | 18.50 | 0.69 | 59.02 | 44.84 |
Dzilam Gonzalez (21°24’66”N 88°94’53”W) | SHW0 | 209.8 | 35.76 | 25.7 | 900 | MG | 27.88 | 55.77 | 16.34 | 7.54 | 17.04 | 0.76 | 59.12 | 38.93 |
Panaba (21°43’08”N 88°39’11”W) | SHW1 | 1 313 | 34.80 | 25.5 | 1000 | SG | 30.99 | 51.77 | 17.23 | 7.87 | 21.24 | 0.69 | 63.25 | 44.95 |
Tizimin (21°36’25”N 88°07’96”W) | SHW1 | 1 555 | 36.36 | 26.0 | 1100 | HG | 22.76 | 60.88 | 17.45 | 7.61 | 15.67 | 0.72 | 58.53 | 38.68 |
Cenotillo (21°12’37”N 88°59’03”W) | SHW1 | 1 423 | 35.96 | 25.7 | 1200 | MG | 26.76 | 55.77 | 17.45 | 7.25 | 10.05 | 0.82 | 48.47 | 36.56 |
Tunkas (20°97’47”N 88°74’09”W) | SHW1 | 1 696 | 37.2 | 25.7 | 1200 | MG | 27.88 | 55.77 | 16.34 | 7.54 | 17.04 | 0.76 | 59.12 | 38.93 |
Weather type (SHW0 = Sub-humid warm - AW0, annual precipitation lowest 10% in summer; SHW1 = sub humid warm - AW1, annual precipitation highest 10% in summer; Max P = maximum precipitation; Maximum T = maximum temperature; Isot = Isotherm, Isoh = Isohyetal); Land use (NG = Non grazing, MG = Moderate grazing, SG = Slight grazing, HG = Heavy grazing); Edaphic conditions (OM = Organic Matter, AD = Apparent Density, FC = Field Capacity, PWP = Permanent Wilting Point).
Temperature, precipitation and evaporation of each locality were obtained from the database of the Water National Commission in Yucatán, México (http://www.conagua.gob.mx).
Locust and vegetation sampling: Population density of S. p. piceifrons and vegetation were sampled throughout a year in three different seasons: north-wind season (December-2013), dry-season (April-2014) and rainy-season (June-2014).
To quantify population density of S. p. piceifrons, the random sampling method was used: We counted the number of locusts that flew up in a standard one meter strip, while we walked along 100 m transect (Cressman, 2001), previously calibration of one meter by each step of sampler was done (Cressman & Dobson, 2001).
Nine transects of 100 m length × 1 m width on each site were established. Transects were separated by at least 100 m. Transects were walked by evaluators and the number of locusts that fled the area were counted. Sampling was carried out early in the morning (7:00-10:00 h). The identification of S. p. piceifrons was by morphological characteristics of Contreras and Magaña (2013).
Vegetation composition was sampled in quadrats of 4 x 4 m distributed along transects where S. p. piceifrons was sampled. Ninety quadrats per site were sampled. To describe vegetation diversity two variables were calculated: plant species richness (PSR) and relative species density (RSD), as described by Capitanio and Carcaillet (2008). The species list was not intended to be an exhaustive account of all plants present in the study area, but to satisfactorily represent the flora of the sites.
Determination of edaphic conditions: Soil samples were obtained at 10 cm depth into a 4 × 6 m2. Six samples were taken in each study site. Determination of soil characteristics included: texture, pH, organic matter content, apparent density, field capacity and permanent wilting point. Land use in the sites was classified as non-grazing, slight grazing, moderate grazing and heavy grazing (Table 1) (Van der Werf et al., 2005).
Locust density was analyzed among study sites and seasons. For such purposes an analysis of variance was done and post hoc pairwise mean comparison by Scott & Knott (1974) was performed when significant differences (P < 0.05) were observed. The analysis was performed in InfoStat software (Di Rienzo et al., 2014).
Data on plant species richness (PSR) and relative species density (RSD) were analyzed by generalized linear models (McCullagh & Nelder, 1989). Both variables were analyzed under two-factors repeated measures, factor 1 included sites (seven levels) and factor 2 included seasons (three levels). All plant species were individually analyzed with a Poisson probabilistic model and a Log link function. Differences among levels of both factors were analyzed by least significant difference (LSD, < 0.05). A Wald statistical test, using the maximum likelihood method, was used to evaluate the effects of covariance (sites and seasons) on PSR and RSD. The statistical analysis was run in SPSS 22 for Windows.
To analyze the relationship of RSD, isotherms/isohyets and climate type vs locust population density, a combination of multiple factor analysis (MFA) and principal component analysis (PCA) were used. A second MFA was run separately to test the relationship of RSD, edaphic conditions and land use with locust population density. This second MFA was carried out using the locust population density during the rainy season due to the higher values observed for this variable in that period. The MFA is a multivariate ordination method that permits examination of common structures in datasets with variables that can be separated into different groups (Escofier & Pagès, 1998). MFA involves two steps. First, a principal component analysis (PCA) is performed on each group of variables. Second, the normalized datasets are merged to form a unique matrix and a global PCA is performed. After MFA and PCA analysis, two statistical tests for correlation coefficient were used: RV among groups (Josse et al., 2008). This method scales from 0 to 1. In 0 every variable in one group is completely uncorrelated with every variable in the other group(s), and in 1 the two groups are completely homothetic (Borcard, Guillet & Legendre, 2011; Pagès, 2015). The LG coefficient reflects the MFA normalization and takes positive values (Abdi, Williams, & Valentin, 2013). The analysis was run in R Software V.2.15.3 (R Core Team 2013) using the FactorMine package (Lë, Josse, & Husson, 2008).
To test the relationship between locust density and RSD, a principal component analysis (PCA) was performed with each plant species. For such a purpose, a varimax rotation was used and with principal components produced (PCs), predictive models with multiple regressions were established. The PCs were produced as independent variables and locust density as dependent variables. The statistical analysis was run in SPSS 22 for Windows (IBM, 2013).
Results
Seasonal population fluctuation of Schistocerca piceifrons piceifrons: The density of S. p. piceifrons was significantly higher in the sites Tunkas, Panaba, Tizimin, Cenotillo and Dzilam González relative to that of Maxcanu and Telchac Pueblo (F = 74.3, P < 0.0001). In general, S. p. piceifrons density was higher during the rainy-season compared to that in north-wind or dry-seasons (F = 50.4, P < 0.0001) (Figure 1).
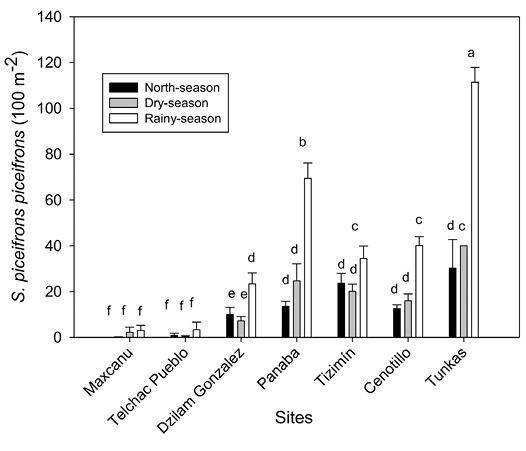
Figure 1 Population fluctuation of S. p. piceifrons in seven sites of the Yucatán Península, Mexico. Bars (mean ± SE). Different letter indicate statistical significant differences (Scott-Knott test, P < 0.05).
Characterization of vegetation diversity: Vegetation diversity was characterized by two variables: plant species richness (PSR) and relative species density (RSD). The most representative plant community included 17 plant species, among them herbs, shrubs, trees and palm trees. Herbs included Panicum maximum, Cynodon nlemfuensis and Brachiaria brizantha, and secondary vegetation composed of trees, shrubs and palm species such as Pisonia aculeata, Guazuma ulmifolia, Leucaena leucocephala, Sabal yapa, Piscidia piscipula, Waltheria americana, Chamaecrista glandulosa, Viguiera dentata, Sida acuta, Panicum ghiesbreghtii, Amaranthus sp., Partenium hysterophorus, Tagetes sp. and Desmodium sp.
Plant species richness (PSR) was significantly different between sites and seasons (χ2 Wald= 17.4, P < 0.001; and χ2 Wald= 23.6, P < 0.0001, respectively; Figure 2). There was a significant difference in PSR among the interaction of these factors (χ2 Wald= 50.9, P < 0.0001).
PSR was higher in Dzilam González (7.3, SE= 0.52) and lower in Maxcanu (4.9, SE= 0.19). Likewise, PSR was higher during the rainy season (7.0, SE= 0.33) than in the north-wind season (6.2, SE= 0.31) and dry season (4.9, SE= 0.28; Figure 2).
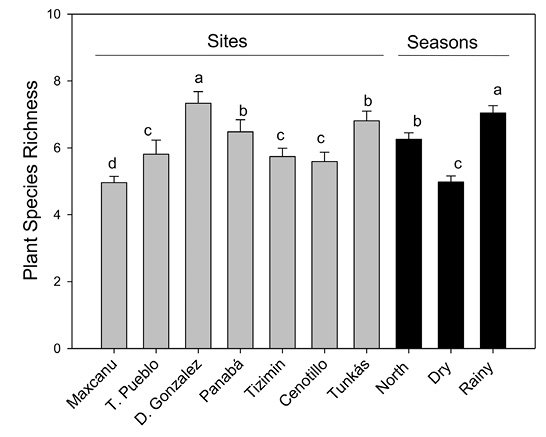
Figure 2 Plant species richness (PSR) among sites of the Yucatán Península, Mexico, and seasons. Bars (mean ± SE). Different letter indicate statistical significant differences (LSD test, P < 0.05).
RSD was significantly different between sites, seasons, and the interaction sites × seasons for six plant species, P. aculeata, P. cuminis, C. glandulosa, P. ghiesbreghtii, Tagetes sp. and Desmonium sp. The other plant species only showed significant differences between sites and/or seasons, except for S. yapa, which showed no differences between sites or seasons (Table 2).
Table 2: Generalized linear model for relative species density (RSD) among sites of the Yucatán Península, Mexico, seasons, and sites × seasons. The Wald χ2 and their significance are shown for each plant species
Plant species | Site | Season | Site × Season |
Pisonia aculeata | 50.5*** | 6.3* | 79.4*** |
Guazuma ulmifolia | 6.6 | 5.2 | 23.0* |
Leucaena leucocephala | 157.5*** | 8.4* | 19.6 |
Sabal yapa | 5.4 | 2.5 | 16.4 |
Piscidia cuminis | 88.0*** | 26.7*** | 38.5*** |
Walteria americana | 77.3*** | 4.3 | 13.5 |
Chamaecrista glandulosa | 255.7*** | 105.0*** | 177.4*** |
Viguiera dentata | 48.9*** | 0.3 | 8.7 |
Sida acuta | 25.0*** | 3.2 | 28.0** |
Panicum ghiesbreghtii | 17.0** | 41.6*** | 21.4* |
Amaranthus sp. | 30.9*** | 10.2 | 12.1 |
Parthenium hysterophorus | 23.9** | 0.3 | 8.1 |
Tagetes sp. | 106.9*** | 11.3** | 39.3*** |
Desmodium sp. | 24.2*** | 17.7*** | 34.1** |
Panicum maximum | 185.2*** | 4.6 | 2.3 |
Cynodon nlemfuensis | 113.6*** | 3.7 | 5.3 |
Brachiaria brizantha | 167.4*** | 0.5 | 3.2 |
*= P < 0.05; ** = P < 0.001; *** = P < 0.0001.
RSD varied significantly between sites. The highest RSD values for Amaranthus sp. (2.3, SE= 0.51), P. hysterophorus (2.3, SE= 1.11), C. nlemfuensis (30.7, SE= 6.78) and P. ghiesbreghtii (6.3, SE= 2.0) were observed in Panaba; for L. leucocephala and V. dentata in Maxcanu (15.2, SE = 2.64 and, 17.3, SE = 4.89, respectively); for G. ulmifolia and W. americana in Telchac Pueblo (0.1, SE= 0.07 and, 24.2, SE= 3.31, respectively); for P. aculeata and B. brizantha in Dzilam González (1.3, SE= 0.41 and, 29.9, SE= 5.81, respectively); for P. maximum in Tizimin (65.4, SE = 3.74); for Tagetes sp. and Desmodium sp. in Cenotillo (14.4, SE = 3.5 and, 3.6, SE = 2.29, respectively); for C. glandulosa and S. acuta in Tunkas (39.2, SE = 6.1 and, 36.1, SE = 6.78, respectively); and for S. cuminis in Maxcanu and Dzilam González (1.9, SE = 0.42 and, 1.5, SE = 0.29, respectively).
RSD also showed variation between seasons. The highest RSD for Tagetes sp. (4.3, SE = 0.31), Desmodium sp. (3.0, SE = 0.11), P. aculeata (0.5, SE = 0.02), P. cuminis (1.2, SE = 0.10) and L. leucocephala (3.4, SE= 0.013) was observed in the rainy season; whereas the highest RSD for C. glandulosa (10.4, SE = 1.50 and, 9.2, SE= 1.39), V. dentata (3.3, SE= 0.77 and, 4.2, SE = 1), P. ghiesbreghtii (3.6, SE = 0.76 and,= 0.52) was observed in the north-wind season and rainy season, respectively. No difference in RSD between seasons was observed for the rest of the plant species.
Association between locust density and vegetation diversity, weather factors and edaphic conditions: The first MFA showed that the first two axes explained almost 42 % of the total variance. Matrices obtained from MFA were no orthogonal (Lg and Rv coefficients in Table 3), which indicates no proximity among groups. In this sense, RSD was identified as the most important, followed by isotherm/isohyets and Max. P/T. No correlation between locust density with other groups was found (Table 3).
Table 3: Multiple Factor Analysis (MFA) for the groups of locust density, RSD and weather factors of the Yucatán Península, Mexico; Lg and Rv coefficients are shown
MFA over annual data | |||||||
Rv Lg | Weather factors | RSD | Locust density | MFA | |||
Type | Max P/T | Isoth/Isohy | |||||
Weather factors | Type | 0.17 | 0.59 | 0.34 | 0.34 | 0.49 | |
Max. P/T | 0.20 | 0.13 | 0.27 | 0.33 | 0.60 | ||
Isoth/Isohy | 0.63 | 0.16 | 0.38 | 0.28 | 0.63 | ||
RSD | 0.66 | 0.62 | 0.77 | 0.39 | 0.86 | ||
Locust density | 0.34 | 0.39 | 0.30 | 0.74 | 0.47 | ||
MFA | 0.78 | 1.13 | 1.08 | 2.62 | 0.75 | ||
MFA over rainy season data | |||||||
Rv Lg | Land use | Soil | RSD | Locust density | MFA | ||
Land use | 0.35 | 0.73 | 0.29 | 0.65 | |||
Soil | 0.66 | 0.44 | 0.14 | 0.38 | |||
RSD | 1.98 | 0.73 | 0.43 | 0.91 | |||
Locust density | 0.51 | 0.15 | 0.67 | 0.76 | |||
MFA | 1.45 | 0.52 | 1.82 | 0.98 |
The second MFA analysis showed that the first two axes explained 58.4 % of the variance. Matrices from MFA were not orthogonal (Lg and Rv coefficients in Table 3). Similar to the first MFA, the second MFA showed RSD as the most important group. In this second MFA, locust density and land use were also considered important groups. No correlation between locust density with other groups was found (Table 3). RSD as the most important group in both MFA was used to analyze the principal component analysis (PCA) of the association between RSD and locust density. RSD showed that six principal components (PCs) explained 83.2 % of the total variation; almost half of this variation was explained by first three components, PC1 (15.3 %), PC2 (14.9 %), and PC3 (14.3 %). The rest of the components (PC4-PC6) explained 38.4 % of the total variation (Table 4). When analyzed, the degree of dependence of locust density as a result of PCs, only PC3 and PC5 were statistically significant. Population density of locusts and PC3 showed a partial negative correlation (Sr 2 = -0.62). PC3 was mainly influenced by L. leucocephala, which suggests that the population density was lower where the abundance of L. leucocephala was higher. In contrast, the population density and PC5 showed a partial positive correlation (Sr 2 = 0.85). PC5 was influenced mainly by P. maximum, which indicates that S. piceifrons density was higher as abundance of P. maximum increased.
Table 4: Principal Component Analysis of RSD of the Yucatán Península, Mexico. The table shows the weight of the variables for each component after the rotation varimax
PC1 | PC2 | PC3 | PC4 | PC5 | PC6 | |
Explained variance | ||||||
Eigenvalue | 2.6 | 2.5 | 2.4 | 2.3 | 2.1 | 2.0 |
Variance (%) | 15.3 | 14.9 | 14.3 | 13.5 | 12.9 | 12.0 |
Cummulative variance | 15.3 | 30.3 | 44.7 | 58.3 | 71.2 | 83.2 |
Plant species | ||||||
P. aculeata | -0.54 | 0.88 | -0.12 | -0.03 | 0.01 | 0.07 |
G. ulmifolia | 0.83 | -0.14 | -0.24 | 0.03 | -0.33 | -0.03 |
L. leucocephala | 0.04 | -0.13 | -0.90 | 0.10 | -0.12 | -0.13 |
S. yapa | 0.01 | 0.08 | 0.35 | 0.82 | -0.07 | -0.01 |
P. piscipula | 0.75 | 0.47 | 0.15 | -0.11 | -0.01 | -0.07 |
W. americana | -0.01 | 0.50 | 0.10 | -0.23 | 0.20 | 0.75 |
C. glandulosa | 0.79 | -0.03 | 0.23 | -0.08 | 0.45 | -0.11 |
V. dentata | 0.03 | -0.19 | 0.67 | -0.17 | 0.07 | -0.15 |
S. acuta | 0.78 | -0.21 | 0.10 | -0.03 | 0.39 | 0.17 |
P. ghiesbreghtii | 0.19 | -0.01 | -0.08 | -0.08 | 0.16 | 0.05 |
Amaranthus sp. | -0.03 | -0.11 | -0.22 | 0.51 | -0.75 | 0.25 |
P. hysterophorus | -0.06 | -0.11 | -0.14 | 0.91 | 0.04 | -0.02 |
Tagetes sp. | -0.13 | -0.01 | -0.09 | -0.09 | 0.34 | 0.81 |
Desmodium sp. | 0.15 | -0.24 | -0.14 | 0.13 | -0.39 | 0.69 |
P. maximum | -0.04 | -0.43 | -0.73 | -0.10 | 0.87 | -0.28 |
C. nlemfuensis | -0.12 | -0.16 | -0.45 | 0.58 | 0.05 | -0.25 |
B. brizantha | 0.01 | 0.92 | -0.01 | -0.06 | -0.08 | -0.06 |
Discussion
In the present work we have determined the seasonal population fluctuation of the S. p. piceifrons and its associations with vegetation diversity in a gregarious zone of the Yucatán Península, Mexico. We observed that within the gregarious zone, population density of S. p. piceifrons varied between sites and seasons. In most of the sampling sites the highest locust population density was observed in the rainy season, in contrast; a dramatic decrease in population density was observed in the dry season. The reduction in population density of S. p. piceifrons in the dry season is attributed to a decline in the abundance of host plants and to changes in locust physiology that produce a recession period (Barrientos et al., 1992; Symmons & Cressman, 2001; Hernández-Zul et al., 2013). These responses fit a seasonal pattern established for some herbivorous insects whereby diapause during the dry season maximizes their survival (Wolda, 1989; Kishimoto-Yamada & Itioka, 2015).
The analysis of the vegetation diversity showed that PSR and RSD were different between sites and seasons. The highest values for PSR and RSD were observed in the rainy season. We ran two MFA’s to find important groups (weather factors, RSD, land use, soil characteristic and locust density) and possible associations among them, particularly with the locust population density. MFA is a multivariate technique that reduces the number of variables arranged in groups and uncover correlations between the different groups analyzed through Rv and Lg coefficients. We found that in both MFA’s the most important group was RSD. We found no correlation of locust density with weather factors, land use or soil characteristics. Interestingly, when a PCA was run with the plant species included in the RSD group, we uncovered an association of locust population density with some plant species. We found that locust population density showed a positive correlation particularly with the abundance of P. maximum. Abundance of this grass species has been also associated with high population density of the S. gregaria locust (Woldewahid et al., 2007). The positive effect of the abundance of P. maximum on population density of locust species may be related to two main factors: 1) P. maximum represents a good source of food not only in the rainy season, but also in the dry season due to its drought tolerance capacity (Ho, Tsai, Huang, & Kao, 2016) due to its efficient water use (C-4 grass species) (Ghannoum, 2009), 2) P. maximum creates a suitable environment for locust development due to plant architecture (leaf abundance and root volume) which permits secondary vegetation to grow. Secondary vegetation like the shrubs G. ulmifolia and S. yapa, and the annual herbs W. americana, S. acuta, P. hysterophorus and Amaranthus sp. serve as food source for S. p. piceifrons (Avilés & Ayala, 1994; Culmsee, 2002; Kokanova, 2014; Poot-Pech, Ruiz-Sánchez, Ballina-Gómez, Gamboa-Angulo, & Reyes-Ramírez, 2016). We suggest that the association between P. maximum and plant species comprising the secondary vegetation creates microclimates that produce a suitable environment for S. p. piceifrons even in the dry season (Ruttan, Filazzola, & Lortie, 2016). In addition, we also suggest that P. maximum is a grass species that may benefit defensive behavior of S. p. piceifrons against natural enemies, similar to that of the desert locust S. gregaria. The desert locust has the ability to change defensive behavior according to the condition of vegetation, shelter plant size and abundance is critical to hiding from predators or escaping quickly by moving among plants (Maeno et al., 2013).
Other studies have found that environmental conditions, including weather factors and edaphic conditions, play an important role in locust population densities (Bouaichi et al., 1996; Rong, Bao, Dian, Zhe, & Xian, 2007). Surprisingly, we found no strong association between weather factors or edaphic conditions with locust density. The weather in the Yucatán Península is fairly homogeneous, especially in the Northern region, where our study sites were located (Orellana et al., 2003). In contrast, although Contreras, Galindo and Ibarra (2013) have mentioned an association of the locust survey with Aw0 clime in Yucatán Península, this only highlight the activity of the locust (due to the monitoring) and not necessarily where the pest is present in a high density. In addition, we would have to consider that the Aw1 clime where we found the highest locust density, is an intermediate clime warm sub-humid between Aw0 and Aw1 (based in the precipitation and temperature) (Köppen, modified by García, 1998). Soils in the Yucatán Península, where S. p. piceifrons breeds and grows, are clay-rich, slightly acid and not very fertile (Bautista, Palacio, Quintana, & Alfred, 2011). All these types of soils sustain grassy vegetation suitable for the development of S. p. piceifrons populations.
In conclusion, population density of S. p. piceifrons was higher in the rainy season in all sites. High plant species richness and abundance were found in the rainy and north-wind seasons. Population density of S. p. piceifrons was not associated with RSD, weather factors, land use or edaphic conditions. A high positive correlation was found between locust population density and abundance of the grass P. maximum.