Services on Demand
Journal
Article
Indicators
-
Cited by SciELO
-
Access statistics
Related links
-
Similars in SciELO
uBio
Share
Revista de Biología Tropical
On-line version ISSN 0034-7744Print version ISSN 0034-7744
Rev. biol. trop vol.62 n.2 San José Apr./Jun. 2014
Ten years of limnological monitoring of a modified natural lake in the tropics: Cote Lake, Costa Rica
Diez años de monitoreo limnológico de un lago natural modificado en los trópicos: el lago Cote, Costa Rica
Diez años de monitoreo limnológico de un lago natural modificado en los trópicos: el lago Cote, Costa Rica
*Dirección para correspondencia:
Abstract
It is located 650m above sea level along the boundary between the North Caribbean and Pacific slopes, near the Southern end of the volcanic Guanacaste mountain range. In the early 1980s the lake’s main outlet was dammed and the outflow was diverted into Arenal Reservoir. Lake Cote was first studied in 1990-1991, and later in 2001, before it was again modified by raising its dam by one meter to use its outflow for hydroelectricity. From 2002 to 2010 it has been monitored twice a year for changes in its limnology. Here I present a summary of its basic characteristics and an analysis of their changes through time. The lake is discontinuous polymictic, and sometimes develops a thermocline at 6m depth that may last for several days as evidenced by the occasional development of an anoxic layer close to the bottom. Since its modification for hydropower production, the surface water temperature has attained higher values than before. Oxygen levels in the lake show periods of hypoxia to anoxia in the hypolimnion, that have become more frequent since modification. Despite its turbid water, the lake has low levels of nutrient concentrations and of chlorophyll a. The trend in these parameters in recent times is a reduction in chlorophyll a and an increase in water transparency, implying a reduction in primary productivity. These changes are discussed in relationship with anthropogenic factors such as the modification of the lake and its management, changes in landscape around the lake and global climate change. Rev. Biol. Trop. 62 (2): 567-578. Epub 2014 June 01.
Key words: Lake Cote, limnology, ten-year monitoring, tropical lake, hydroelectricity, level modification, Costa Rica, neotropics.
Resumen
El lago Cote es el más grande de Costa Rica Esta localizado a 650m sobre el nivel del mar en el límite entre las llanuras del Norte y la vertiente del Pacífico en la Cordillera de Guanacaste, Costa Rica. Al inicio de la década de 1980 se construyó una represa en el río de desagüe y el caudal de salida fue desviado hacia el Embalse Arenal. Se realizó un primer estudio entre 1990 y 1991, y luego en el año 2001 antes de que se construyera una nueva modificación del lago mediante el levantamiento del nivel de la presa en un metro con el fin de utilizar la descarga para la producción de energía hidroeléctrica. Del 2002 al 2010 se realizó un monitoreo limnológico dos veces al año. Aquí se presenta un análisis de las principales características y de los cambios a través del tiempo. El lago es polimíctico discontinuo y desarrolla una termoclina intermitente a 6m de profundidad, la cual puede perdurar por varios días o semanas a juzgar por el desarrollo de una capa anóxica cercana al fondo. Desde su modificación la temperatura superficial del lago ha alcanzado valores mayores a los reportados con anterioridad. El hipolimnion muestra periodos de hipoxia a anoxia que se han vuelto más frecuentes. A pesar de la turbidez del lago, la concentración de nutrientes y de clorofila a son bajos. La tendencia en tiempos recientes es a una reducción de la clorofila a y un aumento en la trasparencia del agua. Estos cambios son similares a los observados en lagos donde se ha logrado atribuirlos al cambio climático global. También se pueden deber a la modificación del flujo del efluente por la operación intermitente de la planta hidroeléctrica, que favorece la acumulación de calor en la columna de agua. Esto debido a que otros factores, como el cambio en el uso del suelo en los alrededores del lago ocurrieron en la década de 1980, por lo que no se les puede atribuir una influencia en los cambios observados en los últimos 10 años.
Palabras clave: Lago Cote, limnología, monitoreo-diez años, lago tropical, hidroelectricidad, nivel de modificación, Costa Rica, neotrópico.
Lakes around the world are a valuable source of fresh water and provide several services for humankind, from sites for recreation to sources of protein (Dungan, 1992). As a result, lakes have been exposed to the impact of human activities, including eutrophication, altered pH from acid rain and introduced exotic species, with diverse ecological impacts (Kalff, 2002). One further modification is the transformation of natural lakes into regulated reservoirs after construction of a dam at its main outlet. Usually reservoirs created for hydroelectricity are subject to water level fluctuations according to their intended use and the availability of the water replenishing the reservoir (Ford, 1990; Kalff, 2002; Roldán Pérez & Ramírez Restrepo, 2008). Small reservoirs are usually managed to meet peak demands of electricity during the day, and the water level fluctuates on a daily basis, while big reservoirs are used to store surplus water supply during the rainy season, for use during drier periods of the year and have a fluctuation that is more seasonal (Kalff, 2002; World Comission on Dams, 2000). These fluctuations have an impact on the reservoir ecology, since the shore is intermittently exposed and flooded on a regular basis. Further, the withdrawal of the water also has an impact on the reservoir’s limnology, especially the depth of the outlet. If the outlet is deep, it affects mainly the hypolimnion and thermocline that usually develops in the reservoir near the dam (Roldán Pérez & Ramírez Restrepo, 2008; Thornton, Kimmel, & Payne, 1990). If the outlet is more superficial it withdraws mainly surface water from the reservoir (Roldán Pérez & Ramírez Restrepo, 2008). The increasing need for sources of freshwater in the world makes it necessary to understand the ecology of freshwater aquatic ecosystems in order to provide the basic knowledge needed for sound sustainable use.
In Costa Rica natural lakes have been far from the main development centers of the country (Umaña, Haberyan, & Horn, 1999), and thus most of these environmental problems have not occurred or have not been detected. Lake Cote is one of these little known lakes, although it is the natural lake with the largest surface area in the country (Umaña et al., 1999). Nevertheless it is now receiving greater interest due to increasing tourist development in the vicinity of the lake. In the early 1980s a dam was constructed at its main outlet and a tunnel diverted the outflow into Arenal Reservoir. The dam was further modified in 2002 to take advantage of the lake for hydropower production. The modification included raising the water level by one meter, which flooded some areas around the lake. In addition, the water level is now subject to fluctuations according to the needs of power production. This modification also includes the management of the water stored for hydropower production, which impose a periodic withdrawal of water at daily periods of peak electricity demand. Nevertheless in the case of Lake Cote, the water level fluctuation is mainly seasonal; with small daily variations (CNFL unpublished data). The outlet is superficial since it is not a constructed reservoir but rather the modification of a natural lake. It can be expected that the lake would have changed its normal behavior due to the modification of its hydrologic dynamics.
The present paper summarizes the results of ten years of a biannual monitoring limnological study of Lake Cote before and after its modification for hydropower production by the CNFL, and compares them with limnological data on the lake, from a previous study in 1990-1991 when the lake was sampled every three months. The monitoring has given the opportunity to collect data on the thermal situation of the lake twice a year for more than ten years of observation, and as such, it also allows for the examination of any effect of the global climate change on this neotropical lake. An analysis for the region has revealed a warming trend in the past decades (Aguilar et al., 2005) and Lake Cote could reveal some signs of these effects. According to the literature it would be possible to observe changes such as an increase in the intensity of the stratification, or a shallower thermocline, and a resulting decrease in productivity (Hambright, Gophen, & Serruya, 1994; Hecky et al., 1994; Plisnier, 2000; verburg, Hecky, & Kling, 2003).
Materials and methods
Lake Cote is located in the Southeast end of the volcanic Ridge of Guanacaste, Costa Rica (between 10°34’25” a 10°35’29” N-84°54’15” a 84°55’13” W). It formerly had a surface area of 198.3hm2 and a maximum depth of 11m (Haberyan, Horn, & Umaña, 2003). The modifications have increased its surface area to 216.1hm2 and depth to 12m, according to data from the Compañía Nacional de Fuerza y Luz (CNFL unpublished data), owner of Cote Hydroelectrical Plant. It has been visited and its basic limnological characteristics have been studied on different occasions since 1990.
During 1990 and 1991 it was visited every three months (August, November, February and June). It was visited again in November 2001, and from 2002 to 2010 it was visited twice per year, in the dry (January to June) and wet season (July to December). Actual visit dates varied from year to year due to logistic limitations. Occasionally additional visits were made as part of a field trip of the limnology course at the University of Costa Rica. A sampling station was established in 1990 near the center of the lake (10°34’45” N - 84°54’56” W). Temperature and dissolved oxygen profiles were determined with an electronic device (YSI Model 51) which was regularly calibrated before each sampling trip. Water samples were taken at the same station every 2m of depth by means of an Alpha bottle, for the determination of chlorophyll a concentration, pH, and conductivity, and at three depths for the determination of nutrients (nitrate, nitrite, ammonium and orthophosphate). Samples were transported in cold storage to the laboratory at the Research Center for Marine Sciences and Limnology (CIMAR) of the Universidad de Costa Rica (UCR).
Samples for chlorophyll a were filtered onto GF/C glass fiber filters in the field with a portable filtration unit, and filters were kept at 4°C for transportation to the laboratory. Chlorophyll a was determined following the SCOR/UNESCO methodology (Parsons et al., 1984). Samples were analyzed at the laboratory following procedures established by Stricklan & Parsons (1984). Chlorophyll a and nutrient values were integrated for the whole water column and values are given per surface unit.
Linear model analyses were performed with R (R Development Cote Team, 2011) to examine the trend of some variables with time. A principal component analysis was performed to examine the general relationships among the main variables using PAST (Hammer, Harper & Ryan, 2001), after a selection of variables according to the pairwise correlations. Variables selected for the Principal Components Analysis (PCA) were surface temperature (Tsup), difference in temperature between surface and bottom (DeltaT), dissolved oxygen at the surface (Oxsup), difference in dissolved oxygen between surface and bottom (DeltaOD), depth of mixing (Zmix), Secchi depth (Secchi), surface Chlorophyll a (Chla), nitrate (NO3) and phosphate (PO4).
Results
Water column: Secchi depth fluctuated between 1.06 and 4.32m (Fig. 1). The lowest values were observed in June 1991, May 2002, October 2008 and June 2009. In June 1991 the low value was related to heavy rains at the time. Low values in 2002 and 2003 occurred during construction of the modification of the dam to increase lake volume and raise the level by 1m, but the lake returned to greater water transparency in late 2003 and the Secchi depth remained near 2m. The low values in late 2008 and June 2009 were related to heavy rain storms, which resulted in higher than normal inputs of suspended matter due to erosion in the catchment basin. There was however, a slight tendency of increased water transparency with time, with higher values in recent years, especially after 2007. A linear model analysis yielded a significant effect through time (F1,26=8.90, p=0.006).
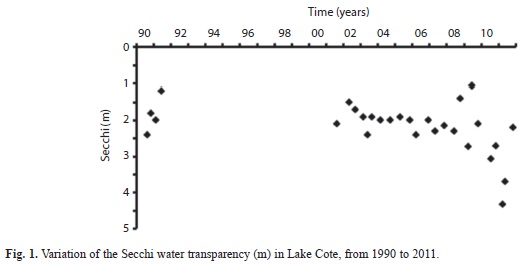
Surface water temperature fluctuated between 21.9 and 27.9°C, with a mean of 25.1°C. Using a linear model analysis, a slight but significant tendency of increased surface temperature with time was observed (F1,27=5.99, p=0.022). At 12m depth the range was between 20.9 and 24.9°C, with a mean of 23.3°C (Fig. 2). In this case, no tendency of temperature increase with time was identified (F1,27=1.20, p=0.28).The lake has been known to stratify since the 1990-1991 samplings, with a weak thermocline developing between 5 and 7m depth marked by an abrupt but small temperature decline (0.5-1.6°C). The highest temperatures at the surface were recorded in September 2004, July 2010, and May 2011. These warming events extended to a depth of 2m on the first date and up to 3m on the latter two dates (Fig. 3C). The lake has been homeothermal on several occasions, usually between November and February, but it has also been observed to be completely homeothermal in August 2001 and May 2002.
The surface concentration of dissolved oxygen varied between 9.4 and 7.06mgO2/L, whereas at the bottom the concentration varied between 7.4 and 0.0mgO2/L. Maximum oxygen levels were observed only before 2003. There has usually been a gradient in oxygen concentration (Fig. 4), though on some occasions the whole water column was completely oxygenated; these times were usually coincident with homeothermal conditions. Though fluctuations in deep water oxygenation were observed, no consistent tendencies were evidenced by the data.
The conductivity of the water in Lake Cote has been low for the whole period of observation, with a maximum of 168μSi/cm in June 1991 at the bottom, and a minimum of 17μSi/ cm in November 2006 at the surface. The mean conductivity was 35.9μSi/cm. Usually, larger values occurred at the bottom, although conductivity did not show a marked stratification in the lake. Minimum values were observed during the rainy season.
3.2 Nutrients and Chlorophyll a: Through the study period the concentration of nutrients varied considerably. Nitrate fluctuated from not detectable levels up to more than 11.0mM/m2 (Table 1). Ammonia reached higher maxima, up to 42.4mM/m2, but remained near non-detectable levels most of the time. Nitrite was always low; below 0.3mM/m2. On the other hand the soluble reactive phosphate reached concentrations higher than 2.2mM/m2 on two occasions, and remained below 0.8mM/m2 for most of the samplings. The variation did not show a distinct dry/wet seasonal pattern.
The Chlorophyll a concentration at the surface of the lake varied between 1.33 and 14.76mg/m3, with a mean of 4.86±3.07mg/m3 (Fig. 5). Maximum values were observed in August 2001 and May 2002. The integrated water column content of Chlorophyll a varied between 25.8 and 95.7mg/m2, with a mean of 57.3±22.1mg/m2. The integrated values were higher than average in 2001, in the years 2004 through 2006, and then again in November 2007. Nevertheless, there is a decreasing trend of Chlorophyll a with time in the last 10 years of sampling for which data are available: Surface Chl. a (F1,23=4.25, p=0.05*), Integrated Chl. a (F1,19=12.6, p=0.002**).
The first axis in the PCA analysis was positively related to both surface temperature and the difference in oxygen with depth in the water column (Fig. 5). Mixing depth was negatively related to the first axis, and so is also inversely related to surface temperature. All these variables determine the physical conditions in the lake, and explained 37% of the variance. The second axis was positively related with Chlorophyll a, nitrate, and dissolved oxygen, which is the result of photosynthesis. Secchi depth was negatively related with the second axis and so it is inversely related with Chlorophyll a. This second axis is more associated with variables that describe the trophic state of the lake, and explained 21.3% of the variance.
Discussion
During the 1990-1991 period of study, the lake was weakly stratified on the four occasions on which it was sampled, with a small thermal gradient at 6m depth. The water column was well oxygenated to a depth of 10m on all occasions; an increase in conductivity was detected only below 10m depth, near the bottom. During this time, the lake appeared to be discontinuous polymictic, despite being deeper than 10m, mainly because it is relatively shallow for its surface area (Relative depth was 0.69 during 1990-1991). In addition, the lake is located in a region of high winds, higher than 15Km/h throughout the year (Muñoz, Fernández, Gutiérrez, & Zárate, 2002), which also influences its water column dynamics. In the period between 2001 and 2011 the lake also showed periods of stratification at about the same depth, and a few occasions when it was homeothermal. Stratification was more common between March and October (Fig. 6), which correspond to the period of the year with lower winds in Costa Rica (Muñoz et al. 2002). In this respect, the behavior of the lake has not changed, even after its modification. However, the observed temperatures reached higher maxima in recent years than a decade before (maximum of 25.5°C), and temperatures have not fallen below 22°C as occurred in February 1991.
The observed increase of the higher surface temperatures could be the result of two factors. One is the short term storage of surface water for later use for hydropower during daily production peaks. This water storage can produce a net accumulation of heat in surface waters if the epilimnion is not exhausted. The other factor is a possible effect of the global climate change. Both mechanisms are not necessarily mutually exclusive. A review of regional data by Aguilar et al. (2005) revealed a warming trend since 1961 for Central America, with an increase in the frequency of extreme maximum temperatures and a decrease in the occurrence of extreme cold spells. A report by the Regional Committee of Hydraulic Resources of Central America (CRRH) published in 2008, also found an increase in the average maximum temperature of about 0.5°C between two periods: 1961-1990 and 1991-2005.
There is only a rain gauge at the lake. Data from this station (Station number 69532-Laguna de Cote) provided by the Costa Rican electricity company (Instituto Costarricense de Electricidad, 2013, unpublished data) shows a decreasing trend in monthly precipitation between 2001 and 2011, which is in agreement with predictions of future scenarios in Northern Costa Rica (Alvarado, Contreras, Alfaro, & Jiménez, 2012). Hidalgo, Amador, Alfaro & Quesada (2013) have also found a decreasing trend in precipitation for Northern Central America, North of 10°N. This decrease in precipitation, together with the observed trend of an increase in extreme dry and heavy rain events (Aguilar et al., 2005; CRRH, 2008) could be associated with an increase in direct solar radiation reaching the lake, which could also account for the warming. Other ecosystems such as Monteverde Cloud Forest, about 35Km Southeast of the lake, have been shown to be affected by warming weather (Pounds, Fogden, & Campbell, 1999). Although the effect of global climate change is controversial, for example see Nedebele-Murisa, Frank and Miles (2012) and Marshall (2012) on the changes in lake Kariba. Other tropical lakes, albeit larger in size, have shown clear effects that can be attributed to global warming (Hecky et al., 1994; Plisnier, 2000; Verburg et al., 2003). According to the empirical equation of Lewis (1987), the bottom and surface temperatures of the lake are expected to be 21.9°C and 26.4°C, respectively; these values are within the observed range of temperatures of Lake Cote, but in recent years surface and bottom temperature reached higher values than expected. The thermocline was shallower during times of higher surface water temperature. A similar effect of decreasing thermocline depth with warming was observed in Lake Kinneret, a subtropical warm lake (Hambright et al., 1994). The turbidity of the lake, with a usual Secchi depth of 2m, enhances the development of the thermocline, as solar heat cannot penetrate deep in the water column (Mazumder & Taylor, 1994) and heat is accumulated in surface waters. It also restricts photosynthetic activity to the upper layers of the water column. The vertical distribution of dissolved oxygen also showed periods of stratification, with anoxic conditions in bottom waters, especially at times of low rainfall and thermal stratification alternating with periods of complete circulation and good oxygenation in the whole water column. The development of anoxic conditions in the lake indicates that it can remain stagnant or stratified, with little mixing at least for several days in a row, in spite of its shallowness and the windiness of the region, as previously discussed.
The lake can be considered as mesotrophic, even though it has a low Chlorophyll a content as compared with eutrophic lakes around the world, some of which may reach values above 100mg/m3 (Kalff, 2002). Yet, the lake is turbid, having a Secchi transparency less than three meters, and as little as approximately one meter on some occasions. This situation is the result of the input of particulate organic matter from the surrounding vegetation, and the re-suspension of fine sediments from the bottom due to the shallowness of the lake and the turbulence generated by the windy weather that prevails in the region.
The chlorophyll content showed a decreasing trend and the Secchi transparency an increasing one. This situation can also be in part the result of the warming trend described above. General impacts of global warming on lakes could be divided in two main outcomes. One long-term effect would be a decrease in water level as a result of reduced moisture and higher temperatures which are expected to increase evaporation (Jung et al., 2010). Such lowering has been found to occur for lakes in tropical Africa during the Holocene (verschuren, Laird, & Cumming, 2000). These changes in the tropics have also been shown to correspond to global events (Hodell, Curtis, Jones, & Higuera Gundy, 1991) and have been associated with global patterns of change, such as changes in the pattern of migration of the Inter Tropical Convergence Zone (ITCZ), and in the intensity of the Pacific Oscillation (Placzek, Quade, & Patchet, 2006), and the Atlantic Oscillation (Street-Perrot & Perrot, 1990). The second main outcome, a more short-term effect, is an increase in the temperature of surface waters, which has been hypothesized to produce an increase in productivity in temperate lakes (De Stasio, Hill, Kleinhans, Nibbelink, & Magnuson, 1996; Jeppesen et al., 2011; Porter, Saunders, Haberyan, & Macubbin, 1996) and shallow Lake Victoria, Africa (Hecky et al., 1994). However, temperature increase has been shown to produce a reduction in the productivity and phytoplankton biomass in other large and deep tropical lakes, such as Lake Tanganyika (O’Reilly, Alin, Plisnier, Cohen, & McKnee, 2003; Plisnier, 2000; Verburg et al.,2003). A similar reduction in phytoplankton has been also detected for the oceans (Boyce, Lewis, & Worm, 2010). In the case of Lake Cote, this latter effect seems to be occurring, as evidenced by the reduction in suspended Chlorophyll a and an increase in the transparency of the lake. The warming favors the establishment of a more stable stratification for longer periods, as has been described for lake Pluβsee, in Northern Germany (Rössner, Müller-Navarra, & Zorita, 2012). In turn, nutrients and sediments are retained for longer periods in the hypolimnion, thus affecting the growth of phytoplankton (O’Reilly et al., 2003; Plisnier, 2000; Verburg et al., 2003) and enhancing the sedimentation of suspended seston, which represents a transport of nutrients out of the euphotic zone. In Lake Cote the nitrate showed a small effect on Chlorophyll a given the low value of the regression coefficient. Petersen & Umaña (2002) working in two other lakes in Costa Rica (Bonilla and Bonillita) that are deeper and more stably stratified than Cote, found that nutrient limitation may vary between nearby lakes, and that a shift from phosphorus to nitrogen limitation may occur with seasonality of rains. The small effect of nutrient variations on chlorophyll a concentration in Lake Cote is an indication that nutrient levels are not limiting and other factors might be limiting to phytoplankton growth, or that assimilation and growth are decoupled in time, as pointed out by some researchers such as Sommer (1989) and Reynolds (2006).
DeSenerpont et al. (2013) proposed that tropical lakes would be affected by increased washout under the assumption of an increase in rainfall. This is not the case in Lake Cote. There have been heavy rain events which produce lower transparency due to an episodic increase in suspended solids, but the overall effect has been of a tendency to increased transparency and higher temperatures instead. The data however does not allow testing for the effect of heavy rainstorms on the thermal stratification. Larger than normal inputs of suspended matter after periods of heavy rains is the result of deforestation in the catchment basin, especially if intensity of rain events has been increasing as reported by Aguilar et al. (2005) and the CRRH (2008) report. Land use changes in the Lake Cote drainage basin occurred before 1990, as can be observed in the works of Sader and Joyce (1988) and Sánchez-Azofeifa, Harris, and Skole (2001). In Costa Rica, most of the deforestation took place before 1985 (Bush, Sathaye, & Sánchez-Azofeifa, 2000), and forest cover has been recovering since then (Baltodano, 2008; Kleinn, Corrales, & Morales, 2002). So, the changes in lake conditions observed in Lake Cote in this 10 year period cannot be attributed solely or mainly to changes in land use and forest cover. A study of the stratigraphy of the lake sediments by Arford (2001) showed that although some eruptive events in nearby active Arenal volcano reached Lake Cote, the most recent ones after the 1968 eruptions did not affect the lake, so this volcanic disturbance can also be considered negligible at Lake Cote during the monitoring period. Although the time length of the observations is short in comparison with other studies of global climate change and it is difficult to ascertain that the observed trends are real long-term trends instead of decadal short term fluctuations, the correspondence with changes observed elsewhere is interesting and suggest that the effect of global climate change is in part responsible for the changes detected in this tropical lake.
Acknowledgments
This study was made possible in part through a research grant from the vicerrectoría de Investigación, Universidad de Costa Rica, Centro de Investigación en Ciencias del Mar y Limnología (CIMAR), No. 808-89-063 in 1989, and later through the contract from the Compañía Nacional de Fuerza y Luz (CNFL) in Costa Rica, to CIMAR for the monitoring of the lake Cote before, during and after the modification of the lake for electricity generation, from 2002 until 2011, projects No. 808-A2-524 and 808-A2-400. The work was possible thanks to the dedicated assistance of Luis Rolier Lara Gutiérrez, Eddy Gómez Ramírez and Aldo Farah Pérez either in the field and with the analysis of samples in the laboratory, and many other people who came along during all these years to the field trips among which I could mention, Jeffry Sibaja, Pablo Gutiérrez, Farrel Ruiz, Octavio Esquivel, Anne Marie Gavlas, Delia Zavala, Arturo Angulo, and many others.
References
Aguilar, E., Peterson, T. C., Ramírez Obando, P., Frutos, R., Retana, J. A., Solera, M., & Mayorga, R. (2005). Changes in precipitation and temperature extremes in Central America and northern South America, 1961- 2003. Journal of Geophysical Research, 110 (D23). [ Links ]
Alvarado, L. F., Contreras, W., Alfaro, M., & Jiménez, E. (2012). Escenarios de cambio climático regionalizados para Costa Rica. San José, CR: MINAET-IMN. [ Links ]
Arford, M. R. (2001). Late Holocene environment history and tephrostratigraphy in northwestern Costa Rica: a 4000 year record from Lago Cote. (Doctoral dissertation, University of Tennessee, Konxville). [ Links ]
Baltodano, J. (2008). Bosque, cobertura y recursos forestales. Ponencia preparada para el Decimocuarto Informe Estado de la Nación. Programa Estado de la Nación, San José, Costa Rica. Retrieved from http://coecoceiba.org/wp-content/subidas/2009/11/ Bosques-coberturas-Baltodano-2008.pdf. [ Links ]
Boyce, D. G., Lewis, M. R., & Worm, B. (2010). Global phytoplankton decline over the past century. Nature, 466, 591-596. [ Links ]
Bush, C. B., Sathaye, J. A., & Sánchez-Azofeifa, G. A. (2000). Estimating the greenhouse gas benefits of forestry projects: A Costa Rican case study. Berkeley, USA: Energy Analysis Department, Lawrence Berkeley National Laboratory. [ Links ]
Comité Regional de Recursos Hídricos. (2008). Clima, variabilidad y cambio climático en Costa Rica. San José, CR: Instituto Meteorológico Nacional. [ Links ]
DeSenerpont, L. N., Elser, J. J., Gsell, A. S., Huszar, V. L.M., Ibelings, B. W., Jeppesen, E., Kosten, S., Mooij, W. M., Roland, F., Sommer, U., Van Donk, E., Winder, M., & Lürling, M. (2013). Plankton dynamics under different climatic conditions in space and time. Freshwater Biology, 58, 463-482. doi: 10.1111/ fwb.12053 [ Links ]
DeStasio, B. T. Jr., Hill, D. K., Kleinhans, J. M., Nibbelink, N. P., & Magnuson, J. J. (1996). Potential effects of global climate change on small north-temperate lakes: physics, fish and plankton. Limnology and Oceanography, 41, 1136-1149. [ Links ]
Dungan, P. J. (Ed.) (1992). Conservación de humedales. Un análisis de temas de actualidad y acciones necesarias. Gland, Suiza: UICN. [ Links ]
Ford, D. E. (1990). Reservoir transport processes. In K.W. Thornthon, B. L. Kimmel, & Payne, F. E. (Eds.), Reservoir limnology: Ecological perspectives (pp. 15-41). New York, NY: Wiley. [ Links ]
Haberyan, K. A., Horn, S. P., & Umaña, G. (2003). Limnology of fifty-one lakes in Costa Rica. Revista de Biología Tropical, 51, 107-122. [ Links ]
Hambright, D., Gophen, M., & Serruya, S. (1994). Influence of Long-Term Climatic Changes on the Stratification of a Subtropical, Warm Monomictic Lake. Limnology and Oceanography, 39, 1233-1242. [ Links ]
Hammer, Ø., Harper, D. A. T., & Ryan, P. D. (2001). PAST: Paleotological Statistics software package for education and data analysis. Paleontologia Electronica, 4, 9p. http://palaeo-electronica.org/2001_1/past/ issue1_01.htm [ Links ]
Hecky, R. E., Bugenyi, F. W. B., Ochumba, P., Talling, J. F., Mugidae, R., Gophen, M., & Kaufman, L. (1994). Deoxygenation of the deep water of lake victoria, East Africa. Limnology and Oceanography, 39, 1476-1481. [ Links ]
Hidalgo, H. G., Amador, J. A., Alfaro, E. J., & Quesada, B. (2013). Hydrological climate change projections for Central America. Journal of Hydrology, 495, 94-112. doi: 10.1016/j.jhydrol.2013.05.004 [ Links ]
Hodell, D. A., Curtis, J. H., Jones, G. A., & Higuera Gundy, A. (1991). Reconstruction of Caribbean climate chage over the past 10500 years. Nature, 352,790-793. [ Links ]
Jeppesen, E., Kronvang, B., Olesen, J. E., Audet, J., Søndergaard, M., Hoffmann, C. C., Andersen, H. E., Lauridsen, T. L., Liboriussen, L., Larsen, S. E., Beklioglu, M., Meerhoff, M., Özen, A., & Özkan, K. (2011). Climate change effects on nitrogen loading from cultivated catchments in Europe: implications for nitrogen retention, ecological estate of lakes and adaptation. Hydrobiologia, 663, 1-21. [ Links ]
Jung, M., Reichstein, M., Ciais, P., Seneviratne, S. I., Sheffield, J., Goulden, M. L., Bonan, G. (2010). Recent decline in the global land evapotranspiration trend due to limited moisture supply. Nature, 467, 951-954. doi:10.1038/nature09396 [ Links ]
Kalff, J. (2002). Limnology. New Jersey, USA: Prentice-Hall. [ Links ]
Kleinn, C., Corrales, L., & Morales, D.(2002). Forest cover in Costa Rica: A comparative study of tropical forest cover estimates over time. Environmental Monitoring and Assessment, 73, 17-40. [ Links ]
Lewis, W., Jr. (1987). Tropical limnology. Annual Review of Ecology and Systematics, 18, 159-184. [ Links ]
Marshall, B. E. (2012). Climate change does not explain historical changes in the pelagic ecosystem of Lake Kariba (Zambia-Zimbabwe). Lakes and Reservoirs: Research and Management, 17, 265-274. [ Links ]
Mazumder, A. & Taylor, W. D. (1994). Thermal structure of lakes varying in size and water clarity. Limnology and Oceanography, 39, 968-976. [ Links ]
Muñoz, A. C., Fernández, W., Gutierrez, J. A., & Zárate, E. (2002). Variación estacional del viento en Costa Rica y su relación con los regímenes de lluvia. Tópicos Meteorológicos y Oceanográfícos, 9, 1-13. [ Links ]
Nedebele-Murisa, M. R, Frank, M. C., & Miles, R. L. (2012). Phytoplankton biomass and primary production dynamics in lake Kariba. Lakes and Reservoirs: Research and Management, 17, 275-289. [ Links ]
O’Reilly, C. M., Alin, S. R., Plisnier, P. D., Cohen, A. S., & McKnee, B. A. (2003). Climate change decreases aquatic ecosystem productivity of Lake Tanganyika, Africa. Nature, 424, 766-768. [ Links ]
Parsons, T. R., Maita, Y., & Lalli, C. M. (1984). A manual of chemical and biological methods for seawater analysis. Oxford, UK: Oxford. [ Links ]
Petersen, R. & Umaña, V. G. (2002). Nitrogen and phosphorus limitation in Lakes Bonilla and Bonillita, Costa Rica. Verhandlungen des Internationalen Verein Limnologie, 28, 1520-1525. [ Links ]
Placzek, C., Quade, J., & Patchet, P. J. (2006). Geochronology and stratigraphy of late Pleistocene lake cycles on the southern Bolivian Altiplano: implications for causes of tropical climate change. Bulletin of the Geological Society of America, 118, 515-532. [ Links ]
Plisnier, P. D. (2000). Recent climate and limnological changes in lake Tanganika. Verhanlungen des Internationalen Verein Limnologie, 27, 1-4. [ Links ]
Porter, K. G., Saunders, P. A., Haberyan, K. A., & Macubbin, A. E. (1996). Annual cycle of autotrophic and heterotrophic production in a small, monomictic Piedmont lake (Lake Oglethorpe): Analog for the effects of climatic warming on dimictic lakes. Limnology and Oceanography, 41, 1041-1051. [ Links ]
Pounds, J. A., Fogden, M. P. L., & Campbell, J. H. (1999). Biological response to climate change on a tropical mountain. Nature, 398, 611-615. [ Links ]
R Development Core Team. (2011). R: a language and environment for statistical computing. R Foundation for Statistical Computing. Vienna, Austria. (www.R-project.org). [ Links ]
Reynolds, C. S. (2006). Ecology of phytoplankton. Edinburgh: Cambridge. [ Links ]
Roldán Pérez, G., & Ramírez Restrepo, J. J. (2008). Fundamentos de limnología neotropical. Medellín, Colombia: Universidad de Antioquía. [ Links ]
Rösner, R., Müller-Navarra, D. C., & Zorita, E. (2012). Trend analysis of weekly temperatures and oxygen concentrations during summer stratification in Lake Plußsee: A long-term study. Limnology and Oceanography, 57, 1479-1491. [ Links ]
Sader, S. & Joyce, A. (1988). Deforestation rates and trends in Costa Rica 1940 to 1983. Biotropica, 20, 11-19. [ Links ]
Sánchez-Azofeifa, G. A., Harris, R. C., & Skole, D. L. (2001). Deforestation in Costa Rica: A quantitative analysis using remote sensing imagery. Biotropica, 33, 378-384. [ Links ]
Sommer, U. (1989). The role of competition for resources in phytoplankton successionl. In U. Sommer (Ed.), Plankton ecology: succession in plankton communities (pp. 57-106). Berlin: Springer. [ Links ]
Street-Perrot, F. A. & Perrot, R. A. (1990). Abrupt climatic fluctuations in the tropics: the influence of Atlantic Ocean circulation. Nature, 343, 607-612. [ Links ]
Thornthon, K. W., Kimmel, B. L., & Payne, F. E. (1990). Reservoir limnology: Ecological perspectives. New York, NY: Wiley. [ Links ]
Umaña, G., Haberyan, K. A., & Horn, S. P. (1999). Limnology in Costa Rica. In R.G. Wetzel & Gopal, B. (Eds.), Limnology in Developing Countries (vol. 2., pp. 33-62). International Association of Theoretical and Applied Limnology. [ Links ]
Verburg, P., Hecky, R. E., & Kling, H. (2003). Ecological consequences of a century of warming in lake Tanganyika. Science, 301, 505-507. [ Links ]
Verschuren, D., Laird, K. R., & Cumming, B. F. (2000). Rainfall and drought in equatorial east Africa during the past 1 100 years. Nature, 403, 410-414. [ Links ]
World Commission on Dams. (2000). Dams and Development. A new framework for decision-making. London, UK: Earthscan. [ Links ]
Alvarado, L. F., Contreras, W., Alfaro, M., & Jiménez, E. (2012). Escenarios de cambio climático regionalizados para Costa Rica. San José, CR: MINAET-IMN. [ Links ]
Arford, M. R. (2001). Late Holocene environment history and tephrostratigraphy in northwestern Costa Rica: a 4000 year record from Lago Cote. (Doctoral dissertation, University of Tennessee, Konxville). [ Links ]
Baltodano, J. (2008). Bosque, cobertura y recursos forestales. Ponencia preparada para el Decimocuarto Informe Estado de la Nación. Programa Estado de la Nación, San José, Costa Rica. Retrieved from http://coecoceiba.org/wp-content/subidas/2009/11/ Bosques-coberturas-Baltodano-2008.pdf. [ Links ]
Boyce, D. G., Lewis, M. R., & Worm, B. (2010). Global phytoplankton decline over the past century. Nature, 466, 591-596. [ Links ]
Bush, C. B., Sathaye, J. A., & Sánchez-Azofeifa, G. A. (2000). Estimating the greenhouse gas benefits of forestry projects: A Costa Rican case study. Berkeley, USA: Energy Analysis Department, Lawrence Berkeley National Laboratory. [ Links ]
Comité Regional de Recursos Hídricos. (2008). Clima, variabilidad y cambio climático en Costa Rica. San José, CR: Instituto Meteorológico Nacional. [ Links ]
DeSenerpont, L. N., Elser, J. J., Gsell, A. S., Huszar, V. L.M., Ibelings, B. W., Jeppesen, E., Kosten, S., Mooij, W. M., Roland, F., Sommer, U., Van Donk, E., Winder, M., & Lürling, M. (2013). Plankton dynamics under different climatic conditions in space and time. Freshwater Biology, 58, 463-482. doi: 10.1111/ fwb.12053 [ Links ]
DeStasio, B. T. Jr., Hill, D. K., Kleinhans, J. M., Nibbelink, N. P., & Magnuson, J. J. (1996). Potential effects of global climate change on small north-temperate lakes: physics, fish and plankton. Limnology and Oceanography, 41, 1136-1149. [ Links ]
Dungan, P. J. (Ed.) (1992). Conservación de humedales. Un análisis de temas de actualidad y acciones necesarias. Gland, Suiza: UICN. [ Links ]
Ford, D. E. (1990). Reservoir transport processes. In K.W. Thornthon, B. L. Kimmel, & Payne, F. E. (Eds.), Reservoir limnology: Ecological perspectives (pp. 15-41). New York, NY: Wiley. [ Links ]
Haberyan, K. A., Horn, S. P., & Umaña, G. (2003). Limnology of fifty-one lakes in Costa Rica. Revista de Biología Tropical, 51, 107-122. [ Links ]
Hambright, D., Gophen, M., & Serruya, S. (1994). Influence of Long-Term Climatic Changes on the Stratification of a Subtropical, Warm Monomictic Lake. Limnology and Oceanography, 39, 1233-1242. [ Links ]
Hammer, Ø., Harper, D. A. T., & Ryan, P. D. (2001). PAST: Paleotological Statistics software package for education and data analysis. Paleontologia Electronica, 4, 9p. http://palaeo-electronica.org/2001_1/past/ issue1_01.htm [ Links ]
Hecky, R. E., Bugenyi, F. W. B., Ochumba, P., Talling, J. F., Mugidae, R., Gophen, M., & Kaufman, L. (1994). Deoxygenation of the deep water of lake victoria, East Africa. Limnology and Oceanography, 39, 1476-1481. [ Links ]
Hidalgo, H. G., Amador, J. A., Alfaro, E. J., & Quesada, B. (2013). Hydrological climate change projections for Central America. Journal of Hydrology, 495, 94-112. doi: 10.1016/j.jhydrol.2013.05.004 [ Links ]
Hodell, D. A., Curtis, J. H., Jones, G. A., & Higuera Gundy, A. (1991). Reconstruction of Caribbean climate chage over the past 10500 years. Nature, 352,790-793. [ Links ]
Jeppesen, E., Kronvang, B., Olesen, J. E., Audet, J., Søndergaard, M., Hoffmann, C. C., Andersen, H. E., Lauridsen, T. L., Liboriussen, L., Larsen, S. E., Beklioglu, M., Meerhoff, M., Özen, A., & Özkan, K. (2011). Climate change effects on nitrogen loading from cultivated catchments in Europe: implications for nitrogen retention, ecological estate of lakes and adaptation. Hydrobiologia, 663, 1-21. [ Links ]
Jung, M., Reichstein, M., Ciais, P., Seneviratne, S. I., Sheffield, J., Goulden, M. L., Bonan, G. (2010). Recent decline in the global land evapotranspiration trend due to limited moisture supply. Nature, 467, 951-954. doi:10.1038/nature09396 [ Links ]
Kalff, J. (2002). Limnology. New Jersey, USA: Prentice-Hall. [ Links ]
Kleinn, C., Corrales, L., & Morales, D.(2002). Forest cover in Costa Rica: A comparative study of tropical forest cover estimates over time. Environmental Monitoring and Assessment, 73, 17-40. [ Links ]
Lewis, W., Jr. (1987). Tropical limnology. Annual Review of Ecology and Systematics, 18, 159-184. [ Links ]
Marshall, B. E. (2012). Climate change does not explain historical changes in the pelagic ecosystem of Lake Kariba (Zambia-Zimbabwe). Lakes and Reservoirs: Research and Management, 17, 265-274. [ Links ]
Mazumder, A. & Taylor, W. D. (1994). Thermal structure of lakes varying in size and water clarity. Limnology and Oceanography, 39, 968-976. [ Links ]
Muñoz, A. C., Fernández, W., Gutierrez, J. A., & Zárate, E. (2002). Variación estacional del viento en Costa Rica y su relación con los regímenes de lluvia. Tópicos Meteorológicos y Oceanográfícos, 9, 1-13. [ Links ]
Nedebele-Murisa, M. R, Frank, M. C., & Miles, R. L. (2012). Phytoplankton biomass and primary production dynamics in lake Kariba. Lakes and Reservoirs: Research and Management, 17, 275-289. [ Links ]
O’Reilly, C. M., Alin, S. R., Plisnier, P. D., Cohen, A. S., & McKnee, B. A. (2003). Climate change decreases aquatic ecosystem productivity of Lake Tanganyika, Africa. Nature, 424, 766-768. [ Links ]
Parsons, T. R., Maita, Y., & Lalli, C. M. (1984). A manual of chemical and biological methods for seawater analysis. Oxford, UK: Oxford. [ Links ]
Petersen, R. & Umaña, V. G. (2002). Nitrogen and phosphorus limitation in Lakes Bonilla and Bonillita, Costa Rica. Verhandlungen des Internationalen Verein Limnologie, 28, 1520-1525. [ Links ]
Placzek, C., Quade, J., & Patchet, P. J. (2006). Geochronology and stratigraphy of late Pleistocene lake cycles on the southern Bolivian Altiplano: implications for causes of tropical climate change. Bulletin of the Geological Society of America, 118, 515-532. [ Links ]
Plisnier, P. D. (2000). Recent climate and limnological changes in lake Tanganika. Verhanlungen des Internationalen Verein Limnologie, 27, 1-4. [ Links ]
Porter, K. G., Saunders, P. A., Haberyan, K. A., & Macubbin, A. E. (1996). Annual cycle of autotrophic and heterotrophic production in a small, monomictic Piedmont lake (Lake Oglethorpe): Analog for the effects of climatic warming on dimictic lakes. Limnology and Oceanography, 41, 1041-1051. [ Links ]
Pounds, J. A., Fogden, M. P. L., & Campbell, J. H. (1999). Biological response to climate change on a tropical mountain. Nature, 398, 611-615. [ Links ]
R Development Core Team. (2011). R: a language and environment for statistical computing. R Foundation for Statistical Computing. Vienna, Austria. (www.R-project.org). [ Links ]
Reynolds, C. S. (2006). Ecology of phytoplankton. Edinburgh: Cambridge. [ Links ]
Roldán Pérez, G., & Ramírez Restrepo, J. J. (2008). Fundamentos de limnología neotropical. Medellín, Colombia: Universidad de Antioquía. [ Links ]
Rösner, R., Müller-Navarra, D. C., & Zorita, E. (2012). Trend analysis of weekly temperatures and oxygen concentrations during summer stratification in Lake Plußsee: A long-term study. Limnology and Oceanography, 57, 1479-1491. [ Links ]
Sader, S. & Joyce, A. (1988). Deforestation rates and trends in Costa Rica 1940 to 1983. Biotropica, 20, 11-19. [ Links ]
Sánchez-Azofeifa, G. A., Harris, R. C., & Skole, D. L. (2001). Deforestation in Costa Rica: A quantitative analysis using remote sensing imagery. Biotropica, 33, 378-384. [ Links ]
Sommer, U. (1989). The role of competition for resources in phytoplankton successionl. In U. Sommer (Ed.), Plankton ecology: succession in plankton communities (pp. 57-106). Berlin: Springer. [ Links ]
Street-Perrot, F. A. & Perrot, R. A. (1990). Abrupt climatic fluctuations in the tropics: the influence of Atlantic Ocean circulation. Nature, 343, 607-612. [ Links ]
Thornthon, K. W., Kimmel, B. L., & Payne, F. E. (1990). Reservoir limnology: Ecological perspectives. New York, NY: Wiley. [ Links ]
Umaña, G., Haberyan, K. A., & Horn, S. P. (1999). Limnology in Costa Rica. In R.G. Wetzel & Gopal, B. (Eds.), Limnology in Developing Countries (vol. 2., pp. 33-62). International Association of Theoretical and Applied Limnology. [ Links ]
Verburg, P., Hecky, R. E., & Kling, H. (2003). Ecological consequences of a century of warming in lake Tanganyika. Science, 301, 505-507. [ Links ]
Verschuren, D., Laird, K. R., & Cumming, B. F. (2000). Rainfall and drought in equatorial east Africa during the past 1 100 years. Nature, 403, 410-414. [ Links ]
World Commission on Dams. (2000). Dams and Development. A new framework for decision-making. London, UK: Earthscan. [ Links ]
*Correspondencia a:
Gerardo Umaña: Centro de Investigación en Ciencias del Mar y Limnología (CIMAR) and Escuela de Biología, Universidad de Costa Rica; gerardo.umana@ucr.ac.cr
1.Centro de Investigación en Ciencias del Mar y Limnología (CIMAR) and Escuela de Biología, Universidad de Costa Rica; gerardo.umana@ucr.ac.cr
Received 05-IV-2013. Corrected 20-X-2013. Accepted 21-XI-2013.