Services on Demand
Journal
Article
Indicators
-
Cited by SciELO
-
Access statistics
Related links
-
Similars in SciELO
uBio
Share
Revista de Biología Tropical
On-line version ISSN 0034-7744Print version ISSN 0034-7744
Rev. biol. trop vol.56 n.1 San José Mar. 2008
Do seasonal changes in light availability influence the inverse leafing phenology of the neotropical dry forest understory shrub Bonellia nervosa (Theophrastaceae)?
Oscar M. Chaves1,2 & Gerardo Avalos1,3
1 Escuela de Biología, Universidad de Costa Rica, Ciudad Universitaria Rodrigo Facio, San Pedro, San José, Costa Rica; ochaves@gmail.com
2 Instituto para la Conservación y el Desarrollo Sostenible San José, Costa Rica; Universidad Nacional Autónoma de México, Antigua Carretera a Pátzcuaro No. 8701 , Col. Ex-Hacienda de San José de La Huerta, C.P. 58190 Morelia, Michoacán, México; ochaba@oikos.unam.mx; incodeso@yahoo.com
3 The School for Field Studies, Center for Sustainable Development Studies, 10 Federal St., Salem, MA 01970 USA; avalos@fieldstudies.org
Abstract: In tropical dry forests most plants are deciduous during the dry season and flush leaves with the onset of the rains. In Costa Rica, the only species displaying the opposite pattern is Bonellia nervosa. To determine if seasonal changes in light availability are associated with the leaf and reproductive phenology of this species, we monitored leaf production, survival, and life span, as well as flower and fruit production from April 2000 to October 2001 in Santa Rosa National Park. Leaf flushing and flower bud production took place shortly after the autumnal equinox when day length starts to decrease. Leaves began expansion at the end of the wet season, and plants reached 70 % of their maximum leaf area at the beginning of the dry season, maintaining their foliage throughout the entire dry period. Leaf shedding occurred gradually during the first three months of the wet season. Leaf flushing and shedding showed high synchrony, with leaf numbers being related to light availability. Maximum leaf production coincided with peaks in radiation during the middle of the dry season. Decreasing day length induces highly synchronous flower bud emergence in dry forest species, but this is the first study indicating induction of leaf flushing by declining day length. Rev. Biol. Trop. 56 (1): 257-268. Epub 2008 March 31.
Key words: photoperiodic induction, inverse phenology, Bonellia nervosa, Santa Rosa National Park, tropical dry forest.
Tropical dry forests portray a diverse mosaic of phenological strategies, which facilitate plant adjustment to changes in the availability of water, light, and nutrients across significant spatial and temporal environmental gradients (Borchert 1994, 2000). The phenological patterns of tropical dry forest plants are complex (Borchert 1994), and range from evergreen species restricted to moist microsites along riparian habitats, to dry-season deciduous species occupying some of the driest habitats including seasonal deserts found in the Neotropics (Borchert et al. 2004). Understanding the integration of the environmental and endogenous controls of such diverse phenological patterns stretches the limits of plant physiological ecology (Borchert 1983, 2000, Rivera et al. 2002, Borchert et al. 2005), requiring a detailed knowledge of physiological mechanisms within the ecological and evolutionary context under which they are expressed.
In the tropical dry forests of Guanacaste, Costa Rica, most woody plants become deciduous during the dry season (Janzen 1967, Frankie et al. 1974, Opler et al. 1980, Reich and Borchert 1984), but some species show an inverse leafing phenology, producing and maintaining all leaves during the dry season, to abscise them with the onset of the rains when the canopy begins to close (Janzen 1970, Sobrado 1986, Reich 1995, Roupsard et al. 1999). Bonellia nervosa C. Presl. (Theophrastaceae) formerly Jacquinia pungens (Janzen 1970, 1983, Daubenmire 1972, Bullock and Solís-Magallanes 1990, Ståhl and Källersjö 2004) belongs to this category. An inverse leafing phenology is prevalent in phreatophytic species (Janzen 1970, Holbrook et al. 1995) where the depth of the root system facilitates access to underground water sources that reduce water stress during the dry period (Dawson and Pate 1996, Roupsard et al. 1999). This strategy expands the previous classification of dry forest phenological strategies into functional groups (Borchert 1994), being in contrast with that of leaf-exchanging species (which drop leaves at the start of the dry season, and expand new leaves almost immediately as depending on the availability of water), or with the phenological pattern of spring-flushing species (which flush new leaves around the spring equinox before the first rains, Elliott et al. 2006). These latter species are restricted to humid microsites that have the water to afford leaf expansion, and remain well hydrated throughout the rest of the dry season (Borchert 1994, Elliott et al. 2006). Phreatophytic species belong into a category of their own, being analogous to cold-temperate understory plants of deciduous forests, which complete their reproductive cycle early in the spring before trees leaf out and the canopy closes.
B. nervosa is an understory shrub abundant in deciduous and semi-deciduous forests throughout the pacific coast of Mesoamerica, from southern Jalisco, Mexico, to northwestern Costa Rica (Ståhl 1989, Ståhl and Källersjö 2004). This species is found in mature and secondary dry forests, forest edges, and pastures, over a variety of soil conditions. The overall structure of B. nervosa is clearly xerophytic. Leaves are coriaceous, with the apex modified as a spine, and are produced synchronously at the start of the dry season. A crop of new leaves, flushed in synchrony, is likely to be more efficient controlling gas exchange during the dry season peak in understory light availability than a crown composed of overlapping leaf cohorts of different ages. This is afforded by a considerably deep root system (down to 8 m, Janzen 1970, 1983, Oberbauer 1985), which facilitates access to subsoil water when the majority of the plant community is deciduous. B. nervosa is the only species in its family showing this intriguing pattern of inverse leafing phenology (B. Ståhl, pers. com.). Under more severe water seasonality (i.e., the Sonoran Desert, Mexico) B. nervosa is evergreen, but shows inverse phenology in the deciduous forests of Veracruz, México (A. Búrquez, pers. com.), which have a rainfall seasonality similar to that of our study site in Santa Rosa, Costa Rica.
In this study, we explored the extent to which seasonal changes in light availability are associated with the leafing and reproductive phenology of B. nervosa. In addition, we measured the synchrony in leaf, flower, and fruit production, and analyzed the effect of plant size on leaf, flower, and fruit production. Understanding how light is related to the phenological behavior of B. nervosa will increase our knowledge on the strength of proximate causes influencing the phenology of tropical plants. This is becoming critically important within the context of rapid changes in weather conditions related to global warming, which are influencing the phenological behavior and carbon budget of tropical forests (Wright et al. 1999, Clark et al. 2003).
Materials and methods
Study Site: this study was carried-out over 18 months (April-July 2000, and November 2000 to October 2001), along the "Indio Pelado" trail in Santa Rosa National Park, Guanacaste, Costa Rica. Santa Rosa lies between the Gulf of Papagayo and the Panamerican Highway (10°44'13'' to 11°00'37'' N and 85°34'48'' to 85°58'51'' W) at 290 m in elevation. The upper plateau maintains tropical premontane forests, whereas the coastal lowlands present a large extension of tropical dry forest (Tosi 1969). Average annual rainfall is 1 423 mm. The dry season starts in late November and extends into late April. During this period most of the canopy trees are deciduous (Janzen 1967). Average annual temperature is 25.7 °C, and average relative humidity is 81 % (Santa Rosa National Park Climate Records).
The "Indio Pelado" trail is dominated by seedlings and saplings of canopy species and understory shrubs (i.e., Cochlospermum vitifolium, Semialarium mexicanum, B. nervosa), trees of 10-20 m in height (i.e., Spondias mombin, Bursera simaruba), and a few 20-35 m tall trees still remaining from the original primary forests (i.e., Pachira quinata, Manilkara chicle, Hymenaea courbaril and Pseudobombax septenatum).
Study species: the genus Bonellia (Theophrastaceae) consists of 22 species of xerophytic shrubs and small trees distributed in dry areas of Mesoamerica, the Greater Antilles, Central America, and northern and western South America (Ståhl and Källersjö 2004). Only B. nervosa has an inverted leafing phenology. In Santa Rosa National Park, B. nervosa reaches a density of 0.1-60 adults per Ha (Janzen 1970). Adults range 1.5-6 m in height, but individuals above 5 m are rare. Leaves are coriaceous, simple, alternate, with the apex modified as a spine. Production of leaf buds start in late September to mid-October (Opler et al. 1980, pers. obs.), and leaves are dropped in July-August (pers. obs.) Small orange flowers are produced in terminal cimes, and are very likely pollinated by bees, as deduced from flower morphology and scent chemistry (Knudsen and Ståhl 1994). Fruit production takes place during the dry season (February-March), one month after anthesis. Fruit expansion extends to the onset of the rains in May. Ripe fruits are orange, and contain up to 10 seeds covered by a sweet, fleshy aril. Fruits are maintained on the plant for nearly two years (Opler et al. 1980). Although dispersal agents are not known with certainty, rodents could easily crack the fruit and eat the aril, helping to disperse the seeds (Janzen 1970).
Measurement of leaf phenology and leaf structure: leaf production and life span were monitored every two weeks from October 2000 to August 2001. We randomly selected 80 individuals with stem diameters >2 cm. Of these individuals, we randomly chose 36 plants of a variety of heights, on which all measurements were concentrated. We randomly selected 20 external branches per plant and marked them with plastic tags (200 leaves per plant, 5-15 leaves per branch). We obtained a sample of 5 leaves taken from each of 30 different adult plants, measured leaf length and width, and then estimated the actual leaf area with a Li-Cor Li-3100 leaf area meter (LI-COR Inc, Lincoln, Nebraska). We used a linear regression to calculate the leaf area in the field using leaf length and width as predictor variables (r2=0.97, N=125, p=0.001). Estimates of leaf area were obtained every week at the beginning of the dry season (between December 2000 and February 2001) using 20 marked leaves from each plant. To monitor the temporal progression of changes in leaf structure, we determined leaf mass per area (LMA) taking ten leaves per plant every two weeks from December 2000 to June 2001.
Leaf, flower and fruit synchrony: synchrony in leaf production was calculated following Augspurger (1983) using the formula: Xi=ej=j/(n-1)fi, where Xi is average synchrony in module production at the population level; ej is the number of days in which plants i and j produced a particular module; fi is the life span of the module in plant i; and n is the sample size. The index varies between 1 (100 % of synchrony) and 0 (total asynchrony).
Assessment of variation in the light environment: hemispherical canopy photographs were taken 0.5 m above the crown under overcast sky conditions using a FC-E8 Nikon Coolpix 950 digital camera adapted to a fish-eye lens of 7.5 mm. Digital images were analyzed using Hemiview (Delta-T Devices Ltd.) We analyzed the specific site distribution of direct and diffuse radiation (site factors) using the expected radiation values per site. We obtained the direct site factor to evaluate the fraction of direct radiation reaching each plant relative to the total amount of light available at the top of the canopy. We calculated the indirect site factor (diffuse factor, or radiation filtered by the canopy) observed at the plant crown relative to the radiation reaching an open site. Using these indices, combined with photon flux density data (PFD) obtained from the Santa Rosa National Park weather station, we calculated values of direct and indirect radiation reaching each plant, according to the canopy conditions and light intensities prevalent at each sampling date.
Statistical analyses: to determine the influence of radiation on leaf, flower and fruit production over time, we applied general mixed linear models (GMLMs) for repeated measures (SAS 1994) considering that deviations from normality and heterogeneity of variances of the response variables did not allow the use of MANOVA or repeated measures ANOVA. GMLMs do not assume normal distributions or homogeneous variances, but rather inspect different probabilistic distributions to calculate the residual error (Breslow and Clayton 1993). The models calculate a response variable Y from a linear function of a group of values y1, y2, ..., yp obtained from the original response variables Y1, Y2, ..., Yp. This response variable is known as the linking function (g(µ)):
g(µ)=ß0+ßixi
According to Breslow and Clayton (1993), the regression coefficients, ßi, compare the effects of each factor ai on the dependent variable Y with a base factor chosen at random, whose effect by definition is zero. When ßi>0 the base factor increases the value of Y, if ßi<0 the base factor decreases the value of Y, and when ßi=0 there is no effect on Y. In this case, the effects of indirect and direct radiation and plant size on leaf, flower and fruit production were analyzed through GMLMs using a logarithmic linking function, since the data and the error showed a negative binomial distribution (X2=74.5, df=224, p=0.34). We obtained the following model:
g(µ) = log(p)= µ + ß1x1+ ß2x2 + ß3x3 + e,
where p is leaf, flower or fruit production assuming a repeated measures model, x1 is the effect of direct radiation, x2 is the effect of indirect radiation, and x3 is plant size. Finally, e is the residual error according to the negative binomial distribution. The degree of significance of each component is calculated using a X2 test. To control for Type I errors we applied a sequential Bonferroni correction following Rice (1989).
Results
Influence of light and plant size on leaf phenology: direct light was related to leaf and flower bud production, and number of flowers. Diffuse light (indirect light) and plant size were not associated with leaf and reproductive phenology (Table 1). Leaf flushing and bud production took place right after the autumnal equinox following a decrease in day length. Leaves started expanding at the end of the wet season (mid to late October), so that plants reached about 70 % of their maximum crown area at the beginning of the dry season (November). Leaf numbers were coupled with the temporal variation in direct light (Table 1, Fig. 1A). The number of new leaves per plant changed with the variation in direct light throughout the study period (r2=0.37, N=27, p=0.0006). Leaf numbers and direct light reached a peak high in February during the middle of the dry season, and then decreased with the onset of the rains in May as the canopy began to close, although decreasing leaf numbers lagged two weeks behind the reduction in direct light after the start of the rains in May (Fig. 1A). Peaks in flower and fruit production in February 2001 coincided with a peak in direct light that lasted two months (Fig. 1B). The increase in direct light coincided with the onset of leaf flushing and the production of flower buds. This temporal relationship was reflected in a significant association between direct light on leaf, flower bud, and flower production, and a significant interaction between direct light and time vs. leaf production (Table 1). All plants were deciduous by August (mid rainy season). Average life span was 11.15 months (SD=1.33, N=120), which included the time from leaf bud production to leaf shedding. This value could be overestimated, since most senescent leaves reached a light yellow coloration by mid July, but remained on the plant a substantial time, before falling off. The synchrony in leaf production was considerably high (mean=0.88, SD=0.06, N=36 plants).
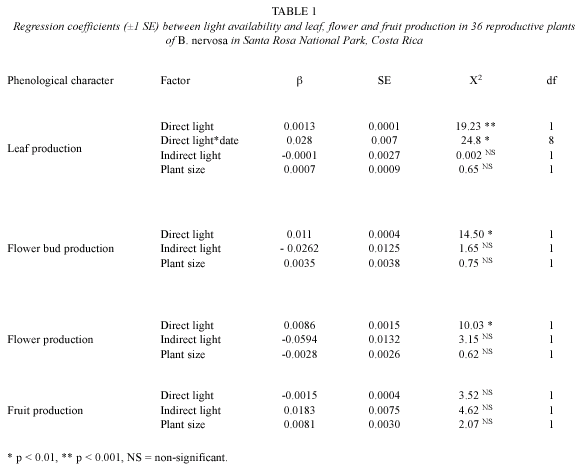
Leaf production coincided with flower bud production in the majority of plants (Fig. 1A). Anthesis started by mid January and reached a peak during the first week of February (Fig. 1B). The synchrony of flower (mean=0.38, SD=0.26, N=36) and fruit production (mean=0.18, SD=0.18, N=36) was relatively low. Only direct light was significantly related to the number of flowers during the study period. The number of fruits was not associated with direct light, indirect light or plant size (Table 1).
In addition to the significant increase in PFD in the understory observed in October 2001 (Fig. 1A), we recorded a slight increase in mean air temperature of 0.76 ºC (from 23.68 ºC ±0.47 in September to 24.44 ºC ±0.7 in October, Fig. 2). The low magnitude of the differences and the timing of peaks in air temperature makes this factor unlikely to have affected leaf production, since the highest temperature values were observed in April at the end of the dry season (Fig. 2), whereas the peak in leaf production was observed in February (Fig. 1A).
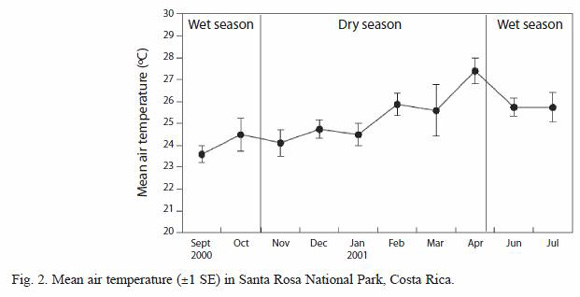
Leaf mass per area (LMA): this parameter showed a steady increase throughout the study period (Fig. 3A). It increased with the advancing dry season, reaching 103 gm-2 (SD=33.77, N=215) at the end of May, and then it decreased during the following months. The decrease in LMA was associated with loss of photosynthetic pigments, and an increase in the yellow hue of the leaf before abscission.
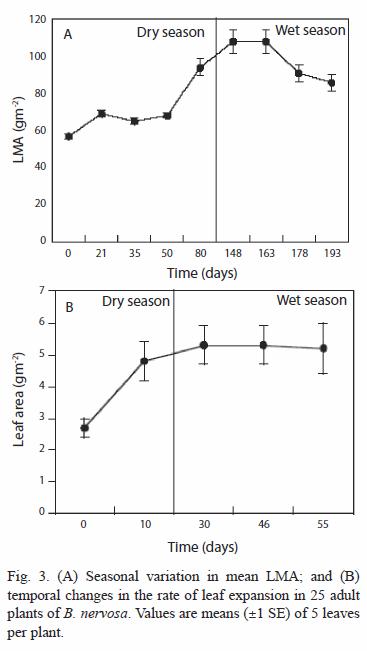
Rate of leaf expansion: leaf expansion was completed in 20.5 d (SD=5.7, N=150, Fig. 3B) reaching 5.28 cm2 (SD=3.32, N=155) at the end of the wet season. Leaf area in fully expanded leaves was not related to the initial light environment in which expansion took place (r2=0.031, N=18, p=0.57).
Discussion
In weakly seasonal tropical forests the vegetative and reproductive phenology of canopy trees is concentrated during periods of high irradiation (Janzen 1967, Wright 1992, Wright and van Schaik 1994, Grace et al. 1995, Angulo-Sandoval and Aide 2000, Hamann 2004). This tendency has been observed for diameter increases in the evergreen forests of Suriname (Schultz 1960), in the flowering of canopy trees of peninsular Malaysia (Wright and van Schaik 1994), in diameter growth and flower and fruit production of canopy trees at La Selva, Costa Rica (Raich et al. 1991, Clark and Clark 1994), in leaf (Barone 1998) and flower production on Barro Colorado Island, Panama (Croat 1969, 1975), and in seed production of canopy trees in Borneo (Wycherley 1973). The increased light conditions caused by El Niño on Barro Colorado Island favored fruit production by reducing light limitation and synchronizing reproduction (Wright et al. 1999).
In contrast, in tropical dry forests, water limitation and thus, susceptibility to seasonal drought is considered the most important factor driving leafing and reproductive phenology (Borchert 1983, 1994, Lieberman and Lieberman 1984). The seasonality of water availability entrains vegetative growth, synchronizing it to match periods in which water is not limiting (Borchert 1983). In this manner, plant physiological status is affected by water seasonality, which controls vegetative growth and consequently determines flower periodicity. However, these controls are not simple, and depend on the vascular architecture, overall tree architecture and morphology, and the pattern of biomass allocation (i.e., amount of biomass allocated to roots). Recently, several authors have pointed out that leaf flushing and flower production in tropical forests during the dry season can be induced by increasing day length (spring equinox in the northern hemisphere, Borchert et al. 2005), being facilitated by sufficient access to subsoil water to afford leaf production and maintenance during the remaining part of the dry season (Rivera et al. 2002, Borchert et al. 2005, Elliot et al. 2006). This scenario could work in the opposite manner for B. nervosa, since leaf flushing and flower bud production took place shortly after the autumnal equinox when day-length started to decrease. Leaves started expansion at the end of the wet season (mid-to-late October), so that plants reached 70 % of their maximum leaf standing crop at the beginning of the dry season, maintaining their foliage throughout the entire dry period. Decreasing day length induces the highly synchronous emergence of flower buds in deciduous tropical dry forest tree species (Rivera and Borchert 2001, Borchert et al. 2004), but induction of leaf flushing by declining day length has not been reported until now. Even in the driest microhabitats of tropical dry forests there is clear selection for increased leaf area when the opportunity for carbon gain is high, as long as water stress is prevented. Borchert (1994) found that trees colonizing dry upland forests concentrated flushing at the start of the rainy season, but those near a river peaked before the start of the dry season. In the monsoon forests of Thailand and India most trees flush new leaves in March and May, the driest and hottest time of the year, just 1-2 months before the first monsoon rains, relying on subsoil water to afford leaf production. In these forests, leaf flushing is induced by an increase in photoperiod after the spring equinox (Elliot et al. 2006). Congruent with these patterns, in B. nervosa whose roots can reach subsoil water leaf and flower production were closely related to increased levels of direct light, following the autumnal equinox. Janzen (1970) observed leaf production from late November to early December coinciding with canopy aperture and increased light intensity, whereas Opler et al. (1980) reported leaf flushing in September. Methodological differences in the monitoring of leaf flushing, as well as microhabitat differences, are most likely responsible for these discrepancies. However, we observed the initiation of leaf production and shortly after, flower bud production in midto-late October with a relatively high degree of synchrony in leaf production.
In contrast to leaf production, flower and fruit production had low values of synchrony. It is likely that plants, independently of their size, invest in reproduction according to their access to resources. Leaf and flower bud production were very likely the result of storage, since the initiation of both processes coincided with increased canopy aperture and both peaked relatively quickly. Flower initiation was not determined by achieving a peak in leaf area, although it is possible that concentrating leaf area during the dry season favors the rapid nutrient accumulation to balance the expenses of growth and reproduction the following year. Although increased light was associated with flower bud production and number of flowers, it was not related to fruit production. To fully explain these differences it is necessary to study the reproductive ecology of B. nervosa, which still is poorly understood. The number of flowers that are fertilized is dependent on the behavior of pollinators, but this aspect, along with the dispersal ecology of the species, is not well known.
Patterns of plant phenology in the tropics are highly complex, and are affected by multivariate factors. Irrigation experiments in dry forests (e.g., Reich and Borchert 1984) and seasonal moist forests (e.g., Barro Colorado Island, Wright and Cornejo 1990) show high variation in the timing of leaf flushing and leaf fall, which for many species are not associated with water stress. Changes in photoperiod, relative humidity, wind speed, and incident radiation are superimposed on the life history strategies of biomass and reproductive allocation, as affected by competitors and seed predators (e.g., Liberman and Lieberman 1984, Aide 1992), as well as by endogenous rhythms (correlative controls following Borchert 2000). Since physical and biological factors work simultaneously, it is not surprising that most phenological studies are based on correlations between changes in phenological characters and the temporal variation in physical factors, whose causality is established by proposing a tentative physiological mechanism. Most of the studies that have increased our understanding of the phenological patterns of tropical plants by presenting testable hypotheses are based on correlative observations and indirect evidence (i.e., consistent patterns across species and ecosystems, Borchert 2000, Borchert et al. 2004, 2005, Elliot et al. 2006). Controlling and isolating environmental factors at the community level is not feasible. Simpler experiments, using seedlings in growth chambers, are more reasonable to implement, but whether their conclusions can be extended to complex tropical dry forest communities is still under dispute. Within this context, our methods of analyses were exploratory, and the clear timing between direct light and leaf and flower production suggests a link between light availability and leaf phenology in B. nervosa. This pattern is congruent with the production of maximum leaf area during periods of high light availability (with leaf flushing cued by decreasing photoperiod), and rapid leaf loss shortly after canopy closure. Our results showed a high degree of synchrony in leaf production, which changed in accordance with canopy aperture. Although in several tropical dry forest species early leaf flushing coincides with peaks in air temperature (i.e., Tabebuia rosea, Gómez and Fournier 1996, and Enterolobium cyclocarpum, Rojas 2001); we do not consider this to be the case in B. nervosa, due to the low magnitude of the temperature differences and the timing of peaks in air temperature.
It is well established that in adults of B. nervosa the root system can reach 8 m in depth (Janzen 1970), allowing access to subsoil water throughout the entire dry season. In Faidherbia albida, another species with inverse phenology, roots can reach 30 m in depth (Dupuy and Dreyfus 1992). Roupsard et al. (1999) showed that the phreatophytic character of this species enables it to withstand the dry season without being exposed to severe water stress. Similar biomass allocation patterns have been observed in seedlings of B. nervosa (pers. obs.).
B. nervosa is specialized to exploit the higher light levels prevalent in the understory during the dry season. This was reflected in the tight coupling between the leafing phenology and increased light levels, the presence of a deep root system, and the magnitude and temporal progression of leaf structural characters. In addition, leaf and flower bud production were triggered by decreasing day length, since both processes began with high synchrony shortly after the autumnal equinox. Due to the potentially high respiratory demands and the reduced light levels, B. nervosa looses all its foliage during the wet season. In most sun-adapted species, high metabolic rates represent high respiratory costs relative to shade-adapted species (Bazzaz and Carlson 1982, Pearcy et al. 1987, Mulkey et al. 1993, Chazdon et al. 1996, Zotz and Winter 1996), and are incompatible with prolonged shade (Givnish 1988, Chazdon et al. 1996).
Future studies should examine the environmental controls of light quantity and quality on the plasticity of expression of the inverse leafing phenology, as well as the factors that influence the transition of B. nervosa seedlings from evergreen to deciduous. It is likely that with higher light availability during the wet season B. nervosa could maintain an evergreen habit, but it is not clear how the leafing phenology changes with light, water access, and photoperiod in other latitudes. Our study has only outlined the basic phenological pattern, and calls for more in situ, mechanistic studies on the plasticity and evolution of the inverse leafing phenology of this dry forest understory shrub.
Acknowledgments
This article is dedicated to the memory of one of the pioneers of the study of the phenology of tropical trees, Luis Fournier. The authors thank Oscar J. Rocha and Luis Fournier for their logistical support during fieldwork. Jorge Lobo helped with statistical analyses. María Marta Chavarría and Felipe Chavarría provided invaluable help during fieldwork in Santa Rosa. Rolf Borchert provided helpful criticism on an early version of this manuscript. Blair Brown proofread the final draft. This research was supported by the Graduate School of the University of Costa Rica (SEP), the Organization for Tropical Studies (OTS) through an Andrew W. Mellon Foundation fellowship, and by the organization IDEAWILD.
Resumen
En los bosques tropicales secos la mayoría de las plantas pierden sus hojas durante la estación seca y las producen con el inicio de las lluvias. En Costa Rica la única especie que muestra el patrón fenológico inverso es Bonellia nervosa. Para determinar si los cambios estacionales en la disponibilidad de luz estaban asociados con la fenología foliar y reproductiva en esta especie, monitoreamos la producción y sobrevivencia de hojas, así como la producción de flores y frutos de abril del 2000 a octubre del 2001 en el Parque Nacional Santa Rosa. La producción de hojas y botones florales ocurrió poco después del equinoccio de otoño, cuando el fotoperíodo comenzó a disminuir. Las hojas comenzaron a expandirse a finales de la estación lluviosa, y las plantas alcanzaron 70 % de su área foliar máxima al inicio de la estación seca, manteniendo las hojas durante todo el período seco. La caída de hojas tuvo lugar gradualmente durante los primeros tres meses de la estación lluviosa. La producción y caída de hojas mostró alta sincronía, estando el número de hojas relacionado con la disponibilidad de luz. La producción máxima de hojas coincidió con picos en la radiación a mediados de la estación seca. La disminución del fotoperíodo induce alta sincronía en producción de botones florales en especies del bosque seco, pero este es el primer estudio que reporta la inducción de producción de hojas debido a la disminución en el fotoperíodo.
Palabras clave: inducción fotoperiódica, fenología inversa, Bonellia nervosa, Parque Nacional Santa Rosa, Bosque Tropical Seco.
References
Aide, T.M. 1992. Dry season leaf production: an escape from herbivory. Biotropica 24: 532-537. [ Links ]
Angulo-Sandoval, P. & T.M.Aide. 2000. Leaf Phenology and leaf damage of saplings in the Luquillo Experimental Forest, Puerto Rico. Biotropica 32: 415422. [ Links ]
Augspurger, C.K. 1983. Phenology, flowering synchrony, and fruit set of six neotropical shrubs. Biotropica 15: 257-267. [ Links ]
Barone, J.A. 1998. Effects of light availability and rain fall on leaf production in a moist forest in central Panama. J. Trop. Ecol. 14: 309-321. [ Links ]
Bazzaz, F.A. & R.W. Carlson. 1982. Photosynthetic acclimation to variability in the light environment of early and late successional plants. Oecologia 54: 313-316. [ Links ]
Borchert, R. 1983. Phenology and control of flowering in tropical trees. Biotropica 15: 81-89. [ Links ]
Borchert, R. 1994. Soil and stem water storage determine phenology and distribution of tropical dry forest trees. Ecology 75: 1437-1449. [ Links ]
Borchert, R. 2000. Organismic and environmental control of bud growth in tropical trees, p. 87-107. In J.D. Viemont & J. Crabbé (eds.). Dormancy in Plants. CABI International, Wallingford, Oxfordshire, United Kingdom. [ Links ]
Borchert, R., S.A. Meyer, R.S. Felger & L. Porter-Bolland. 2004. Environmental control of flowering periodicity in Costa Rican and Mexican tropical dry forests. Global Ecol. Biolgeogr. 13: 409-425. [ Links ]
Borchert, R., S.S. Renner, Z. Calle, D. Navarrete, A. Tye, L. Gautier, R. Spichiger & P. von Hildebrand. 2005. Photoperiodic induction of synchronous flowering near the Equator. Nature 433: 627-629. [ Links ]
Breslow, N.E. & D.G. Clayton. 1993. Approximate inference in generalized lineal mixed model. J. Am. Statist. Assoc. 88: 9-25. [ Links ]
Bullock, S.H. & J.A. Solís-Magallanes. 1990. Phenology of canopy trees of a tropical deciduous forest in Mexico. Biotropica 22: 22-35. [ Links ]
Chazdon, R.L., R.W. Pearcy, D.W. Lee & N. Fetcher. 1996. Photosynthetic responses of tropical forest plants to contrasting light environments, p. 5-55. In S.S. Mulkey, R.L. Chazdon & A. Smith (eds.). Tropical forest plan ecophysiology. Chapman & Hall, New York, USA. [ Links ]
Clark, D.A. & D.B. Clark. 1994. Climate-induced annual variation in canopy growth in a Costa Rican tropical rain forest. J. Ecol. 82: 865-872. [ Links ]
Clark, D.A., S.C. Piper, C.D. Keeling & D.B. Clark. 2003. Tropical rain forest tree growth and atmospheric carbon dynamics linked to interannual temperature variation during 19842000. PNAS 100: 5852-5857. [ Links ]
Croat, T.B. 1969. Seasonal flowering behavior in Central Panama. Ann. Missouri Bot. Gard. 56: 295-307. [ Links ]
Croat, T.B. 1975. Phenological behavior habit and habit classes on Barro Colorado Island (Panama Canal Zone). Biotropica 7: 270-277. [ Links ]
Daubenmire, R. 1972. Phenology and other characteristics of the tropical semideciduous forest in Northwestern Costa Rica. J. Ecol. 60: 147-170. [ Links ]
Dawson, T.E. & J.S. Pate. 1996. Seasonal water uptake and movement in root systems of Australian phreatophytic plants of dimorphic root morphology: a stable isotope investigation. Oecologia 107: 13-20. [ Links ]
Dupuy, N.C. & B.L. Dreyfus. 1992. Bradyrhizobium populations occur in deep soil under the leguminous tree Acacia albida. Appl. Environ. Microbiol. 58: 2415-2419. [ Links ]
Elliott, S., P.J. Baker & R. Borchert. 2006. Leaf flushing during the dry season: the paradox of Asian monsoon forests. Global Ecol. Biogeogr. 15: 248-257. [ Links ]
Frankie, G.W., H.G. Baker & P.A. Opler. 1974. Comparative phenological studies of trees in tropical wet and dry forest in the lowlands of Costa Rica. J. Ecol. 62: 881-919. [ Links ]
Givnish, T.J. 1988. Adaptation to Sun and Shade: A Wholeplant Perspective. Aust. J. Plant Physiol. 15: 63-92. [ Links ]
Gómez, P. & L. Fournier. 1996. Fenología y ecofisiología de dos poblaciones de Tabebuia rosea ("Roble de Sabana") en Costa Rica (Bignoniaceae). Rev. Biol. Trop. 44: 61-70. [ Links ]
Grace, J., J. Lloyd, J. McLntyre, A.C. Miranda, P. Meir, H.S. Miranda, C. Nobre, J. Moncrieff, J. Massheder, Y. Malhi, I. Wright & J. Gash. 1995. Carbon dioxide uptake by an undisturbed tropical rain forest in southwest Amazonia, 1992 to 1993. Science 270: 778-784. [ Links ]
Hamann, A. 2004. Flowering and fruiting phenology of a Philippine submontane rain forest: climatic factors as proximate and ultimate causes. J. Ecol. 92: 24-31. [ Links ]
Holbrook, M.N., J.L. Whitbeck & H.A. Mooney. 1995. Drought responses of neotropical dry forest trees, p. 242-276. In S. H. Bullock & H.A. Mooney (eds.). Seasonally dry tropical forests. Cambridge, England. [ Links ]
Janzen, D.H. 1967. Synchronization of sexual reproduction of trees within the dry season in Central America. Evolution 21: 620-637. [ Links ]
Janzen, D.H. 1970. Jacquinia pungens, a heliophile from the understory of deciduous forest. Biotropica 2: 112-19. [ Links ]
Janzen, D.H. 1983. Jacquinia pungens, p. 265-267. In D.H. Janzen (ed.). Costa Rican Natural History. The University of Chicago, Chicago, Illinois, USA. [ Links ]
Knudsen, J.T. & B. Ståhl. 1994. Floral odours in the Theophrastaceae. Bioch. Syst. Ecol. 22: 259-268. [ Links ]
Lieberman, D. & M. Lieberman. 1984. The causes and consequences of synchronous flushing in dry tropical forest. Biotropica 16: 193-201. [ Links ]
Mulkey, S.S., S.J. Wright & A.P. Smith. 1993. Comparative physiology and demography of three Neotropical forest shrubs: alternative shade-adaptive character syndromes. Oecologia 96: 526-537. [ Links ]
Oberbauer, S. 1985. Plant water relations of selected species in wet and dry tropical lowland forest in Costa Rica. Rev. Biol. Trop. 33: 137-142. [ Links ]
Opler, P.A., G.W. Frankie & H.G. Baker. 1980. Comparative Phenological Studies of Treelet and Shrub Species in Tropical Wet and Dry Forests in the Lowlands of Costa Rica. J. Ecol. 68: 167-188. [ Links ]
Pearcy, R.W., O. Björkman, M.M. Caldwell, J.E. Keeley, R.K. Monson & B.R. Strain. 1987. Carbon gain by plants in natural environments. Bioscience 37: 21-29. [ Links ]
Raich, J.W., E.B. Rastetter, J.M. Melillo, D.W. Kicklighter, P.A. Steudler, B.J. Peterson, A.L. Grace, B. Moore & C.J. Vorosmarty. 1991. Potential net primary productivity in South America: application of a global model. Ecol. Appl. 1: 399-429. [ Links ]
Reich, P.B. 1995. Phenology of tropical forest: patterns, causes and consequences. Can. J. Bot. 73: 164-174. [ Links ]
Reich, P.B. & R. Borchert. 1984. Water stress and tree phenology in a tropical dry forest in the lowlands of Costa Rica. J. Ecol. 72: 61-74. [ Links ]
Rice, W.R. 1989. Analysing tables of statistical test. Evolution 43: 223-225. [ Links ]
Rivera, G. & R. Borchert. 2001. Induction of flowering in tropical trees by a 30-min reduction in photoperiod: evidence from field observations and herbarium collections. Tree Physiol. 21: 201-212. [ Links ]
Rivera, G., S. Elliot, L.S. Caldas, G. Nicolossi, V.T.R. Coradin & R. Borchert. 2002. Increasing day-length induces spring flushing of tropical dry forest trees in the absence of rain. Trees 16: 445-456. [ Links ]
Rojas, K. 2001. Fenología de la copa y del sistema de raíces finas y relaciones hídricas de Enterolobium cyclocarpum (Guanacaste) un árbol de brotación temprana en el bosque tropical seco. Thesis M. Sc., University of Costa Rica, San José, Costa Rica. [ Links ]
Roupsard, O., A. Ferhi, A. Granier, F. Pallo, D. Depommier, B. Mallet, H.I. Joly & E. Dreyer. 1999. Reverse phenology and dry season water uptake by Faidherbia albida in an agroforestry parkland of Sudanese West Africa. Funct. Ecol. 13: 460-472. [ Links ]
SAS. 1994. JMP users guide. SAS Institute, Cary, North Carolina, USA. [ Links ]
Schultz, J.P. 1960. Ecological studies in Northern Suriname. Nord-Hollandse Uitgeversmaatschappij, Amsterdam, Holland. [ Links ]
Sobrado, M.A. 1986. Aspects of tissue water relations and seasonal changes of leaf water potentials components of evergreen and deciduous species coexisting in tropical dry forests. Oecologia 68: 413-416. [ Links ]
Ståhl, B. 1989. A synopsis of Central American Theophrastaceae. Nord. J. Bot. 9: 15-30. [ Links ]
Ståhl, B. & M. Källersjö. 2004. Reinstatement of Bonellia (Theophrastaceae). Novon 14: 115-118. [ Links ]
Tosi, J.A. 1969. Mapa ecológico, República de Costa Rica: según la clasificación de zonas de vida del mundo de L.R. Holdridge. Centro Científico Tropical, San José, Costa Rica. [ Links ]
Wright, S.J. 1992. Seasonal drought, soil fertility and the species density of tropical forest plant communities. TREE 7: 260-63. [ Links ]
Wright, S.J. & C.P. van Shaik. 1994. Light and the phenology of tropical trees. Amer. Nat. 143: 192-199. [ Links ]
Wright, S.J., C. Carrasco, O. Calderón & S. Paton. 1999. The El Niño Southern Oscillation, variable fruit production, and famine in a tropical forest. Ecology 80: 1632-1647. [ Links ]
Wright, S.J. & F.H. Cornejo. 1990. Seasonal drought and the leaf fall in tropical forest. Ecology 71: 1165-1175. [ Links ]
Wycherley, P.R. 1973. The phenology of plants in the humid tropics. Micronesica 9: 75-96. [ Links ]
Zotz, G. & K. Winter. 1996. Diel patterns of CO2 exchange in rainforest canopy plants, p. 89-113. In S.S. Mulkey, R.L. Chazdon & A.P. Smith (eds.). Tropical forest ecophysiology. Chapman and Hall, New York, U.S.A. [ Links ]