Introducción
In 2012, the Government of Costa Rica defined a challenging goal - Costa Rica wants to be carbon neutral by 2021. Converting the countries energy system fully to renewable energy sources until 2020 was one of the most important objectives of the energy policy and economy in Costa Rica since then. Although this goal will not be achieved until 2021, the current (in 2018) new elected government has stated that all efforts to increase the share of renewable energies should be continued and strengthened through appropriate instruments to promote R&D and the implementation of new and innovative technologies in this field. The conditions in the country are extremely good for this by the natural abundance of the wind, solar, hydro, biomass and geothermal energy resources. Within this “renewable energy mix” bioenergy has an important role since the biomass, as a natural stored solar energy, can be used very flexible and contribute to the energy supply security in all the energy sectors such as power, heat and the mobility sector. Globally, biomass is the most important renewable energy (RE) source in use today. RE contribute approx. 14 % to the global primary energy consumption and biomass remained steadily with share of 10% of the total primary energy consumption since 2000 (2);, while an increase of the total primary energy consumption by approx. 30 % was registered. One of the main drivers in the climate change policy is the development of advanced RE technologies to generate heat and power and substitute fossil fuels in the transportation sector.
Caused by the favorable climate conditions, in combination with a highly agricultural economic structure in Costa Rica, the use of biomass as energy source is a promising opportunity to support the full transition of the country from fossil fuels to renewable energy.
Agricultural residues as energy source
In many developing countries, the food production is much more important than in Europe and competes to the production of energy crops (5);. One of the advantages of the utilization of agricultural residues as an energy source is that they do not compete directly to the food (production of food) or feed sectors. Other advantages are the reduction of greenhouse gases by the substitution of fossil fuels, a possible increase in added value (income generation and rural development) in rural areas and the reduction of environmental problems (e.g. harmful emissions from open-field burning or open-air dumping of the biogenious wastes) caused by improper disposal of such residues.
Many agricultural residues can potentially be counted as beneficial resources if they are managed sustainably. The raw material base is diverse in form of e.g. stalks, straw, leaves, roots, husk or seed shells and farmand animal husbandry waste. The properties of these biomass sources vary within a wide range. The most significant difference is between those residues that are predominantly dry (such as straw) and more suitable for thermo-chemical conversion routes (e.g. combustion, gasification, pyrolysis) and those that are wet (such as animal slurries) and more suitable for biological conversion routes (e.g. biogas production) (4);. This paper will focus on substrates and technologies which will be most suitable for biogas production. An overview about methane yields gained from different tropical substrates after approx. 28 days of batch digestion experiments under mesophilic conditions (adopted from different studies) is given in table 1 and shows that especially the methane yields of tropical fruits are comparable with the high yield usually produced by using energy crops such as maize in Europe (5);.
Table 1 Methane (or biogas yields from different tropical substrates *1 adopted from (5); and *2 adopted from (6);, *3 adopted from (7);, digestion experiments OPEFB for 30 days (a) = untreated substrate, (b) after pretreatment with NaOH for 60 min.
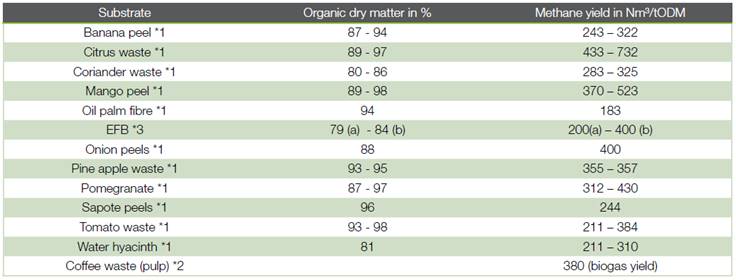
Case study for pineapple and banana residues in Costa Rica
The government of Cost Rica intends to facilitate a comprehensive national plan for the management of solid waste through appropriate legislations and regulations (9);, such as a “law for the integrated management of residues” (10);. As recommended by Ben-Haddej et al. (2010) (10);, for the municipal solid waste, market-based instruments (MBIs) such as “eco-labelling”, “pay-asyou- throw”, and “deposit-refund” can contribute to the implementation of this law.
Meanwhile, Costa Rica is very concern about the agrowaste management in a sustainable way and has set rules for the integrated management of residues (9);. On the other hand, the achievement in neutral carbon emissions from electricity sectors by 2021 can promote the efficient use of the residues as a by-product, rather than producing wastes for the country. The total annual wastes produced from agricultural sectors are very considerable. Unsustainable management of these wastes will have adverse socio-economic and environmental consequences. The successful implementation of sustainable use of the fruit wastes from banana and pineapple depends on the availability of resources and infrastructure, and the multicriteria assessment with respect to the related economic, social, and environmental consequences.
According to the INEC national census, 2015, the total allocated land use to the permanent crops in Costa Rica is around 353,732 hectares, and banana and pineapple cultivations account for almost 15 % and 11 % of the permanent crop lands (8);.
While the pineapples are scattered in the provinces of Alajuela, Heredia, Puntarenas, Guancaste and Limon, 80% of banana is concentrated in Limon. The Spatial distribution of all permanent crops and the percentage concentration of pineapple and banana cultivations are illustrated in figure 2 and table 2.
Digestion experiments with banana residues
The possibility of biogas production from banana residues, specifically from rachis and green unripe bananas, was tested in small scale stirred tank reactors (CSTR) reactors, at mesophilic conditions of 35-38 °C. In addition, the effects of co-digestions of these two substrates as well as whey from a cheese production process was tested by observing the behavior of the FOSTAC meaning the volatile fatty acids and the total inorganic carbonate of different combinations of the substrates. The three substrates (rachis, banana and whey) were investigated in lab-scale batch experiments at 36 °C to determine their maximum biogas production potential.
Figura 2. Distribución espacial de cultivos permanentes en las provincias de Costa Rica (8)
Semi-continuous experiments were conducted to examine the biomethanation of different mixtures of the substrates using different organic loading rates (OLR) between 2.0 - 2.5 kgVS/(m³d). The anaerobic digestion was carried out in completely CSTR at mesophilic conditions (35 - 38 °C). The experiment consisted of five individual reactors with a volume of five gallons (18.93 liter) each. They had the same physical features in order to achieve comparable results. The substrates were analyzed on their TS- and VS contents (table 4) and on their elemental composition (table 5).
The ratio was also used to control the process by lowering and rising the OLR in accordance to (11);. The reactors were initially filled with 14 liters of sludge from a cow and swine manure digester. Then the feeding was weekly increased by 0.5 until the desired OLR was achieved. From there on, daily measurements of the pH, the gas production as well as the gas quality was used to keep track of the process development and every four days the FOSTAC was measured. The FOSTAC was used to increase or decrease the feeding in accordance to the findings of (11);.
For the batch system an extra DM-VS-analysis (table 7) of the freshly grinded substrate was conducted. A mix sludge from a cow and swine manure digester was used as inoculum. , The resulting amount of feed for the batch experiments was 495.9 g Rachis, 102.9 g Banana and 282.2 g Whey, always in combination with 1000 g seeding sludge and a VS addition of 17.34 g. In this manner, three reference systems with the seeding sludge in use, three with seeding sludge and rachis, three with seeding sludge and banana as well as three with seeding sludge and whey were set up, making a total of 12 digesters.
The following five variations of substrate mixtures (percentage based on dry matter in table 6) were tested in the semi continuous reactors.
Figura 3. Producción de biogas a partir de banano en el sistema.
Figura 4. Producción de biogas a partir de suero en el sistema.
Batch experiment with rachis
Rachis produced a total average 8948.94 ml of Gas during a digestion time of 18 days. After that the production dropped drastically and almost died down ton 17th of October (the 7th day) but had another small peak of 312.6 ml. Until the 26 th of October (last day), the control reactor fed only with seeding sludge and produced an average of 1631.38 ml (Figure 4). Therefore, from the rachis decomposition of 7317.56 ml accumulated gas, 5698.7 5ml (78 %) were already produced within the first four days. The CH4 content was on average 62.69 %.
The gas yield from rachis decomposition is calculated following equation 1:
According to the experiments, rachis and banana are suitable substrates for the biogas production. Batch experiments resulted in average gas yields of 0.422 m³/kgVS with 62,69% CH4 for rachis and 0.343 m³/kgVS with 61,46% CH4 for green unripe bananas. However, they are difficult substrates due to their chemical composition and morphological characteristics.
Figura 6. Producción de biogás y concentración de CH4, exp semicontinua de 100% de rachis.
Figura 7. Producción de biogas y concentración de CH4, exp semicontinua de 66 % de rachis, 33 % plátano.
Figura 8. Producción de biogás y concentración de CH4, exp semicontinua.
de 100% de rachis.
Figura 9. Producción de biogas y concentración de CH4, exp semicontinua
de 50 % de rachis, 50 % plátano.
Batch experiment with banana
The digestion time of banana was 16 days. In this time an average of 5956.69 ml gas accumulated with an average CH4 concentration of 61.46 %. Calculating the gas yield (equation 1 above), the YG was 0.343 m³/kgVS (figure 4).
Batch experiment with whey
The course of the digestion of whey was very different compared to rachis and banana. The daily gas production showed several peaks with low yields interrupted by decreased gas productions. In addition, the gas production was measured for a total time of 31 days. The gas production did not lower exponentially but occurred in waves. In total, the average gas production by whey was 8000.88 ml, leading to a gas yield of YG=0.461m³/kgVS and having an average CH4 concentration of 64.74 % (figure 5).
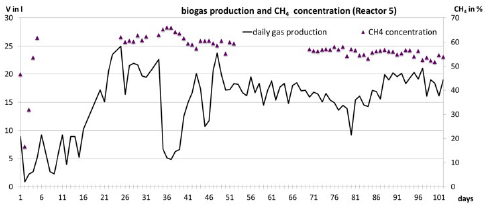
Figure 10 Biogas production and CH4 concentration, semi continuous exp. of 50 % rachis, 25 % banana, 25 % whey.
Figura 10. Producción de biogás y concentración de CH4, exp semicontinua de 50 % de rachis, 25 % plátano, 25 % suero.
Figura 11. Comparación de ecoeficiencia de dos valorizaciones de residuos en Costa Rica.
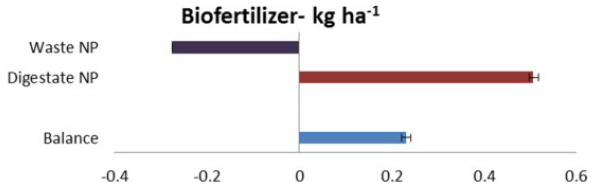
Figure 12 Life cycle assessment of waste valorization of banana and pineapple in Costa Rica. (Reproduced from (12);).
Figura 12. Evaluación del ciclo de vida de valorización de residuos de banano y piña en Costa Rica. (Reproducido de (12);).
Semi continuous experiments
The semi continuous digestion experiments were carried out in the 5 identical lab scale CSTR reactors described previously. The initial values for the experiments resulted from calculations from TS-VS analysis (table 4) and the feed compositions (table 6). Reactor 1 and 2 started with an OLR of 2 and a HRT of 22 and 30 days, respectively. Reactors 3, 4 and 5 started with an OLR of 2.5 and a HRT of 27, 28 and 25. The daily amount of feed was 628 g, 462 g, 506 g, 483 g and 549 g. If the FOSTAC showed signs of reactor instability, the feeding was lowered to prevent acidification. The initial system parameters are shown in table 8 and the results of the experiments are shown in figure 7 , 8, 9, 10 y 11.
The low amount of dry matter in rachis leads to low HRTs in case of a high OLR, but the fibrous structure leads to a slow degradation. System one only worked stable with an OLR of solemnly 1 and for reactor 2, the perfect amount of feed was never established. The gas production in both systems added up to about 8 liters on average with low methane concentrations of 49 % for system 1 and 50 % for system 2. The increase of banana in the feed let to a shift in the point of FOSTAC rising to later stages in the experiment. This effect can be seen very well within reactor 2, 3 and 4. These three reactors showed that a percentage of over 50 % bananas or the additional use of whey as co-substrate were fitted better for a continuously operated system.
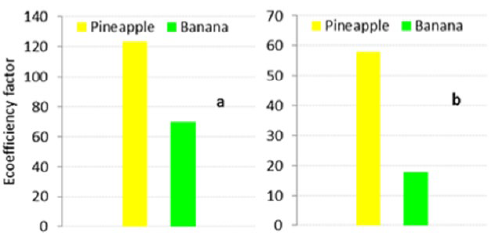
Figure 13 Life cycle ecoefficiency of using biofertilizers “a” versus to not using biofertilizers “b” for the two waste-valorization systems.
Figura 13. Ecoeficiencia del ciclo de vida del uso de biofertilizantes “a” versus no utilizar biofertilizantes “b” para los dos sistemas de valorización de residuos.
Ecoefficiency of waste valorizations of banana and pineapple in Costa Rica
In the study, the ecoefficiency refers to the ratio of the energy as a value-added factor to the GHG emissions as a cost factor. The higher is the ratio, the more ecoefficient is the system. System is the waste valorizations of these residues through anaerobic biogas digestions (figure 12). Data used for the ecoefficiency analysis is obtained from a previous study (12);. The potential wastes and the major assumptions and parameters for biogas and fertilizer productions are documented in table 3.
Life cycle assessment of waste Valorization of banana and pineapple in Costa Rica
The use of fruit wastes will produce electricity and reduce the energy cost of chemical fertilizers in the field. In the life cycle perspective, the overall GHG emission reductions and energy savings in fig. 12, indicate that the net values of the two indicators are positive for both waste fruits.
Apart from the life-cycle profiles of GHG emissions and energy savings in fig. 12, the ecoefficiency which is the ratio of value added (energy) to the costs (GHG emissions), in Table 9, provides a single score to compare easily the two systems above. Under the assumptions and considerations in this study, the waste valorization of pineapple could be 1.8 times greater than banana’s.
Removing the banana residues for biogas would not decrease the nutrient availability as long as the biogas digestate are recycled back to the field. However, a significant credit assigned to pineapple in terms of the analyzed ecoefficiency is due to the inherent energy savings from the displaced chemical fertilizers.
Impact of biofertilizers on estimated ecoefficiency
The study has considered the digestate as a value added product rather than a waste. The contribution of digestate to the energy value added is shown in Table 9. However, ignoring the credit of biofertilizers would increase the cost of life cycle GHG emissions because of the increased chemical fertilizer inputs for the banana to compensate for the nutrient deficiency of the removed residues from the field. Therefore, a scenario is created to show the magnitude of the variation in the estimated ecoefficiency as shown in fig. 13. It should be noted that contrary to the pineapple residues which are usually piled in the open space, banana residues are left on the field for the soil fertilization.
Clearly, a significant decrease of the ecoefficiency is expected when ignoring the value added energy savings from biofertilizers. In scenario “b”, the ecoefficiency of pineapple would decrease by ~53%, whereas for banana, because of the increased costs (i.e. GHG emissions), the ecoefficiency would be lower by 74 % compared to the base case (scenario “a” in fig. 13).
Conclusions
Technically, around 6 million-t of fruit wastes from banana and pineapple production could contribute to not only GHG emission reductions, but also to produce energy value-added in Costa Rica. The waste-valorization through anaerobic biogas would be beneficial in terms of the life-cycle ecoefficiency which is the ratio of the lifecycle value-added energy savings to the GHG emission costs. A comparison between the ecoefficiency factors of pineapple and banana indicates that pineapple would win over banana by a magnitude factor of 1.8. Ignoring the value-added of biogas digestate (biofertilizers) would significantly decrease the analyzed ecoefficiencies of the both systems. The results of this study may be noted by the energy developers, waste managers, and farmers, and further, contribute to achieving the carbon neutral target of Costa Rica in electricity sectors.