Introduction
Photobiomodulation therapy is a non-invasive therapy based on photochemical interactions between infrared laser light energy and biological tissues (1). The chromophore theory explains how light energy is absorbed within cells (2). Various lines of research propose the enzyme cytochrome C oxidase and calcium channels as photoreceptors and transducers of photosignals, which, after being photoactivated, produce an increase in the potential of the mitochondrial membrane, nitric oxide (NO), ATP and modulate the levels of calcium (3,4). Furthermore, photoactivation increases cell proliferation and migration and promotes the synthesis of new proteins (5).
The effect of photobiomodulation has been studied in fibroblast cells, endothelial cells, and osteoblasts. Photobiomodulation has been applied and investigated for different purposes within the various dental specialties and medical sciences in general (1, 3). Standing out in the repair and healing of wounds, in the treatment of alterations of the immune system, in the relief of muscle fatigue, in pain therapy, and in various conditions within the bone (2, 6,7,8,9).
Bone tissue is a specialized type of connective tissue made up of osteoblasts, osteoclasts, and osteocytes, which under physiological conditions are in a constant remodeling mechanism that involves bone formation and bone resorption (10). In a surgical approach to bone tissue, for instance, an osteotomy, the formative mechanisms are mainly triggered because the need for effective repair, with osteoprogenitor cells and osteoblasts playing a cardinal role (11). It has been found, within bone tissue under repair, that the use of an infrared laser increases the alteration of osteoblasts and inhibits osteoclasts, upregulating bone growth factors and NO, maintaining an adequate tissue environment for the reparative process (9, 12). Several studies have shown that the photo-biomodulation therapy improves bone healing by promoting mitochondrial respiration and ATP synthesis, in addition to stimulating angiogenesis (9,13,14,15). Many investigations highlight that the use of low-intensity infrared laser is the most effective in photobiomodulation therapy (PBMT) on bone tissue (16). It is known that infrared light is captured by the cytochrome C oxidase enzyme responsible for increased ATP production, which produces the differentiation of mesenchymal cells into preosteoblasts and then into osteoblasts at the bone tissue level (14).
There is currently a remarkable consen- sus on the benefits that PBMT would produce on bone repair. However, it remains unclear what the best parameters to use in PBMT would be, such as: laser wavelength, energy, number of sessions, among other parameters (17). Finding that some investigations do not unquestionably demonstrate the benefits of PBMT, especially because the optimal level of the photobiomodulatory stimulus is not yet clearly defined, finding great variability of results both in investigations in humans and in animal models (2, 18,19,20,21,22,23,24).
Various energy levels are used in the literature, finding application values from 0.84J to 10J or even more, where it is observed that the application of higher energy levels obtain better results (18,20,23,24,25,26,27,28). In addition, the studies apply various wavelengths of the infrared laser; nonetheless, the vast majority use the Aluminum Gallium Arsenide (AlGaAs) laser with an active medium, with a wavelength of 808 nm (18, 19, 21,22,23,24, 26,27,28,29).
Due to the importance of photobiomodulation in the repair and stimulation of bone tissue, and the need to continue in the search for the standar- dization of energy parameters this research aimed to determine the Effect of PBMT with energies of 1 J and 3 J using an Aluminum Gallium Arsenide (AlGaAs) laser, with a wavelength of 808 nm, on bone repair in a tibial osteotomy model of male produce greater bone repair than that of 1 J.
Materials and methods
Ethical Considerations
In vivo experimental preclinical study approved by the research ethics committee of the Universidad Científica del Sur with dictum 179-2018-POS99. The recommendations of the ARRIVE guide (Animal Research: Reporting of In Vivo Experiments) were followed. 36 male albino rats of the Holtzman strain, 8 weeks old and weighing 300g+/-50g, were used. The animals were housed in the institution's animal facility where they received food and water ad libitum with alternating 12-hour light and dark cycles and with controlled humidity and temperature.
The 36 mice were randomized, using the function =RANDOM.BETWEEN (1, 36) of the Excel program, into 6 groups of 6 animals each: Groups A1 and A2 underwent osteotomy of the tibial bone and was irradiated with 1 J of energy, being sacrificed at 15 and 30 days, respectively. Groups B1 and B2 underwent osteotomy of the tibial bone and was irradiated with 3 J of energy, being sacrificed at 15 and 30 days, respectively. Control groups C1 and C2 underwent osteotomy alone and were also sacrificed at 15 and 30 days, respectively (Table 1).
Surgical protocol
As a sedoanalgesia protocol, which was applied to all animals, 100mg/ml ketamine (Ket-A- 100 ®) was used at a dose of 30mg/kg and xylazine of 20mg/ml (DORMI-XYL® 2) at a dose of 6mg/kg, both drugs were administered intra- peritoneally (30). It was waited until the effects of the drugs were observed. Then, using body depilatory cream (Depile - Aloe Vera and Argon, Veet®), the hair was removed from the left hind legs of the animals. Disinfection of the surgical area with 10% povidone iodine was followed and the length of the ventral aspect of the left tibia of each rat was measured. A midpoint was located where a longitudinal incision was made along the axis of the leg with a No.15 scalpel blade (Elite ® - Surgical Blades, China). The skin, subcutaneous tissue, muscle layer and periosteum were separated with a Williger periostotome (Carl Martin®, Germany). When the tibia was clearly exposed, an osteotomy (low speed motor, at 20000 RPM, NSK®, Japan) was performed in a circular shape of 2mm in diameter with round tungsten carbide surgical drills (PRIMA CLASSIC®, United Kingdom), which had the same diameter as the defect created (31). It was irrigated with abundant sterile saline solution. Immediately after osteotomy, groups A1, A2, B1 and B2 were irradiated with an infrared laser (THERAPY XT-DMC®, Brazil). The wounds were closed with absorbable polyglycolic acid suture (Vycril 4/0 MR 25. Ethicon®, China).
Laser protocol
Low level laser (light) therapy (THERAPY XT - DMC®, Brazil) was used with an active medium of Gallium-Aluminum Arsenide (AsGaAl), with a wavelength of 808nm, power of 100mW, continuous mode. Due to the fact that in the different investigations the energy parameters are not standardized, in the present work it was chosen to use low intensity energy in order to expose the biological tissues as little as possible. Energy of 1 J was used for groups A1 and A2, and 3 J for groups B1 and B2. The laser was applied only once, punctually and in contact in the osteotomy area in all irradiated groups.
Evaluation of bone repair
After surgery, groups A1, B1 and C1 were sacrificed at 15 days and groups A2, B2 and C2 at 30 days. All groups were subjected to a protocol of euthanasia with pentobarbital sodium 65mg/ml (Penta-Hypnol®) at an overdose of 150mg/kg intraperitoneally (30). We waited for the vital signs to disappear and then proceeded to separate the tibiae from the specimens, dissecting them with a margin of approximately 5mm from the surgically intervened region. The samples were housed in bottles with 10% formalin. They were subsequently fixed in 10% phosphate buffered saline for 24 hours. It was then decalcified with ethylenediaminetetraa- cetic acid at room temperature for 4 to 5 weeks (32). Finally, the samples were dehydrated with ethanol and embedded in paraffin. By using a microtome (Kedee KD-2258®, China) the histological slides were obtained and then stained with hematoxylin and eosin. A histological slide was obtained from each sacrificed animal. The slides were evaluated by a specialist in an optical microscope (Micros MCX100 LCD Crocus II®, Germany) using a total magnification of 400x. Bone repair was determined by cell count of osteoblasts and osteocytes in the intervened area, taking 4 fields from this area at random and counting on an hourly basis (10,33). In general, the analysis consisted in that 4 different and characteristic areas were photographed from each sheet within the surgically intervened area. After the cell count of the 4 photographed areas, the average of the number of cells was obtained, which was the value of osteoblasts and osteocytes that was considered for each sheet.
Statistic analysis
The data processing and analysis was carried out using the statistical program SPSS v23. Cell counts are presented as the mean ± standard deviation. Due to the normality of the data verified with Shapiro_Wilk and its homocestacity confirmed by Levene's test, the Student's t test, the ANOVA test and the Tukey test were applied. The level of significance considered was 5%.
Results
Figure 1 shows the histological process of bone repair at 14 days and 30 days after surgery. It is observed that osteoblasts and immature forms of bone trabeculae predominate at 15 days (Figure 1. A, C and E); on the other hand, osteocytes and better bone organization become more evident at 30 days (Figure 1. B, D, F).
Table 2 shows the means ± their standard deviation of the 6 experimental groups. Using the Student's t-test, the number of osteoblasts and osteocytes between 15 and 30 days were compared within the groups that received the same treatment. A significant statistical difference was found in the osteoblast count between groups A1, B1 and C1 vs A2, B2 and C2, respectively, and between groups B1 and C1 vs B2 and C2 in the case of osteocytes.
By means of the ANOVA test, the experimental groups were compared with each other, grouping them according to the time at which they were sacrificed. Table 3 shows the comparisons made at 15 days, between the osteoblast count and, on the other hand, between the osteocyte count, finding significant differences in both cases (p<0.001). Table 4 also makes the same compari- sons between the same cell groups, finding signi- ficant differences (p<0.05). As in both tables the results of the ANOVA tests were statistically significant the Tukey post hoc test was applied.
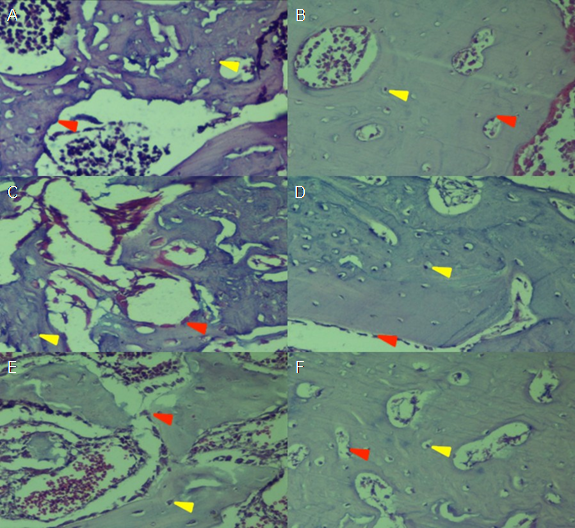
Figure 1. Representative photomicrograph of the intervened areas in the experimental groups (400x magnification). A, C and E: Histological plates at 15 days of groups A1, B1 and C1, respectively. Initial trabecular bone formation is observed; red dates osteoblasts, yellow dates osteocytes. B, D and F: Histological plates at 30 days of groups A2, B2 and C2, respectively. A greater quantity of areas occupied by the bone trabeculae is observed; red dates osteoblasts, yellow dates osteocytes
Table 2 Comparison of the number of osteoblasts and osteocytes between 15 and 30 days.
- | Evaluated Cell | 15 day group | Average | 30 day group | Average | p-value† |
---|---|---|---|---|---|---|
±: Standard deviation †: Student's t-test | Osteoblast | A1 | 61.4 ± 9.6 | A2 | 20.9 ± 4,4 | <0.001 |
- | - | B1 | 26.2 ± 5.3 | B2 | 17.9 ± 4,7 | 0.009 |
- | - | C1 | 68.4 ± 13.5 | C2 | 29.2 ± 4,1 | <0.001 |
- | - | A1 | 38.0 ± 4.0 | A2 | 43.8 ± 8,9 | 0.173 |
- | Osteocyte | B1 | 48.7 ± 10.0 | B2 | 34.9 ± 4,7 | 0.022 |
- | - | C1 | 24.7 ± 7.9 | C2 | 45.8 ± 4,4 | 0.004 |
Table 3 Comparison of the experimental groups at 15 days.
- | Osteoblast | - | Osteocyte | - |
---|---|---|---|---|
Group | Average | p-value† | Average | p-value† |
A1 | 61.4 ± 9.6ᴬ | <0.001 | 38.0 ± 4.0ᴬ | <0.001 |
B1 | 26.2 ± 5.3ᴮ | - | 48.7 ± 10.0ᴬ | - |
C1 | 68.4 ± 13.5ᴬ | - | 24.7 ± 7.9ᴮ | - |
±: Standard deviation.
†: Anova test.
A, B: Different letters indicate statistically significant differences according to Tukey's test.
Table 4 Comparison of the experimental groups at 30 days.
- | Osteoblast | - | Osteocyte | - |
---|---|---|---|---|
Group | Average | p-value† | Average | p-value† |
A2 | 20.9 ± 4.4ᴬ | 0.002 | 43.8 ± 8.9ᴬᴮ | 0.032 |
B2 | 17.9 ± 4.7ᴬ | - | 34.9 ± 4.7ᴬ | - |
C2 | 29.2 ± 4.1ᴮ | - | 45.8 ± 4.4ᴮ | - |
±: Standard deviation.
†: Anova test.
A, B: Different letters indicate statistically significant differences according to Tukey's test.
Discussion
In the present investigation, it was found that a greater number of osteoblasts was observed in all the experimental groups at 15 days compared to 30 days. The pattern found coincides with that reported by Asatoy et al. (19), who found, in a rat tibia osteotomy model, that the osteoblasts in the photobiostimulated groups with energy of 5 J/ cm2, 10 J/cm2, and 20 J/cm2 were higher in week 4 when comparing to the week 8 of evaluation.
In addition, Llapapasca et al. (18), who irradiating an infrared laser energy of 1 J, immediately after extraction in rats, found a significant amount of osteoblasts after 14 days of evaluation. In the initial stages of bone repair, osteoprogenitor cells differentiate into osteoblasts, which begin to proliferate and are responsible for forming the bone matrix composed mainly of collagen proteins, which indicates that these cells predominate in the initial stages of the reparative process (11). One of the effects of PBMT is the increase in Runt-related transcription factor 2 (Runx2) and osterix (osx), which are important early markers of osteoblast differentiation (14).
When comparing the 3 experimental groups with each other at 15 days, it was found that the number of osteoblasts was higher in groups A1 and in the control. Tim et al (24), found in a rat tibia osteotomy model that the effect of PBMT, using a laser with energy of 2.8 J, increased the amount of newly formed bone and the expression of TGF-β, which is the central player in bone homeostasis by inducing recruitment and proliferation of osteoblasts, in addition to increased type 4 morphogenetic protein, alkaline phosphatase (ALP), and Runx2. Moreover, in our study, it was observed that at 15 days, group B1 had fewer osteoblasts than the group irradiated with 1 J (group A1). It is possible that the dose of 3 J (group B1), was excessive and caused a decrease in osteoblastic differentiation, which, as noted, is the predominant bone cell type in the initial stages of the repair process. This finding agrees in general terms with that reported by Brito et al. (23), who evaluated the effect of 4J, 6J, and 10J PBMT on bone repair in a rat tibia repair model. They found that bone mineral density in the injured area was higher in 4J PBMT compared to higher energy therapies, for which they used radiographic analysis using Digora software to measure bone mineral density.
When comparing the 3 experimental groups with each other at 30 days, it was found that the amount of osteoblasts decreased in all groups, being lower in groups A2 and B2 compared to the control. It is known that as the bone matures in the reparative process, the osteoblasts gradually decrease in number (11). Based on this, all groups showed a similar reparative process, although this was slightly more accelerated in the irradiated groups, based on the lower number of osteoblasts found in these groups at 30 days vs. the control group. Brito et al. (21), who applied an osteotomy model in rodents, and Abdel Hamid et al. (22), who extracted premolars from a group of dogs, found through imaging means that the reparative process was evident in all the groups worked, but there was higher bone density when evaluating the groups with PBMT vs. the controls in the fourth week. Furthermore, Çırak et al. (20), when evaluating sockets of rats after extraction of their maxillary right incisors, found a more organized bone repair in the groups that received 10 J/cm2 and 6 J/cm2 over the control. The PBMT improves bone regeneration and formation through two consecutive phases: one with direct effects, where osteoblast proliferation is stimulated, bone growth factors are upregulated, and cytokines and osteogenesis factors are modulated; and one of the indirect effects where an adequate environment is created for bone formation that implies an increase in NO, which promotes vascularization and improves lymphatic circulation (9).
As the reparative process progresses, the osteoblasts become embedded in their own matrix which becomes calcified, the cells now taking the name of osteocytes, which are the mature cells of the bone responsible for maintaining their physiological functions (11). In our work, it was found that the number of osteocytes increased in groups A2 and in group C2 when compared with A1 and C1, respectively. This coincides with that observed by Atasoy et al. (19), who reported that in all their evaluated groups the number of osteocytes was higher in week 8 compared to week 4; None; None In our study, the groups irradiated with 3 J (groups B1 and B2) showed a different behavior since their number of osteocytes was higher at 15 days when compared to 30 days, which may suggest a delay or alteration in the remodeling process at higher doses of energy, which coincides with what was investigated by Brito et al. (23), who found that the dose of 10 J had a lower serum level of alkaline phosphatase (ALP), one of the most used bioche- mical markers of bone remodelings, that the dose of 6 J.
On the other hand, when comparing the number of osteocytes between the 3 experimental groups at 15 days, we found that the rodents with PBMT had a greater quantity of said cells compared to the control, suggesting a more accelerated maturation process of the bone in these groups. De Oliveira et al. (28), associated the effects of PBMT with increased expression of bone growth factor type 2, osteocalcin, and ALP, which are markers of bone cell differentiation and maturation. In addition, Abdel Hamid et al. (22), using cone beam computed tomography, evaluated alveolar bone repair after extraction in dogs at 21 days, finding higher bone density in the groups with PBMT compared to the control. On the other hand, when comparing the 3 working groups at 30 days, it was observed that groups A2 and group C2 showed a similar number of osteocytes; different from that found in group B2 irradiated group with 3 J, which had fewer of these cells than the control group. These findings would indicate that at 30 days there is a similar bone repair between the control group and the group exposed to 1 J, in addition to a better repair process of the control group C2 compared to the B2. This result found between group A2 and C2 coincides with that reported by Desai et al. (21), who, working on a rabbit femur osteotomy model, concluded that there was no difference in the bone repair process between the animals with PBMT and their controls; Besides, Atasoy et al. (19), studying the effect of PBMT on bone repair, found no significant differences in any of the evaluation times between the irradiated groups and the controls. On the other hand, it is appropriate to highlight that there are diverse experimental studies and systematic reviews, both in humans and in animals, which show that the application of infrared laser on bone repair is positive (1,2,8,14); Nevertheless, the methodologies and energy parameters used are very varied and it is still premature to suggest a standardized protocol. On the other hand, according to the review of the various antecedents, it was initially hypothesized that a greater energy in the PBMT would produce greater bone repair. But, the results indicated that the low dose produces better bone repair than the high energy doses based on the results of the histological analysis, where the main indicator in the initial stages of the reparative process is the osteoblast count and the main indicator in the advanced stages is the osteocyte count. As a limitation, it can be indica- ted that, although it is true, the cells evaluated in the microscopic analysis allow us to appreciate the biological process of bone repair, this evaluation, in future work, must be complemented with immunohistochemical and genetic analyses. All this may explain the differences found between our study and those that clearly report the benefits of the various energy levels that are applied in the PBMT at any time of evaluation.
Conclusion
The application of photobiomodulation therapy, by assessing the number of osteocytes and osteoblasts, improved bone repair after 15 days with the application of 1 J of energy with respect to the control; However, at 30 days, based on the parameters analyzed, that improvement in the reparative process was no longer evident due to the similar results found between the group irradiated with 1 J and the control, the response being even lower in the group irradiated with 3 J. These results indicate that low intensity photobiomodulation would have a better effect on bone repair.
Funding
This research did not receive any specific grant from funding agencies in the public, commercial, or not-for-profit sectors.
Conflicts of interest statement
There is no type of financial and nonfinancial conflicts of interest from the authors.
Author contribution statement
Conceptualization and design: J.C.M., E.A.S. and S.J.S.
Literature review: J.C.B., E.A.S.
Methodology and validation: J.C.B., E.A.S., S.J.S.,
E.R.R. and C.E.P.
Investigation and data collection: J.C.B., E.A.S., S.J.S., E.R.R. and C.E.P.
Data analysis and interpretation: J.C.B., E.A.S. and E.R.R.
Writing-original draft preparation: J.C.B., E.A.S., S.J.S., E.R.R. and C.E.P.
Writing-review & editing: J.C.B., E.A.S., S.J.S.,
E.R.R. and C.E.P.
Supervision: E.A.S.