Introduction
Disease-causing pathogens are more frequently reported in forest plantations, resulting in significant economic losses. Them melina tree (Gmelina arborea Roxb: Lamiales: Lamiaceae) is the second most planted forest species in Costa Rica, covering 18 000 hectares according to the last agricultural census (Instituto Nacional de Estadística y Censos (INEC), 2015). Breeding efforts have been dedicated to this tree species in the region over the past 30 years, which has reported genetic gains in growth and yield of over 25-30 % (Ávila-Arias, Murillo-Cruz & Murillo-Gamboa, 2015; Ávila-Arias, Murillo-Cruz, Murillo-Gamboa & Sandoval, 2015; Espitia et al., 2016; Hernández Castro et al., 2021; Hernández-Castro et al., 2021a; 2021b).
In the country, Melina plantations have a high incidence of the disease commonly referred to as ''trunk rot''. Since its initial appearance extensive research has been conducted to achieve better control and management of this disease (Arguedas et al., 2018; Murillo-Gamboa et al., 2016; Salas-Rodríguez et al., 2016; Wingfield & Robison, 2004). Currently, it is understood that the causal agent of this disease is the fungus Ceratocystis fimbriata Hell & Halst (Microascales: Ceratocystidaceae) sensu stricto (Méndez-Álvarez et al., 2020), commonly known as ''Ceratocystis wilt''.
C. fimbriata is a saprophytic fungus found in soils and acts as a primary pathogen on both angiosperms and gymnosperms (Harrington et al., 2014). Its current distribution covers tropical, subtropical, and temperate regions worldwide (Holland et al., 2019). Considered one of the most aggressive soil pathogens, it can survive on the surface of a wound without penetrating the vascular tissue for 7 to 15 days and it can persist on wood fragments for up to 3 months (Cordero Vega, 2017). Species within the genus Ceratocystis classified based on their genetic diversity and geographic range, where C. fimbriata being placed in the Latin American clade (Argôlo Magalhães et al., 2016; Méndez-Álvarez et al., 2020).
The fungus C. fimbriata has been identified infecting at least 31 species in 14 economically significant families (Holland et al., 2019; Valdetaro et al., 2015). In other regions, it affects crops such as coffee, mango, and cocoa (Holland et al., 2019; Valdetaro et al., 2015) as well as forest species including Gmelina arborea, Populus spp, Tectona grandis and Eucalyptus spp. (Ferreira et al., 2010; Valdetaro et al., 2019). In Costa Rica it has been observed in coffee, cacao, xanthosoma and melina forest plantations (Baker et al., 2003; Cordero Vega, 2017; Méndez-Álvarez et al., 2020; Thorpe et al., 2005).
Species within the genus Ceratocystis have several means of transmission and dispersal mechanisms, including by insects, wind, water, root grafts and pruning tools. They typically infect their host through entry wounds (Ferreira et al., 2010; Harrington et al., 2011; Holland et al., 2019). The symptoms caused by this pathogens include vascular wilt, exudation points, stem cankers and plant death (Al Adawi et al., 2014; Argôlo Magalhães et al., 2016; Harrington et al., 2011; Sanches et al., 2008). In Costa Rica, symptoms have been observed in melina plantations as early as 7 months of age (Arguedas et al., 2018).
High host specificity and geographic variation of the fungus has been demonstrated in several studies (Cordero Vega, 2017; Ferreira et al., 2011). Meneses Rojas (2008) suggesting that there maybe numerous undescribed cryptic species of the fungus, some of which could exhibit high specialization. Therefore, when conducting trials to identify Ceratocystis wilt resistant or tolerant plant material, it becomes necessary to work with isolates obtained from the specific host for accurate evaluation (Ferreira et al., 2011).
In the management of forest plantations, disease control has primarily relied on implementing good silvicultural practices, and preventive actions based on knowledge of risk factors (Desprez-Loustau et al., 2016). The use of synthetic fungicides has also been reported (ur Rehman et al., 2015). Currently, the effectiveness of disease protection in forest plantation depends on multidisciplinary collaboration and dynamic approaches such as good silvicultural management, use of biological control and resistant genotypes (Zhan et al., 2014; 2015).
With advancements in plant breeding programs, the selection of resistant or tolerant genotypes to pathogens has become an integral part of disease management strategies (Argôlo Magalhães et al., 2016; Sniezko & Koch, 2017). Therefore, the objective of this research was to develop and refine the protocol for inoculation of C. fimbriata and the evaluation of its effect on elite genotypes of melina, for using in the melina breeding program of the international forest breeding cooperative GENFORES.
Material and methods
Plant material
Melina (Gmelina arborea) elite genotypes 15N and 20, considered as highly susceptible materials to the disease caused by Ceratocystis fimbriata, were vegetatively propagated by cuttings from mini clonal gardens (Méndez-Álvarez et al., 2021). Additionally, genotypes 1, 57 and 58, known for their tolerance, according to previous field test evaluations were included (Méndez-Álvarez et al., 2021; Salas-Rodríguez et al. 2016). A total of 18 4-months-old plants (ramets) were produced from each clone in 6-liter pots with sterile peat as substrate to a height of approximately 60 cm at the facilities of International Forest Breeding Cooperative (GENFORES) on the campus of the Instituto Tecnológico de Costa Rica in Santa Clara, San Carlos, Costa Rica (10° 21' 15.78'' N and 84° 30' 58.88'' W). The location is situated at an altitude of 150 m.a.s.l., with an average annual temperature of 25.7 °C and an average annual precipitation of 3012 mm (Instituto Metereológico Nacional, s.f.), with no dry season. The trial was established between October 2019 and January 2020, and a final destructive evaluation of the trial was conducted.
Inoculum production
The inoculum was produced based on the isolation CIF 001 of C. fimbriata from melina trees in Costa Rica (Méndez-Álvarez et al., 2020), according to the following experimental treatment: 1) solid treatment, where the fungus was grown in Petri dishes with malt extract and yeast extract agar (MYEA) for 8 days at 25 °C. 2) liquid treatment, where one of the plates from treatment 1 was used, to which 15 ml of sterile distilled water was added and scraped with a sterile digralsky loop. The spore suspension was then filtered through sterile gauze, quantified in a Neubauer chamber, and diluted to a concentration of 2.5 x 106 spores/mL.
Pathogenicity test establishment
The test was conducted under completely enclosed greenhouse conditions with a zenithal opening. The greenhouse maintained an average temperature of 28 °C, an average relative humidity of 82.5 % and irrigation was provided once a day for 10 minutes. The trial followed a 3 x 5 factorial experimental design, which consisted of two inoculation treatments plus a control, with 5 elite genotypes and 6 plants per genotype as experimental unit. The genotypes were assigned in a block design and randomly distributed within the greenhouse. Treatments were randomly assigned within each block or genotype, with no buffer area between each block.
For treatment 1 inoculation process, an incision was made with a sterile 0.5 mm diameter punch 5 cm from the plant base. A same diameter disk of MYEA with C. fimbriata mycelium was placed in the previously open wound using a sterile mycological loop, and the wound was tightly sealed with an adhesive plastic wrap. Treatment 2 inoculation process involved opening a 2 cm herringbone-shaped longitudinal incision with a sterile scalpel, 5 cm from the plant base.
Subsequently, 500 µl of a pathogen spore suspension at 2.5 x 106 spores/mL concentration was inoculated using a micropipette. The wound was then sealed with an adhesive plastic wrap. In the control treatment, a superficial incision was made with a sterile scalpel, 5 cm from the base of the plant, and a disc of MYEA agar without the presence of the pathogen was placed. Finally, the wound was tightly sealed by wrapping with adhesive plastic.
Pathogenicity test evaluation
After inoculation, the behavior of the inoculum on the plants was evaluated at 30, 60, 90, and 120 days after the trail was established. The quantitative variables measured were total height (from base to apex) using a 1 m ruler, basal diameter using a no digital vernier, and the total number of functional leaves were counted.
Disease incidence was assessed as binary variable categorized as healthy (alive = 0) or diseased (dead = 1). Equation 1 proposed by Cavallini (1998) was employed to calculate the percentage of incidence for each genotype, and overall of the entire trial at each of the four evaluations.

The degree of resistance was determined by evaluating the internal lesion in each plant, based on the advancement of the fungus lesion from the initial inoculum incision. After 120 days the entire trial was harvested. Previous tool sterilization the control treatment was cut down first, followed by treatments 1 and 2. Each plant was labeled according to its genotype and treatment, the leaves and branches were removed, and the stems were transferred to the forest pathology laboratory in the Forestry Research and Innovation Center, School of Forestry Engineering, Technological Institute of Costa Rica.
In the laboratory, a longitudinal cut was made along the entire length/height of the plant by the use of a sterile tape (sterilized in each repetition). The length of the spot caused by C. fimbriata was measured from the point of injury in both directions (upward and downward), as described by Oliveira (2010). Severity was calculated using equation 2.

Degree of resistance was then determined according to the following scale:
resistant (≤10 %), tolerant (10 < X ≤ 20 %) and susceptible (>20%).
Statistical analyses
The collected data was first analyzed for compliance with homeostacicity assumptions. Subsequently, an analysis of variance (ANDEVA), was performed for each of the variables evaluated (total height, basal diameter, number of functional leaves, incidence, and degree of resistance) at 30, 60, 90 and 120 days. The data was analyzed using a Randomized Complete Block Design (equation 3).
Y = βGenotype + αTreatment + φGenotype xTreatment + e (3)
Where, β = the effect of i-th Genotype, α = the effect of j-th Treatment and φ = interaction effect of i-th Genotype by j-th Treatment (experimental unit).
A Duncan test (α=0.05) was performed to determine significant differences between treatments and genotypes. All statistical analyses were conducted using InfoStat software version 2019 (Di Rienzo et al., 2019).
Results
Pathogenicity evaluation
The results demonstrate the progressive expression of treatment effects on plant growth as infection advanced (Figure 1). At 30 days no visible treatment effects on plant growth were observed. At the evaluation at 60 days the first visible effect was observed, with reduction of height growth and a decrease in the number of leaves in plants inoculated with the fungus. By 90 and 120 days, the effects of both inoculation treatments were evident, resulting in lower height development, lower number of leaves and a slightly lower basal diameter. Number of leaves exhibited the most pronunced effect associated to the two inoculation treatments. A considerably lower number of leaves was observed in the plants at 90 and 120 days in comparison to the control treatment (Figure 1).
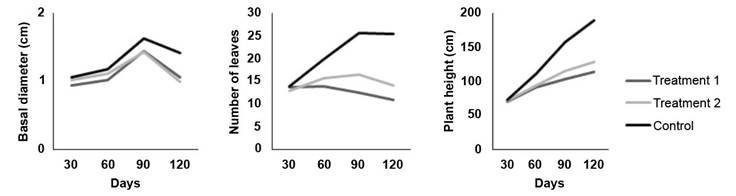
Figure 1 Effect of two inoculation treatments of C. fimbriata in G. arborea plants growth at 30, 60, 90, and 120 days. Treatment 1 (solid medium), Treatment 2 (liquid medium). Instituto Tecnológico de Costa Rica, Santa Clara, San Carlos, Costa Rica October 2019 to January 2020.
In general, trial plants reached an average height of 71.37 cm, a basal diameter of 1.01 cm and 13.51 leaves after 30 days of evaluation. After 60 days, the average height was 98.99 cm, 1.11 cm basal diameter and 16.53 leaves. At the 90-day evaluation the plants had an average height of 125.26 cm, a basal diameter of 1.50 cm and 18.25 leaves per plant. At 120 days the trees reached an average height of 140.25 cm, a basal diameter of 1.45 cm and 15.93 leaves per plant.
The results at 30 days indicate that, there were no significant differences (p>0.05) between inoculation treatments with the control, for any of the three response, total height, basal diameter and number of leaves. However, at the genotype level, significant differences (p<0.05) were observed between clones 20 and 15N in all the three variables. Addtionally significant differences (p<0.05) were found between clones 20 and 57 in terms of the number of leaves.
At the 60-days evaluation, significant differences (p<0.05) were observed between the inoculation treatments and the control plants which exhibited lower height, and lower number of leaves. However, there were no significant differences between treatments in the basal diameter. Regarding the genotypes, it was observed that clone 15N showed significantly lower growth compared to the other four genotypes.
Both the 90 day and 120 day evaluations showed significant differences (p<0.05) among treatments for the three response variables with respect to control. However, there were no significant differences (p>0.05) between the two infection treatments. Regarding the genotypes, significant differences (p<0.05) were observed at 90-days evaluation between genotypes 20 and 1 for plant height, 20 and 57 for basal diameter and, 15N and 57 for number of leaves. At 120-days evaluation significant, differences (p<0.05) were observed between genotypes 20 and 57 for height, 15N and 57 for basal diameter and, 15N and 1 for number of leaves.
Mortality was recorded 38 days after establishment. For the 60-day evaluation, there was a mortality rate of 14.4 % which increased to 21.1 % at 90 days, and finally reached 26,7 % at 120 days. Treatment 1 (solid medium) had the highest number of dead individuals in all three evaluations (Figure 2a). Genotype 15N had the highest number of dead individuals while genotype 1 had only one dead individual throughout the trial (Figure 2b).
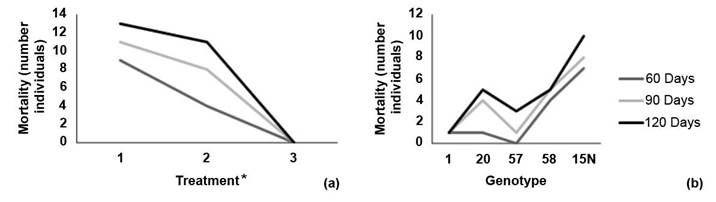
Figure 2 Reported mortality by number of individuals according to (a) inoculation or treatment method and (b) melina (Gmelina arborea) genotype at 60, 90 and 120 days of evaluation. Santa Clara, San Carlos, Costa Rica October 2019 to January 2020.
Test symptoms observed in greenhouse (Figure 3) were similar to those observed in field tests; including leaf wilt (Figure 3d) and the presence of basal sprouts (Figure 3c). This two symptoms appeared first, followed by weeping (Figure 3b), cankers (Figure 3a), and finally plant death (Figure 3e).
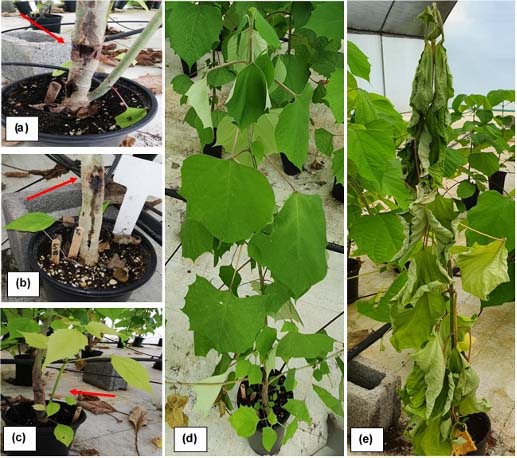
Figure 3 ''Ceratocystis wilt'' symptoms in Gmelina arborea inoculated clones at 90 days (a) Canker (b) Exudation point (c) Basal sprouts and near initial incision (d) Wilting (e) Death and wilting. Santa Clara, San Carlos, Costa Rica October 2019 to January 2020.
After 60 days there were significant differences in disease incidence between the two inoculation treatments. Inoculation with solid medium showed a significantly higher number of diseased plants (Figure 4b). Among genotypes, clone 57 did not register a single diseased plant, while clone 15N showed all diseased plants (Figure 4a).
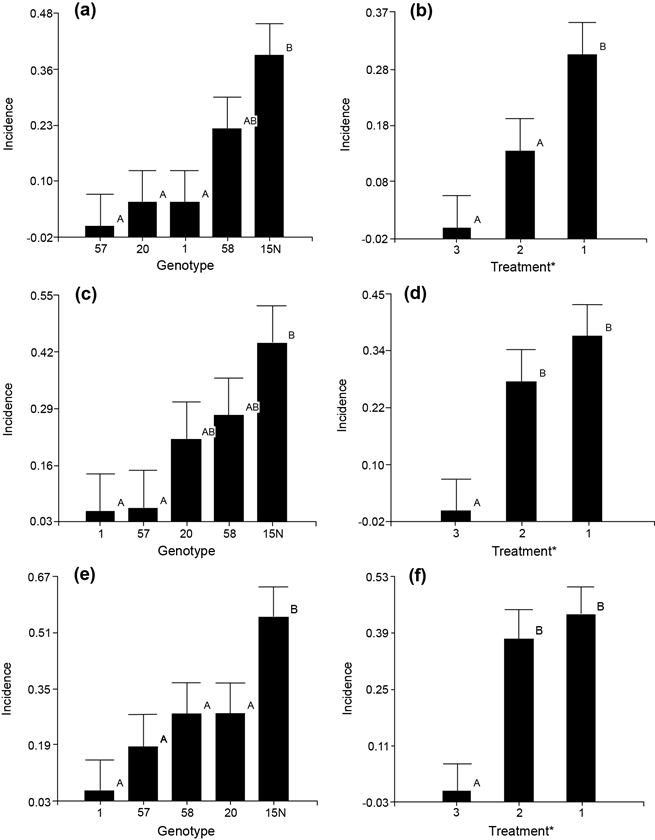
Figure 4 Disease incidence evaluation in the inoculation trial of Ceratocystis fimbriata in Gmelina arborea genotypes. (a) 60-days evaluation according to the genotypes (b) After 60 days according to inoculation method (c) 90-days evaluation according to the genotypes (d) After 90 days according to inoculation method (e) 120-days evaluation according to the genotypes (f) After 120 days according to inoculation method. Santa Clara, San Carlos, Costa Rica October 2019 to January 2020.
After 90 days, the pattern of pathogen incidence became more evident. Although inoculation method 1 consistently exhibited significant differences from 60-day evaluation. Among the genotypes the pattern was almost established after 90 days, but more consistent after 120 days. Clone 20 was always positioned at the middle and showing a moderate tolerance behavior with an incidence rate of 25-28 % throughout the test evaluation.
In the 120-day evaluation, there were no significant differences between treatments (1 and 2), but there were clear differences compared to the control (treatment 3, Figure 4f). At the genotype level the same two-groups pattern persisted with very tolerant and highly susceptible. Clone 15N exhibited the highest susceptibility to Ceratocystis with all its plants showing disease symptoms. On the other hand, clone 1 was the only resistant which showed healthy all its plants. Genotypes 57, 20 and 58 showed a moderate tolerance pattern, although there were not significant differences among them (Figure 4e).
In the final destructive evaluation, no significative differences in the progress of the internal lesion were observed, between the two inoculation methods (Figure 5b). However, significant differences were evident between genotypes 1 and 15N (Figure 5a). Based on this criterion, all genotypes were classified as highly tolerant or resistant (genotype 1), moderately tolerant (genotypes 20 and 57) and highly susceptible (genotypes 58 and 15N, Figure 5a).
Discussion
In pathogenicity trials it is not usually evaluated the effect in growth traits like height and basal diameter. However, in this investigation significant effects of the fungus C. fimbriata on plant development were determinated, compared to the control plants. These growth effects became more pronounced from 60 to 90 days, and further to 120 days, as pathogen advancement within the vascular system of inoculated plants (Figure 1).
A related pattern can be observed between diameter size and disease development caused by C. fimbriata (Murillo-Gamboa et al., 2016). However, this pattern was not observed in the trial, possibly because the tests were performed on very young juvenile plants (less than 1 year old) and in plastic masseters with its limited growth conditions. The symptomatology observed in the pathogenicity trial in greenhouse, was similar to that reported in field work (Arguedas et al., 2018; Murillo-Gamboa et al., 2016; Salas-Rordríguez et al., 2016), such as the presence of canker, weeping, wilting, resprouting and finally death (Figure 2).
At 90 days, incidence reached 21.1 %, a value similar to that reported in commercial plantations with 2.7-year-old clonal material in southern Costa Rica. Where an incidence of 22.6 % was recorded (Murillo-Gamboa et al., 2016), these results support the veracity of these pathogenicity tests. The pathogenicity of the C. fimbriata strain used in some trials was determinant in the time elapsed until the appearance of visible symptoms, from inoculation to mortality (Harrington et al., 2011). In this study, mortality initiation was observed at day 38th, similar to that reported in young plants of Hevea brasiliensis inoculated with strains of C. fimbriata, isolated in Bahia, Brazil, where plants lasted less than 60 days to die (Valdetaro et al., 2015).
It is known that the time elapsed from inoculation to the first symptoms appearance or death, varies considerably among hosts and strains used (Cordero Vega, 2017; Valdetaro et al., 2015). It was observed how none of the single plants within each genotype, presented symptoms at the same time. Except for genotype 15N, where most of its plants (seven ramets) had died at the 60th-day evaluation (Figure 4). This variation in symptoms initiation can be attributed to different factors such as, strain pathogenicity, characteristics of the inoculation method (spore concentration, wound position) and the initial plant conditions like age, vigor, substrate, fertilization, and water regime (Argôlo Magalhães et al., 2016; Sanches et al., 2008).
With respect to strain pathogenicity, C. fimbriata showed little morphological variation among isolates. However, differences have been found within the same species, which may explain its variation and specificity in isolated strains from hosts (Cordero Vega, 2017; Valdetaro et al., 2015). Testing only one single strain does not allow to determine its degree of pathogenicity. Likewise, the inoculation method was homogenized by performing a single wound in the same position in the host plant. However, the type of wound needed to be modified according to the inoculum used (solid and liquid), where it was respectively performed through a circular and herringbone shape.
Differences were found between the two treatments (solid treatment or number 1; and liquid treatment or number 2) at 60 days (Figure 4b). The concentration of the liquid treatment was similar to that reported in pathogenicity trials on cocoa, sweet potato, coffee, mango and Metrosideros polymorpha plants (Al Adawi et al., 2014; Argôlo Magalhães et al., 2016; Barnes et al., 2018; Harrington et al., 2011). These studies reported similar mortality rates to the findings in this research with melina. However, no significant differences were determined at 90 and 120 days between both treatments (Figure 4d and 4f).
Although the experimental material used in the trails was relatively homogeneous, there were still small initial differences in their size and basal diameter. Which may explain that some genotypes showed a significant greater and vigorous growth at 60 and 90 days. Plants were assessed as intolerant by showing any of the wilting symptoms, like basal sprouts, liquid spillage from stem, leaf loss at the end of branch, among others. The degree of tolerance could be also assessed by counting the number of infected ramets per clone.
Even though the greenhouse guarantees good environmental control, some degree of variation may persist that could partially affect experimental results. Therefore, it is recommended to observe the progress pattern of internal lesions caused by the fungus, up and down direction from the wound along the stem. In their investigations reported found differences in the advance of internal necrosis in tissues caused by C. cacaofunesta, as well as in the degree of plant growth (Alvarenga Arriel et al., 2016; Sanches et al., 2008).
In this melina trial similar results were observed, where the evaluation of internal lesion allowed the determination of genotypes as intolerant, despite its lack of external symptoms (Figure 5a). Other research reported using length of internal lesion caused Ceratocystis spp. as a percentage of disease severity (Alvarenga Arriel et al., 2016; Oliveira et al., 2015) in species such as mango, eucalyptus, ficus among others. Thus, identifying genotypes susceptible or resistant to the disease, which can then be utilized in breeding programs for each species (Guimarães et al., 2021; Yakushiji et al., 2019).
This type of results can be associated with the genotype-pathogen response, such as the production of antifungal compounds (Harrington et al. 2011; Valdetaro et al., 2015). Due to the high incidence of C. fimbriata in melina plantations in the country, which promotes a high degree of mortality with an important economic impact, its prevention through the usage of tolerant genotypes, looks like to be one of the best approaches. Therefore, it is essential to focus on the search for genetically resistant or tolerant materials, as one of the best options for integrated disease management (Alvarenga Arriel et al., 2016; Argôlo Magalhães et al., 2016; Yakushiji et al., 2019).
In the search for greater efficiency in the fight against this disease, the degree of virulence of the strain should be considered (Valderato et al., 2015). The most aggressive Ceratocystis strain need to be determined, to use it for screening purposes of the collection of genotypes in the breeding population.
Conclusions
Treatment 1 using the solid medium was found to be the most effective method of inoculating C. fimbriata in melina plants. The evaluation of disease incidence and severity in the collection of genotypes, showed the need to assess results after at least 90 days of inoculation. Among the five clones investigated, genotype 15N can be distinguished as highly susceptible, while genotype 1 as highly tolerant to C. fimbriata (isolate CIF 001). There was no evidence of completely resistant genotypes, but highly tolerants will be included in melina breeding programs of GENFORES.