Introduction
Coral reefs are characterized by high biodiversity, hosting 32 % of all known marine species and provide essential ecosystem services that contribute to the well-being of millions of people (Fisher et al., 2015; Knowlton et al., 2010; Woodhead et al., 2019). They are considered endangered ecosystems (Carpenter et al., 2008) and, most importantly, one of the most sensitive ecosystems to climate change (Riegl et al., 2009). Since 1970, coral cover in the Caribbean has decreased by 80 %, mainly due to the high mortality of reef-building corals, which has led to a phase-shift from coral to algae, and a loss of ecological functionality, as the result of both natural and anthropogenic impacts (Álvarez-Filip et al., 2022; Arias-González et al., 2017; Aronson & Precht, 2001; Jackson et al., 2014; Rioja-Nieto & Álvarez-Filip, 2019). This has brought about the loss of recruitment for the main reef-building corals (Edmunds & Elahi, 2007; Edmunds et al., 2011; Hernández-Delgado et al., 2014; Quinn & Kojis, 2005; Williams et al., 2008), which include the species Orbicella annularis (Ellis & Solander, 1786) and Orbicella faveolata (Ellis & Solander, 1786).
Larval settlement as well as survival and growth of coral species, are integral to the ongoing maintenance of coral populations and necessary for the recovery of coral reefs (Ritson-Williams et al., 2009). Many factors can impact these processes, such as the abundance of fleshy algae, competition with other benthic invertebrates, predation, and sedimentation (Pizarro et al., 2007). To assist in the recruitment of corals, restoration strategies have become more critical over the past decades and have involved culturing coral settlers from gametes to improve the genetic diversity of coral population (Baums et al., 2019). However, one of the significant bottlenecks in this process is post-settlement survival and coral settler growth (Ritson-Williams et al., 2009), mainly due to competition with fleshy algae and predation (Edwards & Gomez, 2007), but also include important abiotic factors such as water clarity, sedimentation, nutrient enrichment, salinity, and temperature (Richmond, 1997).
In controlled conditions (Ex-situ) settlement may be enhanced by using substrates with surfaces containing specific larvae-attracting attributes, including varying colour phosphorescence properties. Phosphorescence is a specific type of photoluminescence whereby, once the light has been absorbed, it is emitted and continues to be emitted even after excitation has ceased (Valeur & Berberan-Santos, 2011). It is a property that can generate micro-environments of coloured light in otherwise dark conditions, sometimes preferred by certain species as a condition for settlement (Mason et al., 2011; Strader et al., 2015).
Post-settlement survival may also be enhanced by using substrates with surface properties that may promote a reduction in competition, nutrient supply, and coral skeleton growth. This can be achieved by engineering substrate coatings with different chemical properties and hydrophobicity (Levenstein et al., 2022). Hydrophobicity can play an important role in reducing competition with algae from the genus Ulva, as it can affect algal spore adhesion and the strength of the adhesion (Chaudhury et al., 2006; Finlay, 2002; Fletcher & Callow, 1992). On the other hand, the presence of hydroxyapatite on the substrate's surface can be a source of phosphate released as the hydroxyapatite potentially dissolves in low phosphate ion concentration scenarios in seawater (Atlas & Pytkowicz, 1977; Stumm & Morgan, 1996). This nutrient may be taken up by symbiotic dinoflagellates and converted into essential organic molecules (Ezzat et al., 2016). Similarly, the presence of calcium carbonate on a substrate's surface provides a source of carbonate and calcium ions, the building blocks of aragonite-based coral skeletons, which can be rapidly released upon CaCO3(s) dissolution in response to local variations in seawater chemistry, affecting CaCO3(s) saturation state, such as acidity build up in the vicinity of the coral tissue-seawater interface (Venn et al., 2013).
The research presented here aims to investigate the synergistic effects of specific physical-chemical properties (hydrophobicity, phosphorescence and mineral-enriched surface chemistry) on settlement as well as post-settlement survival for O. annularis, and O. faveolata from Puerto Morelos, Mexico.
Materials and methods
Substrate fabrication: In 2019, coatings were applied to commercially available floor tiles to test a combination of properties and their potential effect on settlement and post-settlement survival of coral larvae. Each coating was designed to provide a combination of two out of three properties: 1) water repellence (as determined by its degree of hydrophobicity), 2) phosphorescence-based colour, and 3) mineral-enriched surface chemistry. The ceramic floor tiles (Interceramic©, Mexico 5 cm × 5 cm × 5 mm) were used as the substrate to which six sets of coatings (C1 to C6) were applied (Table 1). The tile surfaces were roughened using water-based sandpaper to improve adhesion before applying the coating. A hydrophobic polyester resin coating served as a base for the application of micro or nanoparticles and was applied to all tiles except C6. Tiles C1-C3 contained microparticles of strontium aluminate salts with varying relative concentrations of rare earth elements europium and trivalent dysprosium (SrxEuyDyzAl2O4), each yielding specific colour phosphorescent properties (Distribuidora Química Textil S.A. de C.V., Mexico): light blue (C1), red-orange (C2) and turquoise (C3). Phosphorescent microparticles in tiles C2 also contained a coumarin-type red-emitting fluorescent pigment. On tiles C4, 60 nm hydroxyapatite nanoparticles (Ca10(PO4)6(OH)2, 96 % purity) (MK Impex Corp., Canada, MKN-HXAP-060) were applied, whereas 80 nm nanoparticles of amorphous calcium carbonate (CaCO3, > 98 % purity) (MK Impex Corp., Canada, MKN-CaCO3-080) were applied to tiles C5 and C6. A commercially available superhydrophobic polysiloxane-based coating (Eronde, USA, 9HMR-FIX) was applied on the dry surface of the polyester resin of tiles C1, C2, and C3. In the case of C6, the superhydrophobic coating was applied directly onto the tile surface and served as a base for applying the CaCO3 nanoparticles.
Table 1 Properties and characteristics of substrate coatings.
Substrate ID | White paint | Hydrophobic coatings | Characteristics of micro or nanoparticles | ||
Intermediate hydrophobicity polyester resin | Superhydrophobic polysiloxane coating | Size particles | Properties | ||
C1 | No | Yes | Yes | Micro | Light blue phosphorescent |
C2 | No | Yes | Yes | Micro | Red-orange phosphorescent |
C3 | No | Yes | Yes | Micro | Turquoise phosphorescent |
C4 | No | Yes | No | Nano | Hydroxyapatite |
C5 | No | Yes | No | Nano | Amorphous calcium carbonate |
C6 | No | No | Yes | Nano | Amorphous calcium carbonate |
T1 | Yes | Yes | Yes | No | No |
T2 | Yes | Yes | No | Micro | Light blue phosphorescent |
T3 | Yes | Yes | No | Micro | Red-orange phosphorescent |
T4 | Yes | Yes | No | No | No |
T5 | Yes | No | No | No | No |
T6 | No | No | No | No | No |
In 2021, we evaluated the individual properties of coatings on settlement preference of Orbicella spp. larvae to determine if it is determined by a combination of properties or by an individual property. We focused on substrates C1 and C2 because they elicited greater settlement compared to the other tiles that were tested. The same brand of ceramic floor tiles from the previous experiment was used. We prepared six treatments (T1 to T6, Table 1). All the treatments had a hydrophobic polyester resin coating. For treatment T1 a superhydrophobic polysiloxane-based coating (same as the previous experiment) was applied. Treatments T2 and T3 contained microparticles of strontium aluminate salts with light blue and red-orange colours, respectively. Treatment T4 had a hydrophobic polyester resin coating applied. White paint was added to T5 and T6 consisted of the bare ceramic floor tile without coatings.
Substrate conditioning: Ten replicates of each treatment (T1 to T6) were placed in flow-through seawater aquaria (480 L) for seven weeks to pre-condition them and allow biofilm formation (Sneed et al., 2014). Sea water was filtered to 1 µm and set to the following conditions: temperature 27-28.5 °C, salinity 35-36 psu, pH 7.9-8.3. Concentrations of ammonia, nitrite, and nitrate were undetectable using standard methods (Grasshoff et al., 1999). Therefore, the remaining ten substrates of each treatment were maintained without conditioning. Substrate conditioning was evaluated for Orbicella spp. in 2021 (Table 2). It was not tested in 2019, because we did not have enough replicates.
Collection of gametes, assisted fertilization, and care of embryos: One week after the full moon in September 2019, gamete bundles were collected from four colonies of Orbicella annularis from Jardines Reef (20°49'53.0” N, 86°52'28.2” W), as well as three colonies of O. faveolata from La Bocana Reef (20°52'25.7” N, 86°51'04.2” W). In September 2021 gamete bundles were collected from 10 colonies of O. annularis and two colonies of O. faveolata from Jardines Reef. The gametes were collected using nets that concentrated the gamete bundles into small plastic containers at the top of the nets. These reefs are located in the Puerto Morelos Reef National Park, Mexican Caribbean. Afterwards, all the gametes were transported to the laboratory, where gamete predators (crustaceans, polychaetes, and fish larvae) were removed using transfer pipettes, and gametes from the different colonies of the same species were mixed in a single 6 L container to assist fertilization. Subsequently, excess sperm was removed, and the developing embryos were transferred to a culture system, where they developed into planula larvae.
Table 2 Experimental design of coatings with combinations of properties (2019) and coatings with a single property (2021).
Species, year | Containers | Number of substrates | Larvae per ml | Variables | |||
Coatings | Conditioning | ||||||
Treatments | Replicates per treatment | Treatments | Replicates per treatment | ||||
O. annularis - O. faveolata 2019 | 5 (6 L) | 12 substrates per container, 2 per coating | 0.2 | C1 to C6 | 10 | ||
O. annularis - O. faveolata, 2021 | 10 (2 L) | 6 substrates per container, 1 per coating | 0.1 | T1 to T6 | 10 | C, UNC | 30 |
Abbreviations: Conditioned (C), and Unconditioned (UNC).
Choice experiment: Three days after spawning, 6 000 O. annularis larvae were distributed evenly into five replicate sets of 6 L plastic containers (1 200 larvae per container). Each plastic container had two substrates of each type of treatment (C1 to C6). The same experimental design was repeated for O. faveolata (Table 2). The settlement was recorded on days eight, 13, and 18 after spawning. One day after the last count of the substrates, those with O. annularis and O. faveolata primary polyps were transferred to flow-through aquaria with access to natural light, food, and symbionts. Substrates were scored for survival 13, 20, 27, 34, and 41 days after settlement.
Three days after spawning, 2 000 O. annularis larvae were distributed evenly into ten replicate sets of 2 L plastic containers (200 larvae per container). Each container had six substrate coatings (T1 to T6). Five containers had conditioned substrates, and five containers had unconditioned substrates (Table 2). On days eight, 13, and 18 after spawning, O. annularis settlement was recorded. One day after the last count, substrates with O. annularis primary polyps were transferred to flow-through aquaria. The substrates were scored for survival seven, 14, 21, 28, 35, and 42 days after settlement. The same experimental setup and methodology were used for O. faveolata.
Statistical analysis: A generalized linear model (GLM) with a negative binomial distribution and a logit link was used to analyse settlement in the O. annularis and O. faveolata choice experiment between substrate coatings with combinations of properties (C1 to C6, 6 levels) and in the O. annularis and O. faveolata choice experiment to determine the effect of substrate coatings with a single property (T1 to T6, six levels) and substrate conditioning (Conditioned and unconditioned, two levels). The models are selected by the Bayesian Information Criterion (BIC). Model validations revealed that there was no overdispersion. Likelihood ratio chi-square (LR Chisq) was performed for statistical inference. Tukey tests for post hoc analysis were then used. Kaplan-Meier survival analysis was performed to determine differences in survivorship between substrate coatings (C1 to C6 and T1 to T6, six levels) in the O. annularis and O. faveolata choice experiments. Survival between the substrate coatings (C1 to C6 and T1 to T6, six levels) was compared using log-rank tests. All statistical tests were carried out using R (R Core Team, 2019), and the results were plotted using the ggplot2 package (Wickham, 2009). Data are presented as mean ± standard error (SE).
Results
Coatings with combinations of properties
For Orbicella annularis a maximum of 258 settlements was recorded on the coatings, representing 4.30 % of the 6 000 larvae placed in the containers. A maximum of 171 O. faveolata settlers was recorded on the substrate coatings, representing 2.85 % of the 6 000 larvae used in the experiment.
Choice experiment: Despite the low settlement, distinguishable differences were found in the number of O. annularis settled larvae (Fig. 1) (LR Chisq = 25.74, P = 0.0001), with a greater number of settlers found on substrate C1 when compared to substrate C4 (Tukey, P = 0.0015), and C6 (Tukey, P = 0.02). The number of settlers was significantly greater on substrate C2 when compared to substrate C4 (Tukey, P = 0.007). A total of 196 (3.3 ± 0.43) settlers were observed on the coatings of the substrates on day eight after spawning, 133 (2.0 ± 0.25) on day 13, and 58 (0.9 ± 0.18) on day 18. This shows that the highest number of settlements for this experiment occurred between day three and day 12 after spawning under these conditions. Similarly, for O. faveolata significant differences were found in the number of settled larvae (Fig. 1) (LR Chisq = 12.27, P = 0.03), with lower settlement on substrate C4 when compared to substrate C2 (Tukey, P = 0.04). A total of 27 (0.4 ± 0.10) settlers were observed on the upper surface of substrates on day eight after spawning, 139 (2.2 ± 0.27) on day 13, and 109 (1.8 ± 0.27) on day 18. This shows that the highest number of settlements for this experiment occurred between days nine and 13 after spawning under these conditions.
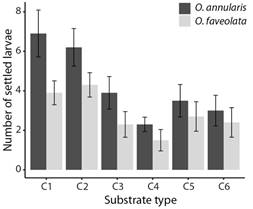
Fig. 1 The maximum number of O. annularis, and O. faveolata larvae settled on substrates with coatings with combinations of properties. Values are means (N = 10), and error bars represent ± standard error.
Post-settlement survival: The highest number of polyps settled was on substrates C1 and C2 compared to other substrates. The Kaplan-Meier survival curves demonstrated a mean survival time of 14.00 ± 0.68 days after settlement on substrate C1, 13.37 ± 0.37 days on substrate C2, 13.00 ± 0.00 days on substrate C3, 16.89 ± 1.23 days on substrate C5 and 18.6 ± 4.08 days on substrate C6 (Fig. 2A). The survival time was significantly lower in substrate C2 than substrate C5 (Log-rank test, P = 0.02).
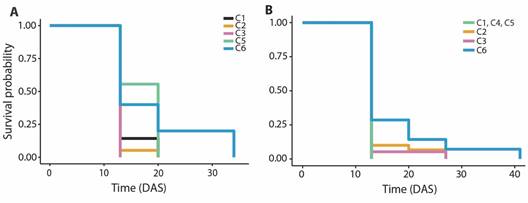
Fig. 2 Survival probability of primary polyps on substrates with coatings with combinations of properties (C1 to C6) for 41 days after settlement (DAS). A. O. annularis. B. O. faveolata.
A total of 109 O. faveolata primary polyps were transferred to aquaria after the last count, distributed between the substrate types, mostly on C1 and C2 substrates. The Kaplan-Meier survival curves revealed a mean survival time of 13.00 ± 0.00 days after settlement on substrate C1, C4, and C5, 14.17 ± 0.68 days on substrate C2, 13.74 ± 0.74 days on substrate C3 and 17.00 ± 2.17 days on substrate C6 (Fig. 2B). No significant differences were found in the survival time between substrate coatings (Log-rank test, P = 0.08).
Coatings with a single property
For O. annularis, a maximum of 64 settlers was recorded on the substrate coatings, representing 3.20 % of the 2 000 larvae originally placed in all containers. A maximum of 33 O. faveolata settlers was recorded on the substrate coatings, representing 1.65 % of the 2 000 larvae placed in the containers.
Choice experiment: Although the settlement was low, differences were found in the number of O. annularis settled larvae between the coatings (Fig. 3) (LR Chisq = 18.96, P = 0.002), with a significantly greater number of settlers found on substrate T6 when compared to substrate T2 (Tukey, P = 0.03), and T4 (Tukey, P = 0.046). No significant difference was found between substrate conditioning and lack of conditioning (Fig. 4) (LR Chisq = 0.63, P = 0.43). A total of 16 (0.27 ± 0.09) settlers were observed on the coatings of the substrates on day eight after spawning, 38 (0.63 ± 0.15) on day 13, and 11 (0.18 ± 0.07) on day 18. This shows that the highest number of settlers for this experiment occurred between day nine and day 13 after spawning under these conditions.
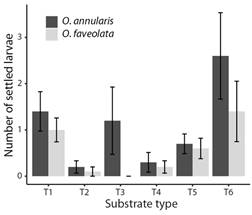
Fig. 3 The maximum number of O. annularis and O. faveolata larvae settled on substrates with coatings with a single property. Values are means (N = 10), and error bars represent standard error.
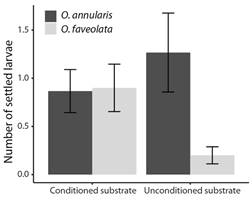
Fig. 4 The maximum number of O. annularis, and O. faveolata larvae settled on conditioned and unconditioned substrates. Coatings with a single property (T1 to T6). Values are means (N = 30), and error bars represent standard error.
For O. faveolata no significant difference was found in the number of settled larvae between the substrate coatings (Fig. 3) (LR Chisq = 1.90, P > 0.05). However, differences were found between substrate conditioning (Fig. 4) (LR Chisq = 13.92, P = 0.0002), with a significantly greater number of settlers found on conditioned substrates compared to unconditioned substrates. A total of 17 (0.28 ± 0.11) settlers were observed on the coatings of the substrates on day eight after spawning, 21 (0.35 ± 0.11) on day 13, and 9 (0.15 ± 0.07) on day 18. This shows that the highest number of settlers for this experiment occurred between days nine and 13 after spawning under these conditions.
Post-settlement survival: A total of 11 O. annularis primary polyps were transferred to aquaria after the last count, distributed between T1, T3, T5, and T6 substrate types, with the highest number of polyps settled on substrates T1. The Kaplan-Meier survival curves demonstrated a mean survival time of 7.00 ± 0.00 days after settlement on substrate T1 and T3, 28.00 ± 0.00 days on substrate T5 and 17.50 ± 3.50 days on substrate T6 (Fig. 5A). No significant differences were found in the survival time between substrate coatings (Log-rank test, P > 0.05).
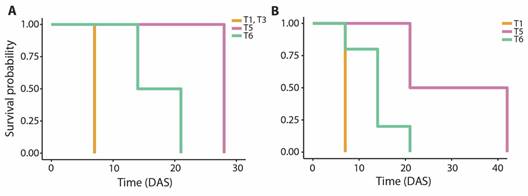
Fig. 5 Survival probability of primary polyps on substrates with coatings with a single property (T1 to T6) throughout 42 days after settlement (DAS). A. O. annularis. B. O. faveolata.
Nine O. faveolata primary polyps were transferred to aquaria after the last count, distributed between T1, T5, and T6 substrate types, mostly on T6 substrates. The Kaplan-Meier survival curves revealed a mean survival time of 7.00 ± 0.00 days after settlement on substrate T1, 31.50 ± 10.5 days on substrate T5, and 14.00 ± 2.21 days on substrate T6 (Fig. 5B). No significant differences were found in the survival time between substrate coatings (Log-rank test, P > 0.05).
Discussion
Overall, our results show that the presentation of substrates with distinct chemical coatings and combinations to two species of coral larvae results in low but distinct settlement patterns and post-settlement survival.
For O. annularis, the substrate with the combination of properties with a high level of hydrophobicity combined with light blue phosphorescent micro-particles (C1) and the substrate with a high level of hydrophobicity and red-orange phosphorescent micro-particles (C2) were the preferred settlement substrates when compared to the other combinations. Nevertheless, the experiment with a single property revealed that the ceramic control (T6) was preferred over intermediate hydrophobicity (T4) and light blue phosphorescent micro-particles (T2). For O. faveolata, we found that a high level of hydrophobicity, combined with the red-orange (C2) phosphorescent micro-particles, was the preferred combination as a settlement substrate when compared to the substrate with intermediate hydrophobicity and hydroxyapatite coating (C4). This means that combinations of properties generate successful settlement on these substrates. Individual properties did not generate this effect. The fact that the greatest number of settlers was observed on substrates with phosphorescent microparticles, and a high level of hydrophobicity confirms that these species use photosensitivity and respond to colour, in this case, light blue and red-orange, to fine-tune the selection of appropriate substrates or microhabitats (Mason et al., 2011). This photosensitivity towards certain wavelengths of light may assist larvae in selecting a microhabitat (Strader et al., 2015), serving as a form of depth gauge (Mason & Cohen, 2012) or guide, given that the distribution of light on coral reefs is highly heterogeneous at the microhabitat level due to depth, water clarity, bottom slope, substrate type and exposure (Brakel, 1979).
The substrate types determined the survival rates of O. annularis and O. faveolata recruits. On transferring the recruits to aerated aquaria, the number of polyps that survived on the substrates dropped abruptly, especially for those coated with polyester resin. This was evidenced by the fact that in the experiment with coatings with combinations of properties, the longest survival time (41 days after settlement) was associated with the only substrate without a polyester resin coating (C6). In the experiment with coatings with a single property, we observed the same pattern; the longest survival time (42 days after settlement) was on a substrate without this polyester resin coating (T5). The polyester resin used is somewhat soluble in water; hence the resin may have slowly dissolved, resulting in an unstable substrate, possibly preventing viable adhesion and compromising long-term post-settlement survival. Additionally, hydrophobic surfaces generate a contact angle with the water greater than 90°. When this contact angle is greater, the adhesion force to this surface will be less (Lejars et al., 2012). Therefore, hydrophobicity generates a weaker adhesion force between the recruit and the substrate and possibly, when generating movement in the aquarium system or due to aeration, the settlers detached from the substrate possibly due to a weak attachment. Therefore, using these hydrophobic surfaces to prevent the colonization of other organisms, such as filamentous algae, can also be detrimental to coral survival since they function as antifouling coatings that allow for easy release when water motion is applied (Lejars et al., 2012). This would mean that, even though the combination of high hydrophobicity and phosphorescent micro-particles presents the most favourable settlement option compared to other combinations, the component used to create intermediate hydrophobicity (polyester resin) and high hydrophobicity may have been detrimental to settler attachment.
However, even though substrate conditioning is known to encourage settlement (Erwin et al., 2008), in the experiment with coatings with combinations of properties, it should also be noted that none of the substrates used in the O. annularis and O. faveolata experiments were conditioned; hence no biofilm could have formed at their surfaces, yet larvae settled successfully, which means that conditioning is unnecessary for these substrates. This represents an advantage over other substrates (Harrison & Wallace, 1990; Patterson et al., 2016; Petersen et al., 2005) that require conditioning prior to larval settlement. However, for the experiment with coatings with a single property, we found that the settlement of O. faveolata was greater on conditioned substrates; this may indicate that these substrates with a single property are not attractive enough on their own to larvae and require conditioning to generate an adequate settlement cue for coral larvae.
Orbicella annularis and O. faveolata larvae showed different behaviours, most notably in their settlement patterns. For O. faveolata, most larvae settle within the first two weeks if an appropriate site is found, with 80 % of larvae remaining in the plankton for more than eight days before attempting to settle (Szmant & Meadows, 2006). Our results show that the peak settlement period for O. faveolata larvae was between nine and 13 days after spawning. For O. annularis, the settlement peak was between three and eight days after spawning. This behaviour may also affect the affinity that O. annularis larvae have for the substrates with the combination of properties. More O. annularis larvae settled and they settled more rapidly when compared to O. faveolata larvae. Furthermore, we did not find this pattern in O. annularis in the single-property experiment, possibly because the larvae were not attracted to the substrates. Thus, they took more time to settle and were therefore settled in a time frame similar to O. faveolata larvae.
In conclusion, this is the first study to report the ability of O. annularis and O. faveolata to detect phosphorescence and hydrophobicity during the substrate selection process, which could be key to micro-habitat selection. Furthermore, this is the first time that the effects of substrate surface properties have been tested on these species, with varying effects. On the one hand, microparticles with blue and red-orange phosphorescence and high hydrophobicity favour settlement in O. annularis and O. faveolata, but some of the components used to coat the substrates may be detrimental to the survival of the polyps over time. These issues need to be addressed as long-term survival is critical in restoration projects involving the outplanting of coral settlers. Finally, it is essential to keep testing different combinations of substrate surface properties to determine the ones that are most conducive to larval settlement and polyp survival as well as being the least favourable to fleshy algal settlement. This will help us to identify the most suitable substrates for each species and is vital for optimizing future restoration efforts.
Ethical statement: the authors declare that they all agree with this publication and made significant contributions; that there is no conflict of interest of any kind; and that we followed all pertinent ethical and legal procedures and requirements. All financial sources are fully and clearly stated in the acknowledgements section. A signed document has been filed in the journal archives.