Introduction
Among the aquatic ecosystems, the tropical rivers of the Andes stand out for the diversity and heterogeneity of the communities they contain (Cavelier et al., 2008). In addition, they are characterized by representing an abundant water supply and high erosion processes and transport of sediments, due to climatic conditions as well as the geomorphology and topography of the region (Gil-Gómez, 2014). In Colombia, this abundance of water has favored its use for hydroelectric generation, particularly in eastern Antioquia, where a significant number of energy projects are concentrated. Consequently, numerous rivers located in this region of the country have been dammed.
One of the main effects of regulation, beyond changes in water quality, is the alteration of the flow regime and habitat for aquatic communities (Ponsati, et al., 2015; Roldán-Perez, 2016). Particularly physical, chemical, and morphological alterations in the channels, both on the spatial and temporal scale (Gil-Gómez, 2014; Roldán- Pérez & Ramírez, 2022) can directly affect the stability of the structure of benthic communities such as phycoperiphyton and macroinvertebrates (Carlisle, et al., 2011; García et al., 2017). For example, flow regulation can cause modifications in the characteristics of the substrata and/or the disposition of allochthonous organic matter, altering the river's capacity to process organic matter and causing changes in trophic functional groups, including an increase in filter-feeding organisms that are filter-collectors on the shredders (Cabrera et al., 2021; Tank, et al., 2010; Vimos-Lojano, et al., 2020). Or it can also induce a proliferation of algae that in turn alters the composition of the macroinvertebrate community, favoring the dominance of species that consume these resources (e.g., mayflies, Tricoptera and Plecoptera-ETP) (Tonkin et al., 2009). The ENSO/El Niño and La Niña phenomena have also been documented for their significant effects on the rainfall and drought regime. Consequently, there are effects on variations in the flow, temperature and water chemistry (turbidity, nitrogen, dissolved oxygen), as well as effects on the characteristics of the habitat that include the availability of substrates and the speed of the flow, causing alterations in the taxonomic and functional composition and, in general, in the structure of the macroinvertebrate and phycoperifiton communities (Ríos-Pulgarín et al., 2016). For this reason, these phenomena should be considered in studies on benthic communities in regulated systems, both to assess possible synergistic effects with regulation, and to avoid misinterpretations of the results.
This is an important and necessary knowledge, since these communities have a fundamental role in the contribution and transfer of energy, either as intermediate or final links with respect to other trophic levels (Mora-Day et al., 2009), given that they consume the organic matter produced by photosynthetic organisms growing as riverside vegetation that is transferred to their predators (Fernández, 2012).
At the base of these trophic chains is the phycoperiphyton, made up of different divisions of algae developed on a substrate that fix inorganic carbon and capture the sun´s energy, converting it into organic compounds (Moreno-Rodríguez, et al., 2017). The next link are the macroinvertebrates, made up mostly of larval arthropods, mollusks, and annelids that are especially abundant in tropical Andean rivers and that have an important role in the grazing of the phycoperiphytic community (Holomuzki et al., 2010).
Numerous authors have studied the relationship of environmental parameters with the structure of aquatic benthic communities (Gordillo-Guerra et al., 2014; Huertas-Farias et al., 2019; Oscoz et al., 2007), but only a few have considered the trophic connections between the communities (Carrasco-Badajoz, et al., 2022; Moreno-Rodríguez et al., 2017; Silva-Poma & Huamantinco-Araujo, 2020;). These connections are important since phycoperiphyton is a source of energy for macroinvertebrates and can exercise bottom-up control (from producers to consumers) in the aquatic ecosystem. Grazing can exercise significant control over algal density and biomass, but different responses are expected, depending on the characteristics of the producer and the herbivore, since the morphology and chemistry of a producer can affect its consumption risk (Holomuzki et al., 2010). This aspect has not yet been explored in regulated tropical Andean rivers, where the effect of seasonality may be less than that of biological interactions. To evaluate this interaction, it is necessary to know the basic biology of the taxa and to make use of statistical tools that identify the relationships between the biological groups.
This study aims to identify the effects of environmental variations and changes in the phycoperiphyton structure on the macroinvertebrate community in regulated Andean rivers. Given the regulation scenario, our starting hypothesis is that changes in phycoperiphyton density and diversity in these systems would have an equal or greater effect than environmental variability on macroinvertebrate abundance.
Materials and methods
Study area: Punchiná is a hydroelectric generating reservoir, with a storage capacity of 62 million m3, located at 750 meters altitude in the eastern part of the department of Antioquia in Colombia (6º12'39'' N & 74º50'26'' W) in the municipalities of Guatapé and San Carlos (Fig. 1). The reservoir and its tributary basins (Guatapé and San Carlos rivers) belong to the Holdridge (1979) ''lower montane tropical humid forest'' life zone with temperatures between 19 °C and 23 °C and average annual rainfall between 1 800 mm and 2 500 mm (Forero et al., 2014). It is a region influenced by the intertropical convergence zone (ITZ) that receives high radiation and presents interaction between the northeast and southeast trade winds, favoring condensation and precipitation. A bimodal precipitation regime generates the distribution of rainfall in two wet periods (April to May and October to November) and two dry periods (December to March and June to September) (Barrera-Olarte, 2018). The soils are of low fertility, acid, stony, and easily erodible, it is a forest vocation land (CORNARE, 2010). Before emptying in the Punchiná reservoir the San Carlos and Guatapé rivers can be characterized by relatively deep, turbid waters with a laminar flow and few emerged substrates or litter accumulations (Ríos-Pulgarín et al., 2020). Both tributaries are regulated by a complex system of reservoirs: A second reservoir is found upstream on the Guatapé River, and the San Carlos River receives turbinated water from a third reservoir through a tributary river.
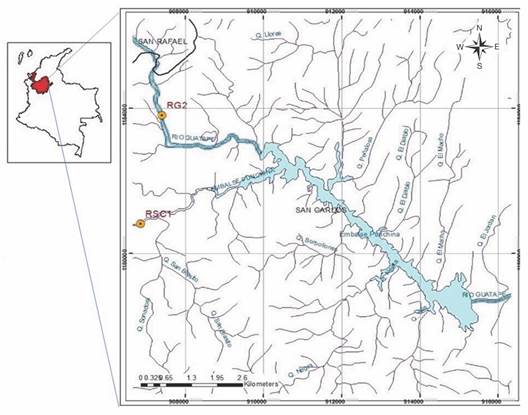
Fig. 1 Sampling sites in the tributaries of the Punchiná reservoir. Modified from Ríos-Pulgarín et al. (2020).
Sampling design: This study was carried out between 2010 and 2018 in three annual sampling periods during different hydrological periods: dry season (June), transition (September) and rainy season (November) for a total of 27 samples. In the Guatapé-RG2 and San Carlos-RSC1 rivers, sections of 100 meters each were selected to establish the sampling sites. The El Niño-Niña/Southern Oscillation (ENSO) conditions for each year were defined according to the NOAA-NCEP ONI (Oceanic Niño Index) report (2014) for the American Pacific and comparisons between the mean historical monthly precipitation of the study basin during the sampling periods (IDEAM, 2018). Based on this information, the periods 2010-2011 and 2016 were defined as La Niña, 2012-2014, 2017 and 2018 as neutral, and 2015 as El Niño.
Sampling: At each sampling sites, the temperature (oC), dissolved oxygen (DO) (mg/l), the percentage of oxygen saturation (%), pH, electrical conductivity (µS/cm) were measured with a Hach HQ40D multi-parameter instrument. Water samples for ex situ analysis of turbidity (N.T.U.), solids (mg/l) (suspended, dissolved and total), total iron (mg Fe/l), nitrites (mg NO2-/l), nitrates (mg NO3-/l), ammoniacal nitrogen (mg NH3/l), total phosphorus (mg P/l), orthophosphates (mg PO4 -3/l), and chemical oxygen demand (COD mg/l) were taken. In all cases, we followed standard methods for examination of water and wastewater using the 22nd edition (Baird & Bridgewater, 2017) and we collected biological samples (macroinvertebrates and phycoperiphyton).
The phycoperiphyton samples were collected in ten substrata immersed in the bed along the 100 m stretch, using plastic brushes and 10 cm2 quadrants until obtaining a scraping area of 100 cm2 per site. Samples were stored in plastic containers and preserved the contents with Lugol's solution. Macroinvertebrates were collected along the same stretch, using a D-net with a 400 µm mesh eye in areas with submerged vegetation, a screen net in sandy areas (both with 0.5 mm mesh eye), and manual sampling on substrates such as rocks or logs using tweezers to form an integrated sample, with a standardized effort of 20 minutes/rig/sample, for a total of one hour of sampling per sample. The specimens were stored in glass PET bottles with 70 % alcohol.
Laboratory work and identification: Both communities were analyzed in the Limnology Laboratory of the Universidad Católica de Oriente. Taxonomic determination of phycoperiphyton was carried out following the keys of Bicudo and Menezes (2006), Guiry & Guiry (2018), and Ramírez (2000). The count of individuals per species was made using an Olympus CKX41 inverted microscope following the methodology of 30 random fields (Lund, et al., 1958) and a magnification of 40X 10. Density is reported as individuals per square centimeter (ind/cm2) (Ross, 1979). Taxonomic determination of the macroinvertebrates was made to the lowest possible level following the keys of Aristizábal (2017), Manzo & Archangelsky (2008), McCafferty (1981), Merrit et al. (2008), and Posada & Roldán (2003). The count was carried out using a Carl Zeiss Stemi 508 stereo microscope at a magnification of 10X 40 and included the individuals collected with the different instruments accumulated per sample and expressed as abundance/sample. The organisms were deposited in the macroinvertebrate reference collection of the Universidad Católica de Oriente CM-UCO and were also registered with the Alexander von Humboldt Biological Resources Research Institute of Colombia using the Darwin Core format (https://ipt.biodiversidad.co/sib/resource?r = uco-002). Based on secondary information, each taxon was assigned to one of the five trophic functional groups (GT), according to the classifications of Silva-Poma & Huamantinco-Araujo (2022), Ríos-Pulgarín et al., (2016), Chará-Serna, et al. (2010) and Tomanova et al. (2006). The five groups were as follow: CF (filterers), CG (gatherers), SH (shredders), SC (scrapers) and PR (predators). In those cases, in which the genus included species with trophic flexibility or with different habits, the corresponding combinations were presented, for example, as CF-CG.
Data analysis: Biological variables were used to establish the basic structure of the communities through the Hill numbers, in terms of abundance or density, richness (Q0), diversity of common species equivalents (Q1), and dominant species (Q2) (Moreno, 2001), using the PAST 3.12 Software (Hammer, et al., 2001) The abundance of groups of macroinvertebrates, indicators of environmental water quality, was estimated, categorizing the organisms into the following groups: low quality due to organic contamination (non-arthropods Gastropoda, Bivalvia, Clitellata and Diptera), high quality (ETP Ephemeroptera, Plecoptera, Trichoptera), and variable tolerance in intermediate scales (other arthropods). The effect of the site and sampling period on the variability of the environment and the community in terms of richness, diversity, and abundance or density was established by creating generalized linear models (GLMs) for each of the variables. The independent variables (factors) were the sampling site, the hydrological period, the year, and the ENSO phenomenon; and the dependent variables included all physical and chemical variables, as well as the richness and abundance or density of macroinvertebrates and phycoperiphyton. For each parameter, the models with the lowest AIC values (Akaike, 1974) were selected, in this case those of the Gaussian family (log) for both environmental and biological variables. Significances greater than 95 % were considered for the FFM model estimators for each parameter, corresponding to the positive or negative effects of the factors on the dependent variables. GLM analyzes were done using R-project software, version 3.6.3. (RStudio Team (2020). The environmental and biological variables that showed a significant response to the factors evaluated using the GLM analyzes were included in the canonical discriminant analysis (ADC). This is an analysis that creates a function capable of classifying factors (sites, years, or ENSO phenomena), considering a series of discriminating variables (in this case, environmental variables, and the taxonomic composition of benthic organisms). The percentage of correctly classified data was obtained through cross validation. The spatial and temporal aggregation of the trophic guilds of macroinvertebrates and algal divisions was visually explored by a multidimensional scaling analysis (MDS) using the Bray-Curtis similarity index as a measure of distance. Both ADC and DMS analyzes were done using RWizard software version 4.3 (Guisande et al. al., 2014).
The relationship between environmental and biological variables, as well as the relative effect of trophic resources (phycoperiphyton) and environmental variables, were evaluated using the factors shaping community assemblages (FCA) analysis that is based on the instability of the environment (understood as the variability in environmental parameters) and the abundance and/or density of individuals of the biological group of interest, in order to find the factors that most influence the spatio/temporal changes of each of the groups functionally or taxonomically considered (Manjarrés-Hernández et al., 2021). For this, a principal component analysis (PCA) was carried out by year and sampling site, using the software past V 2.17 (Hammer et al., 2001) that allowed a determination of the trophic guilds of macroinvertebrates and the environmental variables that provide a greater contribution on the variance, based on the Jolliffe criterion (1972, 1973). Taxa of 18 orders of macroinvertebrates classified according to their trophic guild (corresponding to 73 % of the total abundance of macroinvertebrates), and 4 phycoperiphyton divisions (corresponding to 99 % of the density) were conserved. With these results, the fluctuation index of Dubois (1973) was estimated, modified by Guisande et al. (2006) to calculate the instability of the phycoperiphyton divisions, of the trophic guilds of macroinvertebrates in the environment.
Where s is the number of variables, pi the relative proportion of variable i at a specific time or space, pi the reference state, which is calculated as the average of the relative proportions for variable i during the study period or considering the sampling sites. The FCA analysis used artificial intelligence algorithms in the R package randomForest (Liaw & Wiever, 2018) to determine the relationship between environmental instability and the instability of the phycoperiphytic community, as well as the relationship between the selected groups of the two benthic communities. 10 % of the data was not included in the model for its validation. Likewise, the FCA used the R relaimpo package (Grömping, 2006; Grömping, 2021) to find the contribution of each one of the phycoperiphyton taxa to the changes in the abundance of macroinvertebrates and the contribution of each one of the physicochemical variables to the changes in the instability index of the phycoperiphyton divisions by means of hierarchical partitioning (Chevan & Sutherland, 1991). The analysis was performed in the RWizard version 4.3 software (Guisande, et al., 2014).
Results
Environmental Variability: The GLM analyzes made it possible to determine that at a temporal level in 2012, 2013 and 2015 there was a decrease (negative effect) in the concentrations of DO (average 6.96 -7.34mg/l) and NO3- (average 0.15-1.59 mg/l). Likewise, on a spatial scale a reduction in temperature (22.02 °C) and electrical conductivity (31.43 µS/cm) was observed at the RSC1 site, with the pH (7.42 pH Units) and TSS (31.05mg/l), the latter values closely related to turbidity. In all these cases the model was significant with P < 0.05 (Table 1 and Fig. 2). According to the FCA hierarchical partitioning analysis that estimates the contribution of variables to environmental instability both at a spatial and temporal level (Fig. 3), turbidity and PO4 -3 contributed 39.83 and 62.67 % to environmental instability, thus accumulating more than 90 % of the contribution (R2 = 0.89).
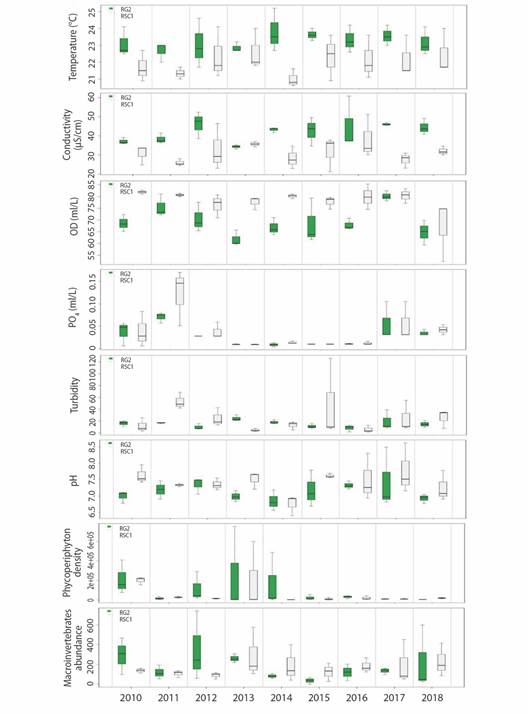
Fig 2 Spatial and temporal behavior of the biological and environmental variables that showed variability in the RG2 (Guatapé River) and RSC1 (San Carlos River) sites, between 2010 and 2018.
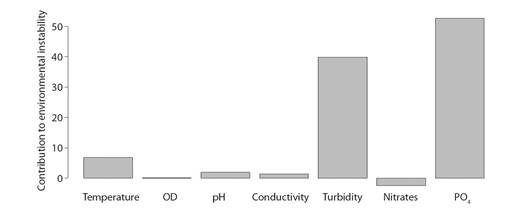
Fig. 3 Contribution of physicochemical variables to instability of the environment using the hierarchical partitioning method, R2 = 0.89.
Variability of the phycoperiphytic community: A total density of 3 973 809 ind/cm2 was recorded, distributed in 8 divisions, 13 classes, 37 orders, 65 families, and 122 taxa. The divisions that contributed the most to the total density were Bacillariophyta and Cyanobacteria with relative densities of 61 and 32 %; the genus Oscillatoria sp. registered the highest relative density (17.73 %), followed by Navicula sp. (15.03 %), Achnanthes sp. (15.01 %), Lyngbya sp. (11.11 %), Synedra sp. (6 %) and Gomphonema sp. (5 %). The RSC1 site showed significantly less diversity (negative effect) (P < 0.04). In 2013 and 2017, the lowest Q0 diversity values were also observed, with minimums of up to 8 taxa and an average between 14 and 15 (Table 1). In 2013 the density increased (positive effect), with an average of 251 439 ind/cm2 in RG2 and 20 678 ind/cm2 in RSC1. In conrast, in 2014 the lowest value was recorded with an average of 2 359 ind/cm2 in RSC1 (Table 1).
Table 1 Average, minimum, and maximum values, coefficient of variation and significance of the generalized linear model (MLG) for the biological and environmental variables during the years of the ENSO periods, and sites studied: RG2 (Guatapé river), RSC1 (San Carlos River).
Variable | RG2 | RSC1 | P Values | |||||||||||||
Mean | Range | CV | Mean | Range | CV | Y | P | S | P | E | ||||||
Temp. | 22.6 | 21.7 | 25.2 | 0.049 | 22.6 | 20.6 | 24.1 | 0.049 | - | RSC1 | 0.00006 | - | ||||
DO | 7.36 | 5.94 | 8.24 | 0.11 | 7.37 | 5.24 | 8.53 | 0.104 | 2012 2013 2015 | 0.037 | RSC1 | 0.00002 | - | |||
Cond. | 36.76 | 33.1 | 60.70 | 0.23 | 36.61 | 21.24 | 51.10 | 0.23 | - | RSC1 | 0.00002 | - | ||||
pH | 7.28 | 6.56 | 8.50 | 0.06 | 7.28 | 6.40 | 8.63 | 0.06 | - | RSC1 | 0.012 | - | ||||
NO3 | 1.03 | 0.16 | 1.94 | 1.03 | 1.02 | 0.03 | 2.03 | 0.53 | 2015 | 0.0000834 | - | - | ||||
Phyco.dens | 92043.00 | 546.47 | 751954.08 | 20756.00 | 55135.08 | 841.02 | 598595.02 | 2.11 | 2013 | 0.0337 | RSC1 | 0.00009 | - | |||
Q0 | 22.74 | 9 | 48 | 0.39 | 17.48 | 1 | 29 | 0.39 | 2017 | 0.0044 | RSC1 | 0.0039 | - | |||
2013 | 0.0399 | |||||||||||||||
Q1 | 6.17 | 1 | 11.77 | 0.41 | 61.22 | 1 | 11.77 | 0.42 | - | RSC1 | 0.036 | - | ||||
Q2 | 4.11 | 1 | 8.98 | 0.45 | 4.08 | 1 | 8.97 | 0.46 | - | RSC1 | 0.0123 | - | ||||
Macro.Abun | 175 | 0 | 742 | 0.9 | 178 | 28 | 575 | 0.88 | 2011 | 0.0011 | - | - | ||||
Q0 | 7 | 0 | 10 | 0.25 | 7 | 4 | 9 | 0.20 | 2011 | 0.00668 | - | - | ||||
Q1 | 6 | 0 | 9 | 0.24 | 6 | 3 | 8 | 0.19 | 2011 | 0.0117 | RSC1 | 0.0129 | - | |||
Q2 | 5 | 0 | 8 | 0.24 | 5 | 3 | 7 | 0.19 | 2011 | 0.0273 | RSC1 | 0.0024 | - | |||
2014 | 0.0398 | |||||||||||||||
2015 | 0.0182 | |||||||||||||||
2018 | 0.0249 |
*The models with the best fit and the lowest AIC criteria for the environmental variables and for the benthic communities were of the Gaussian family (log). Abbreviations: Y = years, S = sites, E = ENSO. temp = water temperature, DO = dissolved oxygen, Cond = electrical conductivity, pH, NO3 = nitrates, Fico.dens = ficoperiphyton density, Q0 = richness, Q1 = equivalent diversity of common species, Q2 = dominant species, Macro.Abun = abundance of macroinvertebrates.
Variability of the community of aquatic macroinvertebrates: The total abundance of macroinvertebrates was 9 465 individuals. These were classified as 136 taxa, 64 families, 18 orders, 6 classes, and 4 phyla. In the RG2 site, ETP organisms represented 30 % of the total abundance, non-arthropods 43.6 %, and other arthropods 26.3 %. The most abundant genera were Melanoides (Neotaenioglossa) and Leptonema (Trichoptera). At the RSC1 site, the most representative groups were the ETP with 60.5 % of total abundance and other arthropods with 37.5 %. During the study, the most abundant genera were Tricorythodes (Ephemeroptera) and Rhagovelia (Hemiptera). The scraper-filter feeders (CF-SC), collector-shredders (CG-SH), and predators (PR) were the ones with the highest contribution to the total abundance (63 %). In the temporal scale, abundance was relatively similar, although it increased in 2013 and 2016 in RSC1 and between 2010 and 2013 in RG2. The MGL identified a significant negative effect on the diversity of dominant species (Q2) in the years 2011, 2014, 2015 and 2018 (P < 0.002), especially in RSC1.
Patterns of environmental and biological stability: The discriminant analysis obtained more than 90.7 % of cases correctly identified by cross validation, except when the sampling years were analyzed, where only 50 % of the cases were correctly identified. No patterns of seasonal variation were found. Discrimination between sampling sites (100 % variance explained by the first canonical axis) confirmed spatial environmental differences that coincided with changes in macroinvertebrate composition (Fig. 4). The RSC1 site was dominated by ETP organisms and other arthropods that were associated with high concentrations of DO and TSS (total suspended solids). In contrast, at the RG2 site non-arthropod organisms stood out, associated with a higher temperature and electrical conductivity. On the time scale, only the year 2013 is discriminated due to the increase in Cyanophytas and Bacilliariophytas, but with an explained variance of less than 50 %. While for the ENSO periods, the discriminant analysis explained 84 % of variance in the first canonical axis, the La Niña phenomenon was differentiated by the greater presence of Charophyta and Chlorophyta, coinciding with a high concentration of nutrients (NO3 - and PO4 -3) and DO. The Bacillariophyta, Cryptophyta, Cyanobacteria, Euglenozoa, Miozoa, and Ochrophyta divisions as well as all macroinvertebrate groups were associated with drier El Niño periods and neutral periods associated with increases in electrical conductivity and temperature.
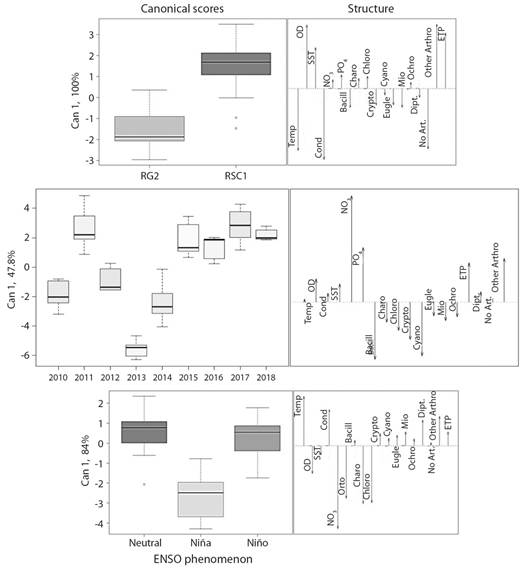
Fig. 4 Discriminant analysis between sampling sites and times, based on environmental variables and composition of benthic species. Abbreviations: Temp = water temperature, DO = dissolved oxygen, TSS = total suspended solids, Cond = electrical conductivity, NO3 = nitrates, PO4 = orthophosphates, Bacill = Bacillariophyta, Charo = Charophyta, Chlo = Chlorophyta, Crypto = Cryptophyta, Cyano = Cyanobacteria, Eugle = Euglenozoa, Mio = Miozoa, Ochro = Ochrophyta, Dipt = Diptera, No Art. = no arthropods, Other Art. = other arthropods, ETP = Ephemeroptera, Trichoptera, Plecoptera.
The EMD analysis also identified an aggregation of benthic communities depending on the sampling site (stress value of 0.068). According to this analysis, spatial differences prevailed in the density of algal divisions and the abundance of macroinvertebrate trophic guilds. At the RG2 site an aggregation pattern of collector-filter and collector-scraper organisms was observed, while at the RSC1 site the guilds were more abundant, the same as the phycoperiphyton (Fig. 5).
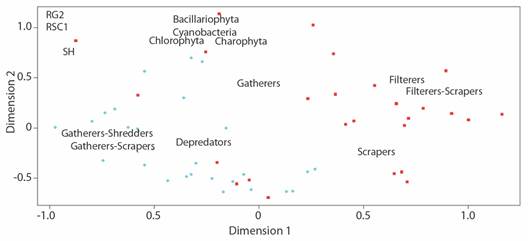
Fig. 5 Multidimensional scaling representing the aggregation of biological data, according to the sampling site, based on the composition of benthic communities.
According to the results of the principal component analysis, four phycoperiphyton divisions, eight macroinvertebrate trophic guilds, and a total of seven physicochemical variables were included in the instability analysis. Table 2 shows the greatest contributions of environmental variables and phycoperiphyton to the instability of the macroinvertebrate community. For both environmental and biological variables, the model shows an R2 greater than 83 %. The trophic guilds that presented the greatest response to environmental instability were scrapers (SC) and filter feeders (CF) that on the spatial scale responded negatively to oxygen and turbidity. On the time scale these groups responded negatively to conductivity and NO3 - but to a much lesser extent. The collector-shredders (CG-SH) responded only in the spatial scale, positively to turbidity and negatively to DO.
Table 2 Interaction between the instability of the physicochemical variables, the instability in the algal density, and the abundance of macroinvertebrates in tributary rivers of the Punchiná reservoir (based on artificial intelligence algorithms from the FCA analysis).
Contribution | |||||||||
Environmental Variables | r2 | Resource (Divisions) | r2 | ||||||
Stability | Temporal | Variables | SC | CF.SC | 0.89 | Variables | SC | 0.84 | |
Temperature | 67.5 | -3.6 | Bacillariophyta | 252.990958 | |||||
pH | 112.3 | 90.9 | Charophyta | -396.76851 | |||||
Conductivity | -169 | 81.5 | Chlorophyta | -642.055658 | |||||
Turbidity | 112.5 | 108.3 | Cyanobacteria | 885.83321 | |||||
Nitrates | 48.3 | -162.7 | |||||||
spatial | Variables | CG.SH | CF.SC | 0.88 | Variables | SC | 0.83 | ||
OD | -2 171.3 | -1 702.8 | Bacillariophyta | 1 309.26547 | |||||
pH | 859.676 | 1 309.258 | Charophyta | -663.071129 | |||||
Turbidity | 3 032.89 | -1 189.85 | Chlorophyta | -2 382.15325 | |||||
Cyanobacteria | 1 835.9589 |
Abbreviations: SC = Scrapers, CG.SH = Gatherers-Shredders, CF.SC = Filterers-Scrapers, CG-SH = Gatherer-Shredders.
The contribution of algal divisions to the instability of macroinvertebrates only affected the guild of scrapers (SC), although significantly higher than the environmental contribution and at both a spatial and temporal level. The divisions Charophyta and Chlorophyta showed a higher and negative contribution to the instability of the scrapers, while Cyanobacteria make a high positive contribution to this instability, both at the spatial and temporal levels. The effect of Bacillariophyta is low at the temporal level and moderate at the spatial level (Table 2).
Discussion
The physicochemical parameters showed, for the most part, relative stability as expected from environmental variability in regulated systems. The pH showed adequate values for the establishment of most aquatic species (6-8 pH units) (Arango et al., 2008). The temperature was characteristic of the tropics (annual average higher than 18 °C and a maximum of 28 °C) (López et al., 2012). According to the DO concentration (> 5 mg/l) and the chemical oxygen demand (< 27 mg/l), both sites offered adequate conditions for aquatic life (Gualdrón-Durán, 2018; Roldán-Pérez & Ramírez, 2022) consistent with the high slope that favors oxygenation (Rivera, 2004). Likewise, inorganic nutrients were found in relatively low concentrations that do not show conditions of enrichment.
No effect of hydrological seasonality was detected, probably a consequence of regulation; however, moderate temporal variations were observed to be associated with the ENSO phenomenon. This is the case of a decrease in the concentration of DO in the years 2012, 2013, and 2015 corresponding to the El Niño phenomenon at both sampling sites. The relationship between the concentration of nitrates with the runoff and the hydrological cycle manifested itself with an increase during the rainy periods of 2015. For the structure of the benthic communities, the algal community divisions Cyanobacteria and Bacillariophyta were the ones that most contributed to the total density. According to Casco and Toja (2003) and Passy (2007) the high densities of these divisions are related to their specialized growth forms that adhere to the substrates and that include different habits such as prostrate, erect, filamentous, chain-forming, or tubes and colonial, according to the availability of resources and disturbances such as flow oscillations (Passy, 2007; Ríos-Pulgarín et al., 2016). The most representative macroinvertebrates were Ephemeroptera, Trichoptera and Gastropoda, in relation to the supply of resources and habitat in each river.
At the spatial level, in the RSC1 site the lower values of diversity and density of phycoperiphyton reflect low environmental stability, favoring the growth of some genera such as Oscillatoria sp, Navicula sp., Cylindrocystis sp., Achnanthes sp., and Lyngbya sp. with adaptations to flow fluctuations and the availability of low-quality substrates. The composition and abundance of macroinvertebrates also showed spatial differences. The RSC1 site was characterized by a greater abundance of the orders Ephemeroptera, Trichoptera, Plecoptera and others of Arthropoda, favored by physicochemical and habitat characteristics such as the presence of substrates like trunks, submerged vegetation, shallower depth, and speed of the current (Ríos-Pulgarín et al., 2020). In contrast, in the RG2 site non-arthropod organisms predominated, especially molluscs of the orders Hygrophila and Neotaenioglossa that are favored by the presence of submerged vegetation and a high organic load (Baqueiro-Cárdenas et al., 2007; Vogler et al., 2012). The discriminant analysis corroborated the differences between sampling sites and associated with the predominance of ETP macroinvertebrates and other arthropods in RSC1 with high concentrations of OD and TSS. The abundance especially of mollusks in RG2 coincided with conditions of higher temperature and electrical conductivity.
The effects of environmental regulation determined the response on the temporal scale; inorganic nutrients showed high concentrations during the La Niña period as a result of increased transport and fragmentation of particulate organic matter upstream of the sampling site (Reddy et al. al., 1999). The green algae of the Chlorophyta and Charofita divisions were related to the La Niña phenomenon, because they are favored by the increase in the concentration of nutrients (Eikland, et al., 2017., Sarmiento Morales, 2017). During the dry conditions of El Niño, in contrast, cyanobacteria (Cyanophyta) predominated, characterized by their ability to secrete mucilages that provide them with enough moisture to protect their reproductive structures (Casco & Toja, 2003) and diatoms (Bacillariophyta) that they are favored by stable conditions in the and an increase in temperature (Rivera Rondón & Donato, 2008). This condition favors the proliferation of certain groups of diatoms when there are sufficient nutrients available (Casco & Toja, 2003). On the other hand, the nutrients did not have significant effects on the algal densities since their concentrations were relatively stable. The effect of hydrological seasonality on benthic communities was only observable during the extreme conditions of the La Niña/ENSO phenomenon. The low incidence of hydrological disturbance favored diverse communities. Under conditions of relative environmental stability, biological interactions in the community structure gained importance. Biggs & Smith (2002), Díaz-Quirós & Rivera-Rondón (2004) and, Martínez & Donato (2003) have suggested that long-term periods of hydrological stability are necessary for the development of a phycoperiphytic community with greater species richness maintaining assemblages in early stages of succession since hydrological disturbance periodically removes taxa that do not have adaptations to trawling (Ríos-Pulgarín et al., 2016). The increase in the speed of the water during the La Niña period generates drag; therefore, most of the benthic organisms thrive in the dry period of El Niño (Cantonati & Spitale 2009; Carmona-Jiménez et al., 2016). These organisms determined the differences in the values of density or abundance during periods when environmental control predominates. In the absence of such controls, the FCA results suggested a significant effect of phycoperiphyton on the macroinvertebrate community.
According to Fenoglio et al., (2020), the most obvious trophic relationship between benthic communities is grazing, carried out by herbivorous invertebrates from biofilms on substrates. So, in addition to habitat conditions and physical and chemical factors, the density of periphytic algae depends on the diet of scraping macroinvertebrates that are frequently found in the ETP group (Tierno de Figueroa, 2019). Among these, the families Baetidae, Leptohyphidae, and Leptophlebiidae (Ephemeroptera), Perlidae (Plecoptera) stand out, as well as the families Hydroptilidae, Glossosomatidae and Xiphocentronidae (Trichoptera) that according to Springer (2010), have genera that are phycoperiphyton scrapers. In the case of the order Plecoptera, their diet is based mainly on diatoms, species that are easily digested by macroinvertebrates, due to the absence of lignin, even though their levels of proteins, lipids, and carbohydrates are relatively low (Bojorge-García & Cantoral-Uriza, 2016; Diaz-Villanueva, 2001). For the order Trichoptera scraping algae is one of the most common sources of food acquisition, with chewing-type mouthparts, sucking algae cell contents (Wiggins, 2004). Organisms of the order Ephemeroptera are also scraper or collector herbivores and feed on algae and tissues of aquatic plants (Flowers & De la Rosa, 2010). Another group that has effects on the richness and density of phycoperiphyton are the molluscs of the order Gastropoda and the species Melanoides tuberculate (Coat et al., 2009; Putz, 1997) that was particularly abundant in the study. Given that the relationship between these two communities is positive, we can assume that the control of the trophic web in these systems occurs from the producers (bottom up).
The higher density of phycoperiphyton in RSC1, coinciding with the lower abundance of collector-scraper or collector-filter macroinvertebrates and a greater abundance of predators, is consistent with a lower incidence of herbivory at this site, as demonstrated by Savic et al., (2018). This negative dependence on grazing has been documented using biomass as a response, but an indirect and positive effect of predation that decreases herbivores has also been found (Hillebrand, 2009, Holomuzki et al., 2010, Rakowski & Cardinale, 2016) and favors the increase of phycoperiphyton. In this sense, when analyzing the stability of the different communities, a possible effect of selective grazing of macroinvertebrates on phycoperiphyton is evident, since Charophyta and Chlorophyta decrease because of grazing, while cyanobacteria are less affected due to their mucilaginous cover, production of toxins, and/or lower nutritional value. For its part, Bacillariophyta, thanks to high reproduction rates, are abundant and constant, so they do not show significant variations in supply. Dunk et al., (2018) have documented the functional responses of phycoperiphyton to grazing. According to these authors, the prevalence of algae with prostrate habits is since they tolerate herbivory better than filamentous or erect ones that are easily removed from the upper layer of the biofilm. However, some species of the scraper orders Gastropoda and Ephemeroptera could remove prostrate algae (Holomuzki et al., 2010). Although this dependency gains importance due to environmental stability, the results suggest that those variables that showed instability may exercise indirect environmental control over trophic guilds. This is the case of temperature that favors collectors and scrapers due to the increase in the supply of substrates. Turbidity, that negatively affects predators due to its dependence on vision, favors scrapers, shredders, and filter-feeding herbivores. So, the effects of grazing can vary according to the prevailing environmental conditions. Peters et al., (2007) suggest that aspects such as spatial heterogeneity associated with substrates, nutrient concentration, or hydrodynamic conditions restrict the trophic guilds that are established. This contributes to the incidence of herbivory in the RG2 site associated with the predominance of mollusks that are favored by higher concentrations of organic matter.
In summary, under conditions of environmental stability, the increase in the densities of phycoperiphyton would increase the scraping macroinvertebrates that use them as a food. Given the reduction in hydrological seasonality associated with regulation, at RG2 and RSC1 herbivory acted as a determining factor of the phycoperiphyton structure, and the effect of environmental variables was only detected during extreme conditions such as the ENSO, with temperature and turbidity as the main factors that presented greater instability and incidence on the trophic guilds. This produces a smaller effect of environmental instability than of instability of the supply of phycoperiphyton on the macroinvertebrate community.
Sampling sites showed little temporal variability in environmental conditions due to regulation. Under these conditions, phycoperiphyton was regulated mainly by herbivorous macroinvertebrates (scrapers), especially ETP arthropods and some Gastropoda mollusks. This trophic relationship was even more relevant than the physicochemical conditions of the environment in the structuring of the benthic communities, confirming the initial hypothesis and showing that in these systems the control of the trophic web occurs from the producers (bottom up), whose densities condition the abundance and composition of macroinvertebrates.
Ethical statement: the authors declare that they all agree with this publication and made significant contributions; that there is no conflict of interest of any kind; and that we followed all pertinent ethical and legal procedures and requirements. All financial sources are fully and clearly stated in the acknowledgments section. A signed document has been filed in the journal archives.