Introduction
Low water availability is one of the environmental factors that can most alter plant structures and behavior (Reddy et al., 2004). Among the strategies developed by plants to survive dry periods are improvements of antioxidant systems, changes in leaf osmotic potentials, as well as in the periods of growth and developmental of vegetative organs, adjustments of photosynthetic metabolism, and morphological such as increased leaf sclerophylly (Chaves et al., 2002; Reddy et al., 2004; Reich et al., 2003; Souza et al., 2015). Water deficits can induce the development of leaves with thick cuticles, low investments in mesophyll formation, and large investments in lignin and sclerenchyma that confer greater tissue density (Edwards et al., 2000; Lüttge et al., 1997; Salleo & Nardini, 2000; Sobrado & Medina, 1980; Souza et al., 2015). Different morphological characteristics reflect different adaptive processes to protect against high light intensities and long dry periods, while still ensuring efficient photosynthetic levels (Ehleringer & Mooney, 1978; Hoffmann et al., 2005; Nicotra et al., 2011; Reich et al., 2003).
The temporal patterns of plant growth observed in savanna environments are linked to climatic seasonality especially the rainy and dry seasons (Williams et al., 1997). The seasonality of water resources stimulates leaf fall in several species (Borchert et al., 2005; Morellato et al., 2000; Williams et al., 1997), although the real trigger of leaf phenology remains uncertain. Rapid decreases in soil moisture, the activities of the endogenous process such as stem rehydration (Reich & Borchert, 1984) and photoperiodism (Rivera et al., 2002) have been proposed to explain leaf fall. Despite that, there is a consensus that resource seasonality is the selective force behind distinct phenological strategies (Camargo et al., 2018; Eamus & Prior, 2001; Goldstein et al., 2008). Deciduousness can have an adaptive advantage when combined with other functional and structural characteristics (Rossatto, 2013), as deciduous species generally have leaves with shorter life spans (and therefore greater investment in photosynthetic tissues), lower specific leaf masses, and higher stomatal conductance and carbon assimilation rates (Eamus et al., 1999; Ishida et al., 2010). The leaves of evergreen species, on the other hand, generally are expected to have greater longevities, lower quantum yields, and evidence of greater water use efficiencies (Rossatto et al., 2013; Somavilla et al., 2014; Wei et al., 2016; Zhu et al., 2013).
Seasonal leaves may optimize carbon allocation during different seasons (Kitajima et al., 1997; Newell et al., 2002). Carbohydrates may be constituents of cell walls, can be stored as starch, or can be oxidized during respiration (as small soluble sugars) (Pallardy, 2008). Those soluble sugars can also decrease the osmotic potentials of the plants and increase the water-holding capacities of their tissues (Pallardy, 2008). Roots, stems, and leaves serve as storage sites for carbohydrates, but the storage and use of those resources appear to be related to the phenology of carbon gain (Pallardy, 2008). Maximum non-structural carbohydrate concentrations have been found when plant canopies are at their fullest among seasonal species (Newell et al., 2002) and, during any given season (dry or rainy), the leaves may evidence different patterns of sugar dynamics depending on their phenological status, which led us to analyze leaf sugar concentrations at different times during the same season.
Herein, three congeneric Byrsonima species from the Neotropical savanna were used as a biological study model to analyze variations in their physiological traits depending on the timing of their leaf phenophases. Byrsonima intermedia, B. coccolobifolia, and B. verbascifolia have been described as evergreen (Boas et al., 2013), deciduous (Silvério & Lenza, 2010), and brevideciduous (Kuster et al., 2017; Lenza & Klink, 2006) respectively. Phenological behaviors may change in different environments or during different periods (Borchert et al., 2005; Cuzzuol & Clippel, 2009; Rivera et al., 2002; Rossatto, 2013), which led us to examine the phenological patterns of those selected species in the field and address the following questions: (i) are there variations in plant functional traits during the same season? (ii) Are there relationships between the functional traits and the phenological statuses of the studied species? We used specific leaf mass to estimate investments in photosynthetic components (Reich & Schoettle, 1988), and their relative water contents to indicate the water status of the plant, chloroplastidic pigments, and fluorescence parameters to evaluate the photosynthetic apparatus and photochemical yields. We also examined the carbohydrate balances of the plants in each season, as well as the phenological stages of the leaves of each species.
Materials and methods
Study area and plant species: The present study was carried out in savanna areas (Cerrado sensu lato in Brazil) at the Panga Ecological Station (19º11'40'' S & 48º19'06'' W), a Private Natural Heritage Reserve (Reserva Ecológica do Panga) belonging to the Universidade Federal de Uberlândia (UFU) and located 30 km from Uberlândia in southeastern Brazil. The area has many Cerrado savanna plant formations, which include open formations (wetlands and savannas) and forest formations (dense Cerrado, evergreen forests, and gallery forests) (Gonçalves et al., 2021). The local climate is humid subtropical, with hot austral summers and dry winters (Cwa) (Alvares et al., 2014), with an average annual rainfall of apprximately 1 500 mm and a mean annual temperature of 23 ºC. Precipitation and temperature data were provided by the Meteorological Station of the Universidade Federal de Uberlândia and are presented in Fig. 1.
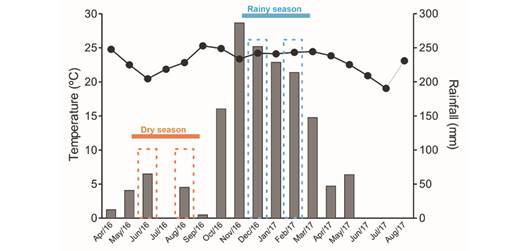
Fig. 1 The seasonal climate in the Brazilian savanna with its rainy and dry seasons. Mean temperature (line) and monthly rainfall rates (bars) from April/2016 to August/2017 are indicated. The periods of the physiological analyses are shown for the dry season (onset of the dry season-June/2016, and near the end of the dry season - August/2016) as well as the rainy season (onset of the rainy season - December/2016, and during the time of more prolonged rainfall-February/2017). The data were acquired from the Meteorological Station at the Universidade Federal de Uberlândia (Uberlândia, southeastern Brazil).
This study was undertaken in a fragment of Cerrado sensu stricto vegetation, the dominant phytophysiognomic formation in the reserve (Gonçalves et al., 2021). Twenty-five adult individuals of Byrsonima intermedia A. Juss and B. cocolobifolia H. B. & K., as well as 20 individuals of B. verbascifolia (L.) DC were randomly marked. Ten individuals of each species (n = 10) were used for the physiological analyses, considering one leaf per individual; the remaining individuals were accompanied during the phenological analyses (n = 15), except for B. verbascifolia (with n = 10), due to the low number of individuals of that species in the Cerrado fragment.
Fully developed leaves, without signs of herbivory, were collected both during the dry season and the rainy season for physiological analyses. Samples were collected at the onset of the dry period (June/2016) and again later in the dry season (August/2016), as well as at the onset of the rainy season (December/2016) and again later in the rainy season (February/2017) (Fig. 1). Only young leaves were collected from the three species in December/2016, as leaf sprouting was the principal phenophase of both B. intermedia and B. coccolobifolia (Fig. 2, Fig. 3).
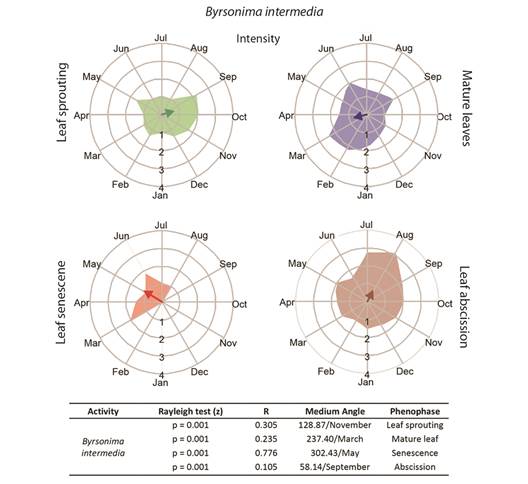
Fig. 2 Intensities (circular histograms) and activity indices (table) of the leaf phenophases evaluated for Byrsonima intermedia. The leaf sprouting was presented in green coloration, mature leaves in blue, senescence red, and abscission in brown coloration. The results of the Rayleigh (z) test, the mean angle concentration index (R), and the mean angle (indicating the month with the greatest vector) in each phenophase are listed in the table.
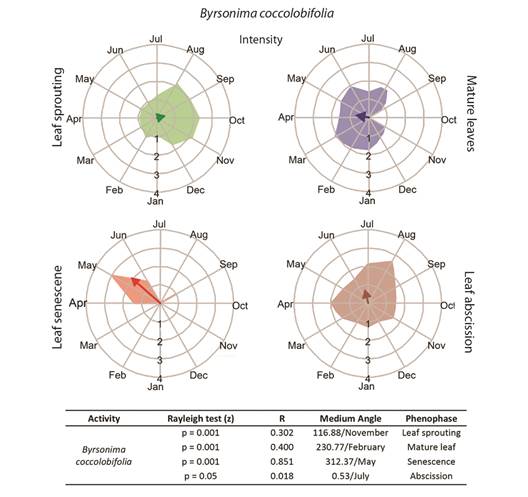
Fig. 3 Intensities (circular histograms) and activity indices (table) of the leaf phenophases evaluated in Byrsonima coccolobifolia. The leaf sprouting was presented in green coloration, mature leaves in blue, senescence red, and abscission in brown coloration. The results of the Rayleigh (z) test, the mean angle concentration index (R), and the mean angle (indicating the month with the greatest vector) in each phenophase are listed in the table.
Phenological analyses: The vegetative phenology of the three species studied was monitored every month. The presence or absence of foliar events (leaf sprouting, mature leaves, senescence, and abscission) were recorded for each plant. This method estimates the percentage of individuals in the population evidencing a given phenological event and indicates the synchronicity of that specific phenological event during the year (Morellato et al., 2010). The intensities of the foliar events (the expression of each phenophase by populations) were estimated for each plant according to Fournier (1974), using a semi-quantitative interval scale of five categories (0 to 4). The levels of leaf deciduousness were determined considering: (1) evergreen species, as those that retain their leaf areas approximately constant, or immediately recover them throughout the year (Borges & Prado, 2014; Camargo et al., 2018); (2) brevideciduous species, as those that retain approximately half of their leaves and quickly replace them during the same season (Borges & Prado, 2014; Lenza & Klink, 2006); and (3) deciduous species, as those that lose their leaves for a period of at least 30 days during the year (Borges & Prado, 2014; Damascos et al., 2005). This classification has been used for many tree species growing in the Brazilian savanna (Borges & Prado, 2014).
Specific leaf mass and relative water content: The specific masses and relative water contents of the leaves were obtained considering mature leaves (completely expanded leaves on the 2nd or 3rd node), except in December, when leaf sprouting was the predominant phenophase (and the most expanded leaf of the same season was used for the determinations). Specific leaf masses (SLM) and relative water contents (RWC) were determined using 0.5 cm2 leaf fragments (n = 10 per species). Those samples were weighed in order to obtain their fresh masses (FM), and then oven-dried at 60 ºC for 72 h to obtain their dry masses (DM). The SLM was obtained as the ratio between the dry mass (DM) and sample area (A) following Witkowski & Lamont (1991), and the sclerophylly index following Groom & Lamont (1999). RWC was obtained based on the FM, using the equation, according to Turner (1986).
Carbohydrate (starch, total soluble sugars, and water-soluble polysaccharides) contents and osmotic potential: Leaf samples from each species (n = 10) were oven-dried, ground, and homogenized, and 0.2 mg of each sample was centrifuged in 15 ml of methanol:chloroform:water (12:5:3) solution for total soluble sugar (TSS) extraction. The residues were resuspended in 5 ml of 10 % ethanol to determine their water-soluble polysaccharide (WSP) contents. Starch content was determined after further resuspension of the residue in 5 ml of 30 % perchloric acid. Carbohydrate content was determined by the phenol-sulfuric acid colorimetric method (Chow & Landhäusser, 2004; Dubois et al., 1956), using glucose as the standard. The absorbance of the characteristic yellow-orange color of the extracts was measured at 490 nm for hexoses (Dubois et al., 1956), using a spectrophotometer (BioSpectro, SP-220, São Paulo, Brazil).
The osmotic potential (Ys) was estimated using van't Hoff's equation as proposed by Niinemets & Kull (1998). In this equation, CAST is the total soluble sugar concentration (kmol kg-1), Dw is the dry mass (DM)/fresh mass (FM) (kg kg-1) ratio, s is the water density (kg m-3), R is the universal gas constant, and T is the temperature in K°.
Chloroplastidic pigment content: To obtain the chloroplastidic pigment contents, one leaf was collected from each plant (n = 10 per species), stored in a cool environment, and protected from light until extraction. Leaf disks (0.5 cm2 area) were immersed (under green light) in 5 ml of 80 % acetone for 48 hours. The samples were then grounded, and another 5 ml of 80 % acetone was added. The extract was subsequently centrifuged, and the supernatant subjected to spectrophotometry at 470, 643 and 663 nm (BioSpectro spectrophotometer, SP-220, São Paulo, Brazil). The Chlorophyll a, b and carotenoid (xanthophylls and carotenes) contents were calculated according to Lichtenthaler & Wellburn (1983).
Photosynthetic efficiency (chlorophyll a fluorescence): Photosynthetic efficiency was assessed by the chlorophyll a fluorescence method, using a modulated fluorometer (MINI-PAM, Walz, Germany). Measurements were performed on eight individuals of each species (n = 8) between 8:00 and 11:30 am. The maximum quantum yield (F v /F m ) values of photosystem II (PSII) were determined after leaf adaptation to the dark for 30 min, with F v corresponding to the difference between the maximum (F m ) and minimum (F 0 ) fluorescence of the dark-adapted PSII (Genty et al., 1989).
Data analysis: The seasonality of activity and the intensities of the phenology data were tested, and the mean angles and the concentration coefficients (r) of the phenophases (considering their mean periods) were calculated using the Rayleigh (z) test for circular distributions (Zar, 1998). The concentration coefficient refers to the length of the vector, and the mean angle of that vector corresponds to the mean date for the period around which a phenophase activity is most concentrated. When the mean angle is statistically significant, it means that the phenophase demonstrates seasonality. The concentration of the mean angle, indicated by (r), can be considered a measure of the degree of seasonality (Morellato et al., 2000). The circular tests were performed using the R software package (R Core Team, 2014).
The specific leaf mass, relative water content, carbohydrate content, osmotic potential, and chloroplastidic pigment content data were evaluated by determining the existence of interactions between predictor variables (months and species). Differences between months and between species were determined using generalized linear models (GLM) with Gaussian distributions, with R software. Fisher's F test (R Core Team, 2014) and resources of the Rcmdr package (Fox, 2005) were used. For the variables whose F tests were significant, the means among species and among months were compared by the RT4Bio package test (Reis-Junior et al., 2015).
For fluorescence data analysis, the existence of interactions between predictor variables (months and species) and differences among months and species were determined by generalized linear mixed models (GLMM) using R software (R Core Team, 2014). Data normality was tested, followed by data log transformation, and the Tukey test was applied to determine variations among the different months.
Results
Leaf phenology: Byrsonima intermedia and B. coccolobifolia were classified as brevideciduous because they conserve up to 50 % of their leaves throughout the year (Fig. 2, Fig. 3); B. verbascifolia was classified as evergreen because it keeps most of its leaves throughout the year (Fig. 4). The individuals of each species showed synchronization of most of their phenophases, except leaf abscission in B. coccolobifolia. The concentration index of the mean angle (r) was low for all three species during sprouting, mature leaves, and abscission, thus indicating poor synchrony of the populations during those phenophases. Byrsonima intermedia and B. coccolobifolia produced new leaves throughout the year, but their production was more concentrated in September-November (Fig. 2, Fig. 3). Byrsonima verbascifolia, on the other hand, showed clear seasonal sprouting, senescence, and abscission, with no leaves retained between August and September, and new leaf production starting at the end of September (P ≤ 0.05, R = 0.541, and mean angle = 124.0) (Fig. 4).
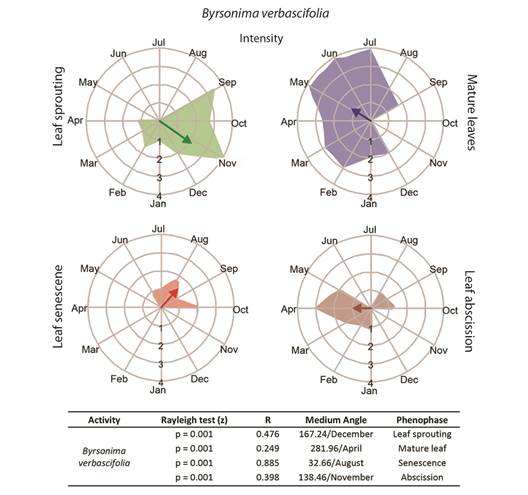
Fig. 4 Intensities (circular histograms) and activity indices (table) of the leaf phenophases evaluated in Byrsonima verbascifolia. The leaf sprouting was presented in green coloration, mature leaves in blue, senescence red, and abscission in brown coloration. The results of the Rayleigh (z) test, the mean angle concentration index (R), and the mean angle (indicating the month with the greatest vector) in each phenophase are listed in the table.
Variations of functional traits: Interactions between months and species were observed for many of the functional traits analyzed (water-soluble polysaccharides - F(6,95) = 2.95, P ≤ 0.05, total soluble sugar - F(6,95) = 8.89, P ≤ 0.001, and osmotic potential based on it - F(6,95) = 5.19, P ≤ 0.001, and total chlorophyll content - F(6.97) = 2.28, P ≤ 0.5), showing the connection between the timing of each leaf phenophase and the leaf phenological pattern of each species.
No differences in SLM values were observed when comparing months or species separately (Fig. 5A), although the relative water content values of B. intermedia showed lower values at the onset of the rainy season (December, F(3,34) = 7.60, P ≤ 0.001), as compared to the other months (Fig. 5B).
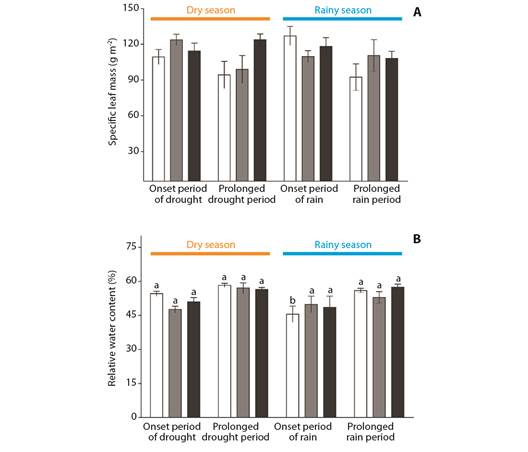
Fig. 5 A. Specific leaf mass-SLM, and B. B. and relative water content-RWC of Byrsonima intermedia-white bars, Byrsonima coccolobifolia-gray bars, and Byrsonima verbascifolia-black bars. Samples were collected at the onset of the dry season (June), after the prolonged dry season (August), as well as at the onset of the rainy season (December) and after the prolonged rainy season (February). Data are reported as the mean ± standard error of the mean. Lower case letters represent differences between months (seasons). The absence of any letter signifies the absence of any difference between those factors.
Considering the analysis of carbohydrates, the three species showed differences in their starch contents in terms of the timing of the seasons (Fig. 6A). Byrsonima intermedia and B. verbascifolia had lower starch contents at the onset of the rainy season (December) than during the other periods evaluated (F(3,28) = 3.73, P ≤ 0.05; F(3,35) = 5.93, P ≤ 0.01 respectively). Byrsonima occolobifolia also evidenced its lowest starch contents during the prolonged dry season (August) as well as at the onset of the rainy season (December) (F(3,32) = 3.26, P ≤ 0.05), showing that this carbon resource may be more related to the phenological status of the leaves than to the season. Water-soluble polysaccharide (WSP) concentrations were also different only in terms of the timings of the seasons (Fig. 6B). Byrsonima intermedia had the highest WSP levels during the prolonged dry season (August) and after the prolonged rainy season (February) (F(3,28) = 38.46, P ≤ 0.001), while B. coccolobifolia and B. verbascifolia had their highest WSP levels during the prolonged rainy season (February, F(3,32) = 33.82, P ≤ 0.001; F(3,35) = 11.33, P ≤ 0.001, respectively). The lowest values were observed at the onset of the dry season (June) for all the species.
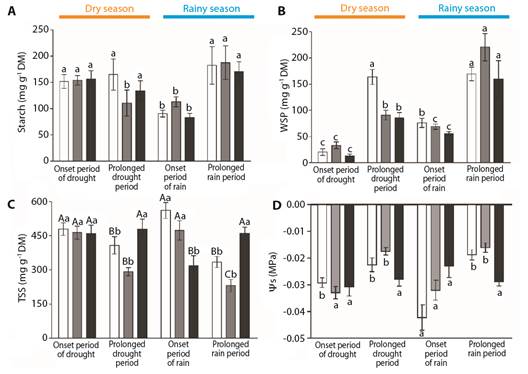
Fig. 6 Carbohydrate content in three Byrsonima species. Samples were collected at the onset of the dry season (June), after the prolonged dry season (August), at the onset of the rainy season (December), and after the prolonged rainy season (February). A. Starch content. B. Water-soluble polysaccharides (WSP). C. Total soluble sugars (TSS). D. Osmotic potentials (Ys). Data are reported here as the mean ± standard error. Lower case letters represent differences between months (seasons), while upper-case letters represent differences between species. Byrsonima intermedia-white bars; Byrsonima coccolobifolia-gray bars; and Byrsonima verbascifolia-black bars.
Regarding the total soluble sugar (TSS) results (Fig. 6C), differences were observed among species and among the timings of the seasons. Byrsonima verbascifolia had higher values than the other species during the prolonged dry season (August) (F(2,17) = 7.22, P ≤ 0.01) and after the more prolonged rainy season (February) (F(2,25) = 18.7, P ≤ 0.001), with its lowest values at the onset of the rainy season (December) (F(2,27) = 9, 07, P ≤ 0.001). Comparing the timing of the seasons, B. intermedia and B. coccolobifolia showed oscillations during the year, with the highest TSS values at the onset of both the dry season (June) and the rainy season (December) (F(3,28) = 10.54, P ≤ 0.001; F(3,32) = 14.76, P ≤ 0.001 respectively). Byrsonima verbascifolia had the lowest TSS levels at the onset of the rainy season (December) (F(3.,5) = 3.80, P ≤ 0.05). Osmotic adjustments based on total soluble sugars (Ys) (Fig. 6D) showed differences only among months. Byrsonima intermedia had the lowest Ys values at the onset of the rainy season (December) (F(3,28) = 9.61, P ≤ 0.001), while B. coccolobifolia evidenced oscillations, with the lowest values at the onset of the dry season (June) and at the onset of the rainy season (December) (F(3,32) = 12.50, P ≤ 0.001). Byrsonima verbascifolia evidenced no differences among the different months.
Comparing the three species, differences could be observed in their total chlorophyll contents-TCC (Fig. 7A) and total chlorophyll/carotenoid ratios (Fig. 7B), reinforcing the different patterns among the different species and oscillations within the same season. The different patterns among the different species could be detected by observing that Byrsonima intermedia had higher TCC values than the other species during the prolonged dry season (August) and after the prolonged rainy season (February) (F(2.22) = 6.26, P ≤ 0.5, F(2.27) = 3.43, P ≤ 0.5, respectively) (Fig. 7A). The highest values of the total chlorophyll/carotenoid ratios were observed in B. verbascifolia at the onset of the rainy season (December) (F(2.25) = 5.44, P ≤ 0.5) and in B. intermedia after the prolonged rainy season (February, F(2.27) = 7.11, P ≤ 0.5) (Fig. 7B). There were no differences in carotenoid contents or chlorophyll a/b ratios among the different species (Fig. 7C, Fig. 7D). Comparing the months, it was possible to detect changes in the total chlorophyll content (Fig. 7A), total chlorophyll/carotenoid ratio (Fig. 7B), and carotenoid content (Fig. 7C). Byrsonima intermedia evidenced its lowest total chlorophyll content value during the prolonged dry season (August-F(3,34) = 7.82, P ≤ 0.5), while B. coccolobifolia had its highest chlorophyll levels at the onsets of the dry (June) and rainy seasons (December) (F(3,30) = 11.30, P ≤ 0.5) (Fig. 7A); Byrsonima verbascifolia evidenced oscillating values throughout the year, with its highest total chlorophyll content being observed at the onset of the dry season (June) (F(3,105) = 27,54, P ≤ 0,5) (Fig. 7A). Considering the chlorophyll/carotenoid ratio, Byrsonima intermedia and B. coccolobifolia had their lowest values during the prolonged dry season (August - F(3,34) = 6.67, p ≤ 0.5; F(3,30) = 5.64, P ≤ 0.5 respectively), while B. verbascifolia evidenced oscillating values during the year, with its highest values at the onsets of the dry (June) and rainy (December) seasons (F(3,105) = 4.73, P ≤ 0.5) (Fig. 7B). Carotenoid contents were higher in B. intermedia and B. verbascifolia at the onset of the dry season (June) (F(3,34) = 3.18, P ≤ 0.5; F(3,105) = 6.27, P ≤ 0.5 respectively), but B. coccolobifolia evidenced oscillating values during the different months, being higher at the onsets of the dry (June) and rainy (December) seasons (F(3,30) = 7.80, P ≤ 0.5) (Fig. 7C). No differences in chlorophyll a/b ratios were observed among the different months (Fig. 7D). Despite the observed changes in photosynthetic pigment contents, few modifications of chlorophyll fluorescence were detected, highlighting the lowest maximum quantum yield (F v /F m ) values for B. intermedia and B. coccolobifolia during the dry season (χ = 17.57, df = 3, P < 0.05) (Table 1).
Table 1 Maximum quantum yields of photosystem II (Fv / Fm) of the three Byrsonima species. Measurements were made at the onset of the dry season (June) and after the prolonged dry season (August), as well as at the onset of the rainy season (December) and after the prolonged rainy season (February), in a savanna environment
Dry season | Rainy season | ||||
The onset of the dry season | Prolonged dry season | The onset of the rainy season | Prolonged rainy season | ||
B. intermedia | 0.733 ± 0.07b | 0.739 ± 0.01b | 0.771 ± 0.07ab | 0.805 ± 0.01a | |
B. coccolobifolia | 0.710 ± 0.08b | 0.721 ± 0.06b | 0.796 ± 0.04ab | 0.804 ± 0.03a | |
B. verbascifolia | 0.795 ± 0.04ab | 0.778 ± 0.01ab | 0.812 ± 0.02a | 0.808 ± 0.03a |
* Lower case letters indicate differences among the different months according to ANOVA, at a 5 % level of probability. Values are reported as the mean ± standard deviation.
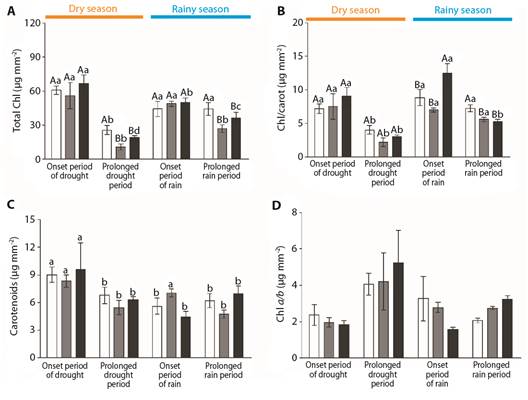
Fig. 7 Chloroplastidic pigment contents of Byrsonima intermedia-white bars, Byrsonima coccolobifolia-gray bars, and Byrsonima verbascifolia-black bars at the onset of the dry season (June) and after the prolonged dry season (August), and at the onset of the rainy season (December) and after the prolonged rainy season (February). A. Total chlorophyll content. B. Chlorophyll/carotenoid content. C. Carotenoid content. D. Chlorophyll a/b ratio. Data are reported here as the mean ± standard error of the mean. Capital letters represent differences among the different species, and lower-case letters represent differences among the different months. The absence of any letter signifies the absence of any difference between the factors.
Discussion
The leaf phenology described for the three Byrsonima species studied here are different from their previously reported patterns (Boas et al., 2013; Kuster et al., 2017; Lenza & Klink, 2006; Silvério & Lenza, 2010), with B. intermedia and B. coccolobifolia classified here as brevideciduous and B. verbascifolia as evergreen. Our data reinforce the view that environmental seasonality may act differently on plant growth and development, changing phenological expression mainly when related to water resource availability during the year (Borchert et al., 2005; Cuzzuol & Clippel, 2009; Morellato et al., 2000; Williams et al., 1997) and to photoperiod differences during the transition from the dry to the rainy season (Rivera et al., 2002; Rossatto, 2013). Byrsonima intermedia, B. coccolobifolia, and B. verbascifolia initiated new leaf sprouting in the transition between the dry to rainy period. The peak of leaf sprouting occurs at the onset of the rainy season and, independent of an induction factor, new leaf production occurred at a time that guaranteed tissues with high productivity during the period of greatest water availability. Additionally, the timing of each leaf phenophase seems to be determinant for whole plant metabolism in Byrsonima species, adjusting them to seasonal resource availability.
The discrepancies found between our phenological data and those reported in the literature indicate the phenological plasticity of Byrsonima species, which may alter their leaf phenology in response to environmental changes within the same ecosystem. Variations in the phenological behaviors of plant species indicate different strategies in response to similar environmental conditions (Camargo et al., 2018). The marked seasonality of water resource availability in the savanna leads to the predominance of brevideciduous species within the plant community (Camargo et al., 2018; Kikusawa, 1991), which, like B. intermedia and B. coccolobifolia in our study, lose their leaves during the dry season as a mechanism of water stress escape by reducing transpiration (Lohbeck et al., 2015; Singh & Kushwaha, 2016). Evergreen species (such as B. verbascifolia in our study) maintain their leaves during the dry season, but generally have other strategies to overcome water stress (such as xylopodia or well-developed deep root systems with greater water absorption efficiency) (Franco et al., 2005; Jackson et al., 1999) that allow them to maintain their leaves throughout the year (Franco et al., 2005).
Evergreen species commonly have higher SLM values than deciduous species, but that was not observed in our study. SLM represents a balance between leaf density and leaf thickness (Witkowski & Lamont, 1991), and may be used to quantify how much matter the plant invests per unit of photosynthetic area (Villar et al., 2013); it can also determine differences in water storage capacities. The similar SLM values among the different Byrsonima species may have influenced RWC. Furthermore, the similar environmental conditions that the three Byrsonima species studied here were subjected to affect their SLM values, as their leaves are more sensitive to the prevailing conditions during leaf expansion (Witkowski & Lamont, 1991), including light, temperature, water scarcity, and nutrients (Meziane & Shipley, 1999; Niinemets, 2001; Poorter et al., 2009; Tholen et al., 2012). Ishida et al. (2010), in their studies of evergreen and deciduous species, showed that differences related to hydraulic conductance and osmotic adjustments in plants are more dependent on the species themselves than on phenological patterns, an observation that seems to fit for Byrsonima species.
The phenophases of evergreen species are less dependent on rainfall (Singh et al., 2006), but can serve as good models for studies of the effects of water seasonality on the dynamics of stomatal opening, and its implications for CO2 assimilation. In general, evergreen species evidence better control of stomatal conductance and have leaf characteristics that reduce transpirational water losses (stomatal hydraulic resistance may represent 30 to 80 % of the total hydraulic resistance of the plant body) (Ishida et al., 2010). The reduction of stomatal conductance, however, directly affects the entry of CO2, and therefore reduces assimilation efficiency (Chaves et al., 2002). The similar maximum quantum yield (F v /F m ) values seen in B. verbascifolia throughout the year suggest greater water use by that evergreen species (Givnish, 2002; Ishida et al., 2010) as well as a sustained photosynthetic rate throughout the year, allowing the old leaves to persist until there is complete nutrient translocation to younger leaves (Jackson, 1978).
The deciduous species studied here showed higher photosynthetic yields during the season of abundant water supply (Table 1), mainly after complete hydric recovery during the prolonged rainy season. Low precipitation is usually responsible for the low photosynthetic yields observed in savanna plants under water deficit conditions (Ronquim et al., 2009; Zhang et al., 2007). A low water potential during the dry season in Copaifera langsdorffii was enough to impose significant restrictions on total photosynthesis (Ronquim et al., 2009). In the present study, however, the three Byrsonima species evidenced strategies that guaranteed the maintenance of the water statuses of their leaves during the dry season, and despite the low F v /F m values observed during the extended dry season in the deciduous species, their photosynthetic yields were not severely affected by water deficits. Medina & Francisco (1994) reported that Godmania macrocarpa (and other plant species) were also able to maintain high photosynthetic rates even with low relative water contents during the dry season.
The concentrations of chloroplastidic pigments in savanna plants may vary between the dry and rainy seasons, as was seen with B. verbascifolia and Solanum lycocarpum respectively (Kuster et al., 2017). The highest concentrations of chloroplastidic pigments were observed in our study at the onset of the dry season (June), although those chlorophyll levels decreased with the advance of the dry season. Our data reinforce that chloroplastidic pigment concentrations are not related solely to light variations, but also vary with other environmental conditions, such as water availability and phenological conditions. Similar results were reported for Polylepis tarapacana and other plant species (González et al., 2007; Inoue, 2010). According to Inoue (2010), increased pigment contents may be related to lower solar incidence during the winter months, although that might not be the case with vegetation formations in tropical regions having open canopies. The high concentrations of chloroplastidic pigments seen in the Byrsonima species studied here appeared to be a strategy to maintain high photosynthetic rates in the remaining leaves. Moreover, the lowest total chlorophyll/carotenoid ratios and the high carotenoid content values encountered at the onset of the dry season indicate that, under water deficit conditions, Byrsonima plants still invest in mechanisms of photoprotection and excess energy dissipation in their photosynthetic systems.
The resumption of plant growth at the end of the dry season/beginning of the rainy season in the three Byrsonima species studied here, help explain the low leaf starch contents observed at the onset of the rainy season. In contrast, at two months into the rainy season the leaves are at their peaks of structural and functional maturity, with high water-soluble polysaccharide contents (including structural carbohydrates such as pectins) and high photosynthetic rates-with the storage of large amounts of starch. Similar observations were made of the leaves of Sinningia aghensis (Cuzzuol & Clippel, 2009) during the rainy season, with higher starch contents after prolonged rains as a consequence of higher photosynthetic yields due to greater water availability and greater synthesis of photoassimilates and carbohydrate storage. The high F v /F m values observed here in the prolonged rainy season demonstrate the maturity of the photosynthetic tissues in the month following leaf sprouting in abundantly available water. The high F v /F m values also point to the absence of severe photoinhibition and suggest the presence of effective mechanisms for excess energy dissipation even during the dry season.
Many studies have reported that savanna tree species are isohydric (maintaining their water contents relatively constant throughout the year and not performing osmotic adjustments), as they have deep root systems and can extract water from the deepest soil layers (Bucci et al., 2005; Bucci et al., 2008; Franco, 1998; Meinzer et al., 1999; Prado et al., 2004). Osmotic adjustment is, however, an acclimatization response and an adaptation that keeps photosynthetic tissues hydrated under water deficit conditions, (and may reflect high concentrations of soluble carbohydrates in mesophyll cells (Chaves et al., 2009; Irigoyen et al., 1992; Vieira et al., 2017), as was observed in the present study. Medina and Francisco (1994) detected osmotic adjustments in Curatella americana and Godmania macrocarpa, two Venezuelan savanna species with contrasting foliar phenologies. The maintenance of leaf conductance at low water potential levels in both species was ensured by reductions of their osmotic potentials related to increases in their soluble sugar concentrations (Medina & Francisco, 1994). Here, only B. coccolobifolia showed low osmotic potential throughout the dry season, evidencing osmotic adjustment as an alternative to the maintenance of their relative leaf water contents. Byrsonima intermedia and B. coccolobifolia, on the other hand, evidenced lower osmotic potential values at the onset of the rainy season, with high total soluble sugar values possibly indicating the mobilization of carbohydrate reserves at the beginning of the flowering period.
Our data showed divergences in the leaf phenology of three Byrsonima species in relation to previous studies, demonstrating the importance of confirming the phenology of plants before undertaking ecophysiological studies. Savanna seasonality did not drastically affect the photosynthetic efficiencies of the Byrsonima species studied here, even during periods of low water availability. The seasonality of their carbohydrate contents evidenced osmotic adjustments in B. verbascifolia that helped conserve its relative water content during the dry season and represented a mobilization of soluble sugars at the beginning of the flowering period. The metabolic differences between the onsets and peaks of the same seasons indicate the high importance of the phenological status of the leaves (or even the whole plant). Thus, differences in the leaf phenology and physiological strategies among the Byrsonima species studied here converged on drought resistance and the seasonality of water resources in the Brazilian savanna.
Ethical statement: the authors declare that they all agree with this publication and made significant contributions; that there is no conflict of interest of any kind; and that we followed all pertinent ethical and legal procedures and requirements. All financial sources are fully and clearly stated in the acknowledgements section. A signed document has been filed in the journal archives.