Introduction
Aquatic macroinvertebrates (thereafter AM) are one of the most diverse communities in lotic ecosystems and contribute significantly to energy flow in the ecosystem because they belong to multiple trophic groups (Cummins, 2008). Additionally, they are important food items for ichthyofauna, especially in the tropical rivers of medium and high elevation (Gutiérrez-Garaviz et al., 2016). Due to their small size, the mobility of the AM is restricted; and thus, the presence or dominance of some genera in rivers can reflect local conditions of water chemical quality, which makes them important indicators of ecological quality of the system (Forero et al., 2014; Lozano-Ortiz, 2005; Roldán-Pérez & Ramírez-Restrepo, 2008). Both, the structure of the physical habitat and its temporal variability, can affect the presence and diversity of AM (Ríos-Pulgarín et al., 2016).
Macroinvertebrates are highly dependent on the hydrological regime because the flow of water determines the stability of the riverbed, dispersion processes and the supply of allochthonous organic matter, according to the coverage of the riverbank and runoff processes during the rainy season (Dudgeon, 2011; Rodríguez-Barrios et al., 2011; Wang et al., 2013), but the response to this variability is also modulated by the local habitat (Tomanova & Usseglio-Polatera, 2007). For example, the order of the river and the type of substrate are related to the richness and taxonomic composition (Melo & Froehlich, 2001) and rivers with dense riparian vegetation covers offer greater substrate stability against hydrological disturbances and faster recovery of communities (Longo et al., 2010). Since the habitat of macroinvertebrates is the result of interaction among the river substratum, the hydrology and the surrounding terrestrial ecosystem, under different habitat conditions, there would be different responses (e.g. richness or abundance) of the macroinvertebrate assemblage against hydrological alterations.
Several studies suggest that the structure and dynamics of aquatic communities, particularly AM, respond simultaneously to habitat heterogeneity and temporal variability, as suggested by theoretical models of Habitat Templet or Hydrological Disturbance (Ríos-Pulgarín et al., 2016; Tomanova & Usseglio-Polatera, 2007; Townsend & Hildrew, 1994; Winemiller et al., 2010). This model, representing the effects of habitat and the hydrological cycle on aquatic communities, are applied to the AMs that are recurrently eliminated by the dragging of floods that clear out the riverbed, selectively eliminating the organisms that later recolonize, as expected in rivers whose flow rates vary seasonally. However, it is not clear how communities change in regulated systems, where both connectivity and seasonality of flows are reduced. Hence the interest in evaluating the responses of the AM community to the hydrological variability of Andean rivers, which face an increasing pressure for their regulation for purposes of energy generation. In fact, in these systems important effects of the reduction of seasonality on benthic fauna have been documented, during prolonged conditions of low or high flows, such as those that occur during the El Niño phenomenon, which is highly prevalent in the tropical Andes (Blanco, 2003; García et al., 2012; Longo et al., 2010; Ríos-Pulgarín et al., 2016).
In terms of connectivity, the discontinuity in rivers would divide them into discrete regions (Stanford & Ward, 2001), whose biological structure, should respond differently to seasonality (Terra & Araújo, 2011). This would imply that in regulated systems, habitat conditions would be of greater importance, as it has been documented in other communities such as ichthyofauna (Leite et al., 2015). These changes in connectivity and seasonality can have important effects on the composition and abundance of AM. Thus, elucidating their effect on the AM communities generate information of interest to management models of the Andean tropical basins.
Among the most frequent hydrological alterations in the Andean rivers are the reservoirs for hydroelectric generation, whose construction and operation implies the regulation of the flows of the intervened currents. Upstream of the reservoir, the river flow is maintained but downstream the flow is significantly reduced or increased, by regulation, and the effect of hydrological seasonality may disappear, because the new regime depends mainly on the generation rule, that is, on how much water is required to generate power. Under these regulation conditions, three determining processes for the presence and abundance of aquatic organisms are affected, the connectivity of the system (including flows of nutrients, sediments and organisms, etc.), the seasonal variability (rain / dry) and habitat availability.
Eastern Antioquia is one of the regions with the greatest abundance of water resources in Colombia and generates 30 % of the country’s production in approximately 13 500 ha of reservoirs that regulate numerous rivers (Ríos-Ocampo & Vélez-Gómez, 2015). There is located the Tafetanes-Calderas system, a complex of consecutive reservoirs and diversions that regulates the flow of three rivers. In this scenario, we studied the composition, richness, and abundance of AM, with the aim of evaluating the effect of regulation on their diversity, based on the hypothesis that the river sections after regulation present fewer diversity than non-regulated ones, due to a potential loss of seasonality of the system. In this scenario, we studied the composition, richness, and abundance of AM, with the aim of evaluating the effect of regulation on their diversity, based on the hypothesis that, due to a potential loss of seasonality of the system, firstly, the sites after regulation are expected to have less values of alpha diversity (Q0, Q1 and Q2 values). Secondly, groups of AM exhibit different tolerance to water quality (Ephemeroptera, Plecoptera, Trichoptera, hereafter EPT, other arthropods and non-arthropods), should display different patterns of affectation. Finally, because of the regulation, the ENSO phenomena should have less effects in the sites downstream of the dams.
Materials and methods
Study area: The Tafetanes-Calderas system is a complex of consecutive reservoirs and diversions that regulates three rivers located in the East of the department of Antioquia-Colombia (Fig. 1), western of the Magdalena basin, recognized as the world’s most diverse diversity hotspot (International Conservation, 2020). The region includes pre-mountain and low mountain rain forest life zones (Holdridge, 1967), with rainfall between 3 850 and 5 552 mm/year and an average annual temperature of 18 °C (IDEAM, 2020a). The sampling sites were located consecutively before and after each reservoir as follows: S1 and S2 correspond to the Tafetanes river sites before (1 780 masl) and after the Tafetanes reservoir (1 740 masl), S3 and S4 to the Calderas river sites before (1 340 masl) and after the Calderas reservoir (1 324 masl), and S5 and S6 to La Arenosa creek sites before (1 142 masl) and after the discharge of turbine waters from the Calderas Reservoir (1 097 masl) (Fig. 1).
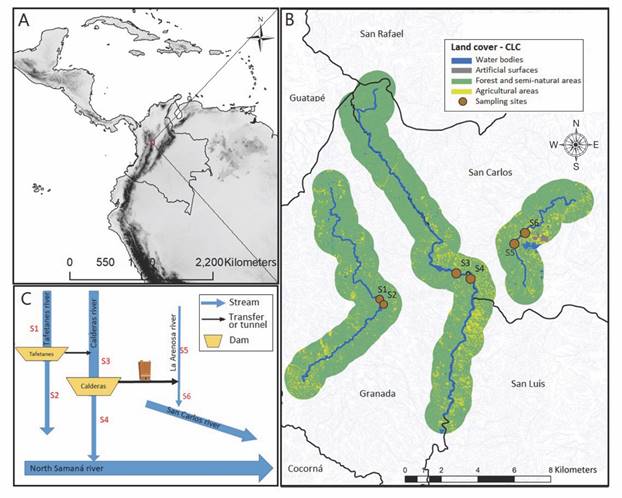
Fig. 1 Study area. A. location; B. flow diagram, reservoirs and drains of the Calderas system; C. location of sampling sites and coverage map of the Calderas, Tafetanes and La Arenosa rivers.
The ArcGIS 10.7.1 (ESRI, 2019) software was used to establish the vegetation covers; a SENTINEL 2019 satellite image, with resolution 1:10 000 of the study area, which was processed in four bands and later classified with five cover types: forests and semi-natural areas, agricultural territories, clouds, water, and wet areas and artificial areas, using a buffer area of 1 km both sides along each riverside. The riverbank in the three rivers present mainly semi-natural forest cover, with percentages above 80 %. The Calderas and Tafetanes rivers have a greater slope, depth and size of rocks in the substratum than La Arenosa, but this last present better conservation in the water catchment area.
Sampling design: Each river was sampled four times a year for three years, both sites by river, up and downstream the regulation (six sites), in different moments of the hydrological cycle: rain, drought and transition (waters rising or falling). In order to evaluate the environmental variability, in each period and sampling site, the in situ values of dissolved oxygen (DO), conductivity, pH, and water temperature were recorded at 9:00 h, just before AM collection, using Hach brand cells. Additionally, water samples were taken for turbidity, total dissolved solids (TDS) and total suspended solids (TSS) in Analtec laboratories. All in situ measurements and in the laboratory were made according to the criteria of the Standard Methods (APHA, 2017). The sampling periods were categorized in relation to El Niño phenomenon according to NOAA (2020) and IDEAM (2020b), considering each period as Niño, Niña or Neutral (without alteration of the ENSO/ El Niño phenomenon).
Biological sampling: For the collection of AM, a screen network (kicking net) was used in the center of the river and a 405 cm2 D-net net with 500 µm mesh on the banks. For every site sampling was performed in a transect of 100 m x 1 m and was standardized by time of 30 minutes. Likewise, manual collection of AM was made in all possible habitats such as vegetation, submerged wood remains, rocks, deposition areas, among others; for which 10 substrata were randomly selected at each site, according to the methodology described by Roldán (1996) and Roldán-Pérez and Ramírez-Restrepo (2008). The obtained specimens were packed in plastic bags with 96 % ethanol and transported to the Limnology Laboratory of the “Universidad Católica de Oriente” for subsequent identification. The samples were identified at maximum taxonomic resolution using specialized taxonomic keys (Archangelsky et al., 2009; Aristizábal, 2002; Bedoya & Roldán, 1984; Manzo & Archangelsky, 2008; Manzo, 2005; Merritt et al., 2008; Posada-García & Roldán-Pérez, 2003; Roldán, 1996; Spangler & Santiago-Fragoso, 1992). All the specimens were identified at the genus level, except for an unidentified morphospecies of the sub-family Orthocladiinae, and another of the family Chironomidae (hereinafter Chironomidae mf1). The identified specimens were cured and deposited in the Aquatic Macroinvertebrate Collection of the Limnology Laboratory of the “Universidad Católica de Oriente” (CAM-UCO).
Data analysis: The variability of the physicochemical and biological data at each of the sites was visually inspected using box and whisker graphs. To reduce the numbers of physical-chemical variables was performed a Principal Component Analysis (PCA) based on a correlation matrix, with 100 Bootstrap pseudo-copies. Then, those variables were used to explore their effects on the AM diversity.
To evaluate the diversity of the AM community, the following aspects were considered: the taxon composition, abundance, and diversity aspects; based on the number of effective species Q0, Q1 and Q2 (Moreno et al., 2011), respectably, for each site and sampling period. Thus, the diversity Q of order zero (Q0) refers to the species richness, regardless of their relative abundance; the diversity of order one (Q1) considers the relative abundance of each of the species in the community; finally, diversity of order two (Q2), refers to the diversity of the most abundant or dominant species (common species) (Jost, 2006).
To establish the differences in the taxonomic composition between samples, cluster analysis was used, where the composition by order was considered an attribute of the sample and the groups were identified by calorography. The similarity matrix was obtained with the Bry-Curtis index, using the R-Wizard software (Guisande et al., 2014). This similarity analysis was also performed at the minimum taxonomic level, to corroborate the results.
To establish the differences in taxon composition among samples, percentage similarity measures were used, where the taxon composition was considered an attribute of the sample, and the clusters were identified using clusters graphs. The similarity matrix was obtained with the Bry-Curtis index, using PAST v 4.11 (Hammer et al., 2001).
To identify spatial (sampling sites, regulation condition) and temporal patterns (ENSO phenomenon) in the composition of the AM community in relation to environmental variables, Canonical Discriminant Analysis (DCA) was conducted. The analysis included the biological variables: total abundance, diversity (Q0, Q1, Q2) and abundance of the groups: non-arthropods (genera of the classes Gastropoda, Bivalvia, Clitellata and Rhabditophora), ETP (Ephemeroptera, Plecoptera, Trichoptera), Diptera (12 genera) and other arthropods (genera of the orders Trombidiforme, Hemiptera, Lepidoptera, Megaloptera, Coleoptera, Odonata and Decapoda). This grouping represents species according to their tolerance and bioindication of environmental quality, considering that ETP genera are indicators of good water quality, non-arthropods and Diptera are indicators of poor quality, and other arthropods include groups with variable tolerance, but in intermediate ranges. The contributions of the different biological groups and environmental variables in the discriminant analyzes by factor were represented graphically as vectors whose length is proportional to the contribution of each variable to the explanation of the variance. Subsequently, a non-metric multidimensional scaling (NMDS) was performed using a matrix of distances, considering the species (morphospecies), based on the Bray-Curtis index in PAST v 4.11 (Hammer et al., 2001). The stress value reflects how well the ordination summarizes the observed distances among the samples. To reduce the stress, a three-dimensional (3D) ordination space was performed of which two axes were shown. Stress values below 0.15 were considered a good indicator of configuration or fit (Kruskal, 1964). Also, an analysis of similarities (ANOSIM) was used to test the overall AM communities for significant differences in taxon composition between sampling sites (Bray-Curtis similarity, 9 999 permutations, pairwise comparison by Bonferroni corrected P-values).
Finally, to evaluate the effect of environmental conditions (temperature and conductivity) and regulation factors and ENSO on biological variables, the following generalized linear model (GLM) was used, where;
(1)
Biological variables (Abundance, Q0, Q1 and Q2 index), were used as response or exogenous variables yjklmn are the biological variables (Abundance, Q0, Q1 and Q2 index), and regulation (Regulated, unregulated) and ENSO were used as endogenous variables or factors. Temperature and electrical conductivity were integrated as covariates to the GLM; In addition, the error associated with each measurement was taken as random. b0 is the intercept, aj is the effect of the j-th regulation factor (Regulated, unregulated), Jk is the effect of the k-th ENSO, b1 is the linear regressor for the covariate temperature (T), b2 is the linear regressor for the covariate conductivity (C). eijklmn, is the random error associated with each measurement.
Seven generalized linear models were compared with different probability families (Gaussian, Poisson and Gamma) and with different link functions; this, to identify the model that best fit the type of data analyzed. The best models were selected regarding the lowest value of the Akaike (AIC) and Bayesian (BIC) information criterion and in relation to the real behavior of the variable in the field. Only factors with a significant effect perceived in previous analyzes were included in the GLM analysis, this in order not to include bias in the final model. These analyzes were carried out using the specialized R-project software, version 3.6.3 (R Core Team, 2020) and R-Wizard 4.1 software (Guisande et al., 2014).
Results
Environmental variability: The parameters measured in the field, such as water temperature, pH and dissolved oxygen, presented little spatial or temporal variability, with temperature averages of 18.4 °C for Tafetanes, 19.6 °C for Calderas, and 21.7 °C for La Arenosa; while the dissolved oxygen averages for the three sources were between 7.5 and 7.7 mg/l; and the pH average was kept in neutral ranges, with averages between 7.3 and 7.4 pH units. The variability of electrical conductivity was between 11.1 µS/cm and 54 µS/cm. Other parameters were analyzed in the laboratory (Total Suspended Solids TSS, Total Dissolved Solids TDS, Total Solids TS and Chemical Oxygen Demand COD), equally low values were found for the three rivers. In the case of TSS, averages of 41.4 mg/l were found in Tafetanes, 13.5 mg/l in Calderas, and 10.7 mg/l in La Arenosa; the average TDS concentrations for the three rivers were very similar with values between 38.1 mg/l and 44.1 mg/l. The organic matter that could be oxidized, biodegradable or not, represented in the COD, presented averages concentrations of 25.8 mg/l in Tafetanes, 22.04 mg/l in Calderas, and 21.9 mg/l in La Arenosa. The measured parameters showed low concentrations, typical of surface sources anthropically little intervened and with good physicochemical water quality according to Chapman. After the PCA, just five variables were selected for the NMDS (data not shown). The variability of both physicochemical and biological parameters is presented in Fig. 2.
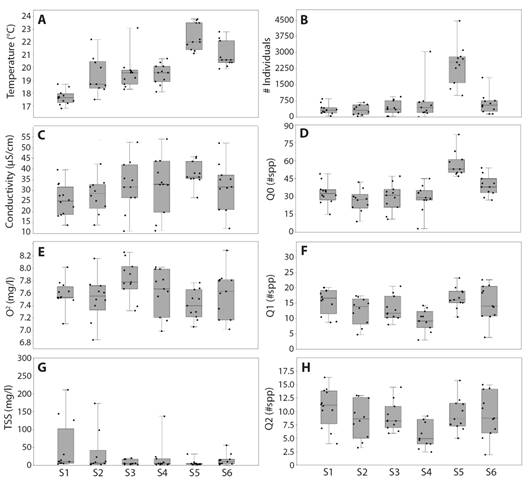
Fig. 2 Box plot of variability by sites at the Calderas system. Environmental variables on the left column and biological variables on the right column. Whiskers represents the range of the values for each variable. A. Water temperature. B. Abundance. C. Conductivity. D. Diversity order 0 (species richness). E. Dissolved oxygen. F. Diversity order 1. G. Total suspended solids. H. Diversity order 2.
Structure of macroinvertebrates community: In total, 53 214 individuals from 165 taxa were collected, and most of them were from the orders Ephemeroptera (23.8 %), Trichoptera (23 %), Coleoptera (21.76 %) and Diptera (18.47 %), totaling about 90 % of catches. These orders were important at all sites and sampling periods, but their abundances were higher at non-regulated sites, especially at site 5 (Fig. 2B). At the family level, Elmidae, Baetidae Leptoceridae, Leptohyphidae, and Chironomidae were the most abundant at all sampling sites and times, and they predominated at site S5.
The species composition showed that an unidentified taxon of the Orthocladiinae subfamily, and another of the Chironomidae family (hereinafter Chironomidae mf1), as well as Macrelmis mf, Nectopsyche mf., Simulium mf., Leptohyphes mf., Smicridea mf., Anacroneuria mf., Rhagovelia mf., Chimarra mf., Camelobaetidius mf., Baetodes mf., Thraulodes mf., Grumichella mf., were the most abundant and frequent at the different sites and years of sampling. However, the similarity analysis showed differences between regulated and unregulated sites for both, taxa and order levels. In addition, it denoted substantial differences in the taxonomic composition in the regulated sites during the ENSO El Niño period. The figure that best represents the pattern is shown (Fig. 3). The orders that best represent the difference in composition between ENSo and regulation conditions were Trichoptera, Diptera, Coleptera, and Ephemeroptera.
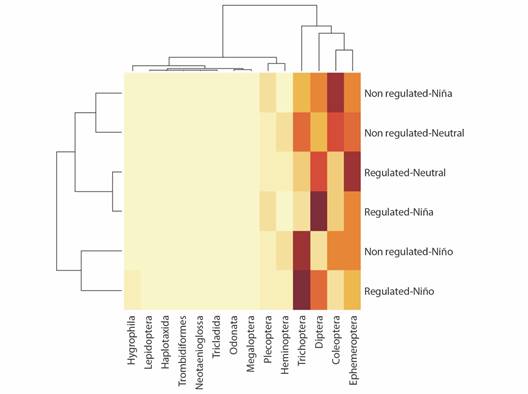
Fig. 3 Similarity analysis in the composition of aquatic macroinvertebrate species among sites and sampling times (ENSO phenomena) at the Calderas system.
Spatial and temporal patterns: The discriminant analysis among sampling sites, intervention states, and El Niño phenomena on the environmental and biological variables obtained between 65 % and 75 % of cases correctly identified through cross-validation; and it explained between 70 % and 100 % of the variance observed in the first canonical axis (Fig. 4).
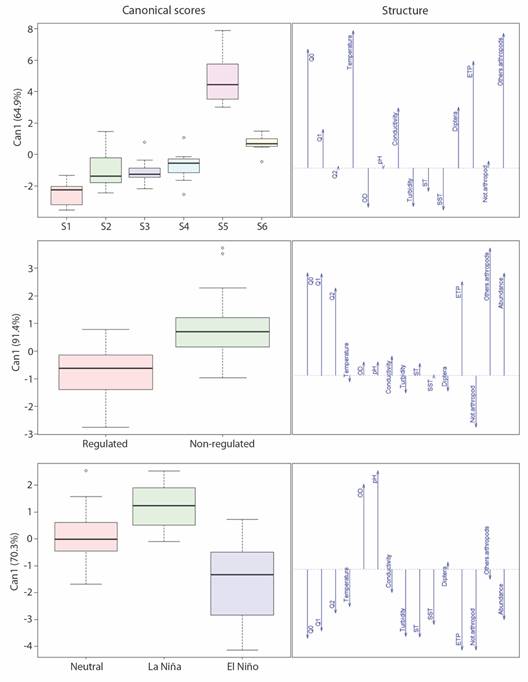
Fig. 4 Discriminant analysis of the sampling sites, regulation condition, and ENSO phenomena, based on environmental variables, abundance, richness, and composition of aquatic macroinvertebrate species at the Calderas system. Whiskers represents the range of the values for each variable.
A gradient was observed at the sampling sites with clear differentiation from the S5 site, based on greater abundance, species richness (Q0) and the presence of ETP organisms, and other arthropods; accompanied by a notable difference in water temperature, conductivity and dissolved solids. The regulated sites were distinguished by a greater presence of Diptera and non-arthropod organisms, accompanied by temperature difference and low concentrations in TSS; and consequently, low turbidity. On the other hand, non-regulated sites were characterized by higher values in most of the environmental and biological variables. The El Niño phenomenon was differentiated by the abundance of ETP and non-arthropods organisms. The former dominating in the nonregulated sites and the latter in the regulated sites, while the La Niña phenomenon was characterized by the greater presence of Diptera and other arthropods. The NMDS ordination (stress = 0.14, r2 = 0.53) separated the samples from S5, while a mixture of samples from other sites was observed (Fig. 5). It was supported by the significant difference detected among sites (ANOSIM, R = 0.3935, P = 0.0001), in which pairwise comparison suggested differences of S5 with every other sampling site, including the site S6 on the same river (La Arenosa) (P = 0.0015). Every other comparison between sites at the same river was not significant (P > 0.05).
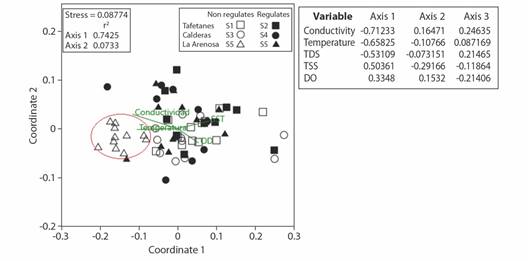
Fig. 5 Non-metric multidimensional (NMDS) ordination (stress = 0.014, r2 = 0.53) for the total set of sampling site of aquatic macroinvertebrates together with the environmental variables, temperature, suspended solids (TSS), dissolved solids (TDS) and dissolved oxygen (DO) at the Calderas system. Matrix data determined by Bray Curtis distances (9 999 permutations) of species abundances. Empty and fill symbols denote sampling sites before and after regulation respectively.
Effects of regulation on diversity: The model that best fit the abundance variable was the model with Gamma distribution and logarithmic link function; the values for this model of AIC and BIC were 949.09 and 964.307, respectively. By the Q0 index, the model with the lowest AIC and BIC values (474.5 and 489.722, respectively) was the one that adjusted to a Gaussian distribution with an identity link function. Otherwise, for the Q1 (AIC = 378.38, BIC = 393.596) and Q2 (AIC = 353.14, BIC = 368.362) indices, the best models were those that fit a Gaussian probability distribution with an inverse link function. The estimates and their respective standard errors are summarized in table 1.
Table 1 Estimates for biological and physico-chemical variables measured in AM and their relationship to environmental factors in the rivers of the Tafetanes-Calderas system.
Biological variables | Physicochemical variables | |||||
Abundance of AM | Dissolved oxygen | |||||
Estimate | Error Standard | P-value | Estimate | Error Standard | P-value | |
Intercept | 632.93 | 498.07 | 0.21 | 7.51 | 0.25 | < 0.01 |
Drought season | -426.67 | 325.24 | 0.19 | 0.15 | 0.16 | 0.35 |
Transition season | -1 497.50 | 622.78 | < 0.05 | 0.88 | 0.31 | < 0.01 |
2017 | - | - | N.A | 0.28 | 0.12 | < 0.05 |
2018 | - | - | N.A | -0.34 | 0.09 | < 0.01 |
Niña | 1 334.15 | 745.96 | 0.08 | - | - | N.A |
Niño | 1 229.82 | 523.80 | < 0.05 | - | - | N.A |
site 2 | -67.40 | 199.73 | 0.74 | -0.05 | 0.09 | 0.62 |
site 3 | 58.55 | 192.59 | 0.76 | 0.24 | 0.09 | < 0.05 |
site 4 | 271.46 | 192.59 | 0.16 | 0.04 | 0.09 | 0.69 |
site 5 | 2 081.18 | 192.59 | < 0.01 | -0.16 | 0.09 | 0.09 |
site 6 | 243.73 | 192.59 | 0.21 | 0.01 | 0.09 | 0.92 |
Q0 | TEMPERATURE | |||||
Intercept | 40.45 | 8.61 | < 0.01 | 17.30 | 1.08 | < 0.01 |
site 2 | -6.05 | 3.45 | 0.09 | 1.69 | 0.42 | < 0.01 |
site 3 | -3.29 | 3.33 | 0.33 | 2.02 | 0.42 | < 0.01 |
site 4 | -3.66 | 3.33 | 0.28 | 1.95 | 0.42 | < 0.01 |
site 5 | 24.98 | 3.33 | < 0.01 | 4.81 | 0.42 | < 0.01 |
site 6 | 6.52 | 3.33 | 0.06 | 3.38 | 0.42 | < 0.01 |
Q1 | CONDUCTIVITY | |||||
Intercept | 19.43 | 4.54 | < 0.01 | 18.50 | 9.28 | < 0.05 |
site 2 | -2.89 | 1.82 | 0.12 | 2.32 | 3.61 | 0.52 |
site 3 | -2.37 | 1.75 | 0.18 | 6.90 | 3.61 | 0.06 |
site 4 | -6.10 | 1.75 | < 0.01 | 7.14 | 3.61 | < 0.05 |
site 5 | 1.14 | 1.75 | 0.52 | 12.51 | 3.61 | < 0.01 |
site 6 | -0.52 | 1.75 | 0.77 | 6.50 | 3.61 | 0.08 |
Q2 | TSS | |||||
Intercept | 14.44 | 3.72 | < 0.01 | -19.89 | 42.74 | 0.64 |
2017 | - | - | N.S | 50.39 | 21.06 | 0.02 |
2018 | - | - | N.S | 18.07 | 15.92 | 0.26 |
site 2 | -2.02 | 1.49 | 0.18 | -20.45 | 16.63 | 0.22 |
site 3 | -1.68 | 1.44 | 0.25 | -43.76 | 16.63 | < 0.01 |
site 4 | -4.94 | 1.44 | < 0.01 | -32.35 | 16.63 | < 0.05 |
site 5 | -1.23 | 1.44 | 0.39 | -45.14 | 16.63 | < 0.01 |
site 6 | -1.35 | 1.44 | 0.35 | -36.71 | 16.63 | < 0.05 |
Regarding the model that is best adjusted to the AM abundance variable, it can be observed that there is a significant (P = 0.0278) and negative effect of the regulation on the abundance of AM. A significant and positive effect of temperature (0.27365) and conductivity (0.03469) on this variable was also identified, which means that as temperature and conductivity increase, the abundance of AM also increases (Table 1).
For the Q0 variable, a negative (-7.906) and highly significant (P = 0.000627) effect of regulation was also found; suggesting that regulation diminishes the richness of AM. For this variable, as in abundance, very similar effects were also found for temperature (Estimate 3.989, P = 0.00000337) and conductivity (Estimate 0.3451, P = 0.019788). Also, contrasting effects were observed between La Niña phenomenon (Estimate -0.295, P = 0.92179) and El Niño phenomenon (Estimate 5.923, P = 0.049414) on the richness of AM (Q0).
Regarding variables Q1 and Q2, only effects of regulation were observed (P < 0.05); For the other factors (site, ENSO), no effects were identified.
Discussion
Human intervention on rivers for hydroelectric generation, by dams and reservoirs, suppose a degradation of river ecosystems by interrupting the natural flow of water, with consequent reductions in the diversity of aquatic biota (Anderson et al., 2018; Bredenhand & Samways, 2009; Lees et al., 2016). However, many of the responses of the macroinvertebrate community of the tropical Andean rivers to the regulation are still unknown. Therefore, this work evaluated whether the regulation affects the diversity of AM, as a consequence of the potential decrease in environmental variability in a system of three regulated rivers in the Colombian Andes (Calderas system).
According to the initial hypothesis, a reduction in seasonality of regulated rivers was observed, which was reflected in little temporal environmental variability. Under these conditions, the results suggest that differences in AM diversity, particularly richness and abundance, were related to both sampling site and effect of regulation on rivers. The three regulated systems presented lower diversities downstream to the reservoirs, regardless of the hydrological moment or the climatic phenomenon (ENSO). Significant negative effects of regulation were found on abundance, total richness (Q0), of common species (Q1) or of dominant species (Q2), as has been documented in regulated rivers in temperate zones (Martínez et al., 2020), but unlike these, in the Calderas system there is no seasonal component in the diversity response. This reduction in seasonality contributes to explain the negative effect of regulation, since the presence of dams and diversions reduces flow peaks and creates uniform and not very dynamic habitats, which concentrate organic matter and favor the more tolerant species.
The reduction of post-reservoir AM richness has been documented in some empirical studies (Mesa, 2010; Milner et al., 2019), and others summarized in the review by Wu et al. (2019), in which an increase in the abundance of pollution-tolerant groups, and the reduction of less tolerant groups such as ETPs, are reported. However, natural interruptions in the course, such as waterfalls up to 70 meters high, seem to have less effect on the reduction of ETP communities, as documented in rivers in the Cerrado of Brazil (Andrade et al., 2020), confirming that the decrease in seasonality has an equal or greater effect on the composition and abundance of tropical Andean macroinvertebrates than the loss of connectivity.
On the other hand, even though the effects on the flows associated with the ENSO / El Niño events have been documented in this region of the Colombian Andes (Poveda et al., 2001), there were no significant effects of the ENSO on the abundance of AM. Nevertheless, both the dry (El Niño) and the rainy (La Niña) ENSO periods were related to the lowest abundances, in relation to the neutral years; except in sites S2 and S4 where the dry bed condition maintains low levels, regardless of the hydrological period, ratifying the reduction of the effect of hydrological variability in regulated systems. At these sites, they only presented differences at moments of maximum precipitation, when runoff or the discharge of the reservoirs increase the water level. In this sense, the response of the AM to the ENSO hydrological phenomena will depend on the conditions of the local habitat and the interaction with anthropic regulation. A positive effect of El Niño on Richness (Q0) was also observed in Calderas system, as has been documented in other studies, in response to the decrease in drag and the increase in habitat heterogeneity (Blanco, 2003; Longo et al., 2010; Ríos-Pulgarín et al., 2016; Rodríguez-Barrios et al., 2011), however, the significance of this effect is restricted to unregulated sites. This suggests a buffer effect of reservoirs on hydrological extremes, and consequently a decrease in the impact of ENSO on diversity in regulated systems.
In terms of composition, effects of both regulation and sampling site are also observed. The results of this work showed greater abundances of species of EPT and other arthropods in the sites of non-regulated flow, while the species which are indicators of organic contamination and tolerant to hydrological disturbance (annelids, mollusks, and Diptera Chironomids) predominated in the regulated sections as dry beds. This is probably related to unfavorable conditions in the downstream sites of the regulation (S2, S4 and S6), where the first two are dry beds, in which the permanently reduced current favors puddle formation, modifying the temperature and oxygen concentration. The last site (S6) has the opposite effect since, after regulation, its flow is significantly increased when receiving the turbine waters of the Calderas reservoir. This also implies a reduction in temperature and an increase in organic matter (mainly non-biodegradable), suspended solids and turbidity. Such fluctuations in water level and water quality would affect the AM community as a consequence of the discontinuity in the ecological characteristics (Hurtado et al., 2005).
Simplified communities like these, with an abundance of tolerant taxa, have been documented in degraded environments (Mesa, 2010). However, it is important to say that Chironomids, such as Orthocladiinae, were found in both impacted and conserved rivers, probably due to their species richness and wide range of habitat preferences. The species in this group have been found widely distributed in all types of aquatic habitats in the Andes from 72 to 4 425 masl (Ospina-Torres et al., 2018). This subfamily is phytophile, and is favored by the presence of periphyton, particularly filamentous algae (Cranston, 1995); so that shallow and clear water sites such as S5 or as a dry bed (S4) would offer abundant resources for this group, despite the contrasts in environmental quality.
Likewise, it is important to highlight the differences in composition of the communities (Fig. 4, Fig. 5), where site S5 stands out, not only for its richness and diversity of common species-weighted by its relative abundance (Q1) but for a different composition, with 18 exclusive morphospecies and between 30 and 100 times more abundance of genera such as Hexatoma, Macrelmis, Microcylloepus and Nectopsyche; if it is compared to other sites. These genera are recognized as bioindicators in aquatic ecosystems. For example, Hexatoma (Diptera) is highly related to riparian coverage and is intolerant to pollution (Iñiguez-Armijos et al., 2018). According to Ríos-Touma et al. (2011), Elmids such as Macrelmis prefer highly oxygenated surface waters which guarantee the formation of the plastron (Buss et al., 2002); Microcylloepus are often found under rocks, under the bark of submerged trunks, or in dead leaves and submerged branches (Iñiguez-Armijos et al., 2018); Nectopsyche has a high dependence on riverside vegetation (Ríos-Touma et al., 2011). The aforementioned can be associated with a riverbank in better conditions since site S5 has greater upstream coverage and less deforestation in the river near the canal; and, therefore, it has a greater offer of allochthonous habitat and resources, as it has been reported for other highly forested tropical streams (Iñiguez-Armijos et al., 2018). These results ratify that riparian vegetation along the catchment area is important to the AM community (Martínez et al., 2020; Meißner et al., 2019; Villeneuve et al., 2018). Thus, the conservation status of the vegetation cover and the structure of the habitat, and not only the absence of regulation, seem to favor the diversity of AM in this system. So that, the conservation of the forest adjacent to the riverbed would be a potential buffer for the impacts generated by the regulation; and in turn, a source of recolonization for the downstream-regulated sections (Milner et al., 2019).
In terms of the influence of water quality variables, the temperature was the parameter that showed the greatest variation and also the greatest effect on AM diversity. Since its variation was related to both the regulation and the thermal floor of each river. Temperature effects especially affected the creek La Arenosa (1 100 masl) after receiving the turbine waters that enter from the Calderas reservoir (1 340 masl), between sites S5 and S6, where the reduction of between 1.4 and 4 °C in temperature, added to the increase in flows, substantially modify the habitat and contribute to the differences found in the taxonomic composition, abundance, richness, and diversity of species. This effect is a consequence of regulation, that by means of a transfer equates the temperature of the river of a thermal floor that is naturally warm with the temperature of a colder thermal floor. The effect of temperature on diversity is related to the metabolism, growth rate and emergence periods of the different species (Martínez et al., 2020).
Finally, since it is a mountain system with low and medium flows, it is possible that the relative importance of the regulation differs regarding larger rivers. This is a future research field in order to have better elements for the management of regulated systems, incorporating both hydrological parameters and aspects of land use and vegetation cover at different scales, more comprehensively reflecting the factors that regulate the ecology of each river at a local and regional scale.
Regulated systems presented lower diversities than non-regulated ones; due to, although there were some temporary changes in environmental variables, the taxonomic composition, abundance, and total richness responded to spatial differences, being greater in non-regulated sites regardless of the hydrological moment. However, the most notable spatial differences are related to the site where the best habitat supply favors greater richness and abundance. Thus, the conservation status of the vegetation cover and the structure of the habitat, not only the absence of regulation, seems to favor the diversity of aquatic macroinvertebrates in this system. In this way, the conservation of the forest adjacent to the riverbed would be a potential source of mitigation of the impacts generated by the regulation.
Ethical statement: the authors declare that they all agree with this publication and made significant contributions; that there is no conflict of interest of any kind; and that we followed all pertinent ethical and legal procedures and requirements. All financial sources are fully and clearly stated in the acknowledgements section. A signed document has been filed in the journal archives.