Introduction
The fish species Hoplias malabaricus, known in Brazil as “Traíra” belongs to the Erythrynidae family with three genera of Characiformes: Erythrinus Scopoli, 1777, Hoplerythrinus Gill, 1896, and Hoplias Gill, 1903 (Nelson, 2006). They are carnivores (piscivores) and are fish widely distributed in the Neotropics, found in all Brazilian states, covering all watersheds in South America. This genus can efficiently adapt to different environmental conditions (Bialetzki et al., 2008). The genus Hoplias comprises two complexes of species: the Hoplias lacerdae group, with nine species: Hoplias brasiliensis, Hoplias aimara, Hoplias patana, Hoplias teres Hoplias intermedius, Hoplias microlepis, Hoplias macrophthalmus, Hoplias australis, and Hoplias curupira; and the Hoplias malabaricus group which, based on cytogenetic and molecular biology studies, has also been considered a species complex (Jacobina et al., 2011; Marques et al., 2013; Santos et al., 2009; Vitorino et al., 2011).
Another freshwater species, abundant and widely consumed by riverside populations, is the Pygocentrus nattereri, known as “Piranha-caju” or “Piranha-vermelha”. It has a carnivorous food preference from the Serrasalmidae family, a native, non-endemic, and epicontinental species. They have a reddish color, with a grayish head and back, and some articles even record individuals up to 50 cm. Among the numerous species of Amazonian fish, P. nattereri stands out for its economic interest in extractive fishing, handicraft making, aquarium hobby, and the sale of industrialized products, such as dehydrated piranha soup. The species P. nattereri has the characteristic of fish from the Amazonian floodplains due to its abundance in scientific fish samplings, thus being used as an indicator of the stability of these environments (Freitas & Siqueira-Souza, 2009; Murrieta-Morey & Malta, 2016; Silva et al., 2014; Siqueira-Souza & Freitas, 2004).
According to studies by Thatcher and Brites-Neto (1994), adult nematodes that parasitize fish inhabit the digestive tract or body cavities, while in the larval stage, they infect the muscles. There are four larval stages before the adult and, in the case of parasitic forms of fish, the last three are parasitic (Thatcher & Brites-Neto, 1994). Birds, fish, amphibians, and reptiles are hosts for nematodes of the genus Eustrongylides, with birds being definitive hosts and the others being intermediate or paratenic hosts (Benigno et al., 2012; Moravec & Kohn, 1993). According to Eiras et al. (2016), these helminths have zoonotic potential in Brazil, but no reports have been described in Brazilian territory (Barros et al., 2006; Benigno et al., 2012; Eiras et al., 2018; Fontenelle et al., 2016). Larvae can be in subcutaneous tissues, liver, mesentery, and between muscle fibers (Melo et al., 2016).
Pollution directly interacts with parasitism in complex ways, making it difficult to describe its effects on disease (Lafferty, 1997). This interaction becomes clearer in reviews of fish parasites (Williams & Mackenzie, 2003). Some pollutants are toxins, which can harm both the host and its parasites’ immune systems. Some may even preferentially show higher concentrations in parasite tissues than in hosts (Sures, 2008a). However, observations contrary to this have also been made (Andrews et al., 1988). These possibilities lead to a diverse set of predictions about the effect of toxic pollutants on parasites (Sures et al., 1999).
Specific predictions for some parasites and pollution are possible. Fish parasites provide information about water pollution through both their presence or absence and the parasites’ capacity to accumulate heavy metals in tissues, mainly arsenic, copper, lead, zinc, and cadmium (Sures & Taraschewski, 1995). Intestinal parasites like Proteocephalus percae and Acanthocephalus lucii have this ability; their tissues contain 300 times more of these toxins than the muscles and liver of their host fish.
The principal source of mercury, Hg, contamination for humans is the intake of fish and other aquatic organisms. Hg present in fish is approximately 90 % in the form of methylmercury (MeHg). MeHg is the most toxic chemical form of Hg for living beings (Crespo-Lopez et al., 2021). In the Amazon and mainly in the region surrounding the Tapajós River basin, the presence of MeHg in fish that commonly feeds the population has been shown (Lino et al., 2018). Thus, monitoring MeHg in fish is of fundamental importance for understanding the contamination levels in the environment and humans by this metal.
When ingested, fish can be a relevant vehicle for heavy metal transmission. Some studies (Albuquerque et al., 2021; Alcala-Orozco et al., 2020; da Silva-Costa et al., 2022; Lima et al., 2015; Silva et al., 2019) in fish from Amazonian environments, including in specimens consumed by indigenous communities (Vasconcellos et al., 2021), point to the detection of worrying levels of these metals in species of commercial interest. Human contamination by fish gains importance when considering the preference of Amazonians for this protein source, to the detriment of others (Barreto-Sa et al., 2019; Haddad-Junior et al., 2021; Lino et al., 2018; Lopes et al., 2016). The present study is the pioneer in describing Eustrongylides sp. larvae (Nematoda: Dioctophymatoidea) and the interaction with their fish hosts as indicators of the accumulation of contamination by HgT (Total Mercury) in the Brazilian Amazon. It also describes the distribution of HgT in the internal organs of fish of the species Hoplias malabaricus and Pygocentrus nattereri collected, mainly in oxbow lakes in Rio Tapajós, in the municipality of Santarém, in the state of Pará, which is a place that suffers negative impact of anthropic actions due to gold mining above the Tapajós River, consequently, carrying it to the area where the collections took place.
Materials and methods
Collection Location: Fish were collected by fishing in oxbow lakes of tributaries of the Tapajós River, in the Amazon Basin, near the Alter do Chão district of the municipality of Santarém-Pará, coordinates (2°17’46” S; 54°53’47.74” W - Quantum GIS version 3.14.15, QGIS Development Team, 2020) (Fig. 1). Gillnets measuring 30 m in length and 2.5 m in height, and with a mesh size of 30, 35 and 40 mm between knots were used to capture the fish, along with bait. Collections occurred concurrently to compare data obtained in two fish species (H. malabaricus and P. nattereri) from the same environment (Fig. 2C, Fig. 2D). The collections took place throughout the year 2022. Sixteen specimens of H. malabaricus and 24 specimens of P. nattereri were captured. These specimens were obtained in two hydrological periods: in winter (characterized as high waters), collected in the month of April (six specimens of H. malabaricus and 14 P. nattereri), and in summer (characterized as dry waters), collected in December (ten specimens of H. malabaricus and ten P. nattereri). After collection, fishes were euthanized using clove oil (150 mg/L diluted in water), their total length (Tl, in centimeters) and whole weight (in grams) were measured using (for the collection of the total length, a measuring tape was used and for the weight a scale was used), then fish were dissected at the collection site, and sex determined according to Vazzoler (1996).
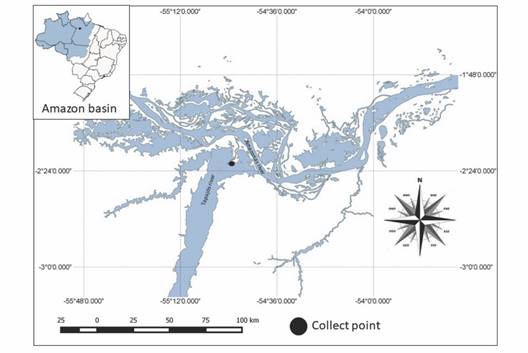
Fig. 1 Map of Brazil, highlighting the Amazon River Basin. Geographic coordinates (2°17’46” S; 54°53’47.74” W - QGIS version 3.14.15, QGIS Development Team, 2020).
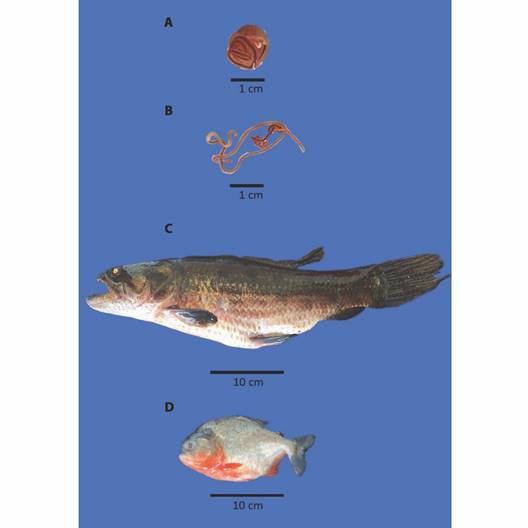
Fig. 2 A. Encapsulated and B. free larvae of Eustrongylides sp.; Host species, C. Hoplias malabaricus and D. Pygocentrus nattereri.
Collection of parasites and organs: After being anesthetized and euthanized, the fish were examined under a stereomicroscope to remove all Eustrongylides sp. larvae encapsulated in musculature (Fig. 2A, Fig. 2B), and these were separated for the morphometric study. For morphological identification, we examined ten specimens of parasites, which were clarified according to Santin et al., (2009) and studied by light microscopy, with magnification from 100X to 400X, according to Moravec and Kohn (1993), after the preparation of temporary slides. The photomicrographs were processed in a Zeiss Axioplan microscope with an Axiocam ERc 5s camera.
The ecological terms used, and the parameters of prevalence, abundance, and mean intensity of infection were calculated according to those proposed by Bush et al. (1997). These parameters were calculated using Quantitative Parasitology 3.0 software (Rozsa et al., 2000), according to Reiczigel et al. (2019).
All Eustrongylides sp. larvae and the following fish organs (head, kidney, heart, liver, spleen, small intestine, gonads, and muscles) were frozen at -20 °C for HgT analysis. Muscle samples were free of this type of parasite.
HgT analysis: Aliquots weighing between 0.01-0.05 g (wet weight) were analyzed for HgT concentration. For such analysis, the Direct Mercury Analyzer - DMA - 80 Tri cell (Milestone - Italy) was used. To ensure the reliability of the results, the certified reference material BCR-463 Tuna Fish (SIGMA) was used, with reference values of 2.85 ± 0.16 mgHg/kg and obtaining values of 2.80 ± 0.07.
Calculation of the bioconcentration factor (BCF): The Hg bioconcentration factor (BCF) evaluates the capacity of bioaccumulation of the metal in larvae of Eustrongylides sp. by comparing the concentration of HgT accumulated in these larvae with that contained in the musculature of infected specimens of H. malabaricus and P. nattereri. The BCF estimate was calculated and analyzed according to (Schludermann et al., 2003; Sures, 2008b; Sures et al., 1999). BCF was calculated using the expressions: BCF = (C (parasite) /C (host tissue) ).
Statistical analysis: Comparative analyzes referring to HgT concentration in Eustrongylides sp. larvae related to that of the fish organs (head kidney, heart, liver, spleen, small intestine, gonads, and muscle), and between oxbow lakes were conducted using Principal Component Analysis (PCA). Spearman’s correlation coefficient was used to test for significant associations between HgT concentrations in larvae and host tissues/organs. All statistical tests were performed using the SPSS 8.0 program (Tanaka & Mori, 1997).
Results
Morphological characterization, based on ten specimens of third instar larvae of Eustrongylides sp.: body of larvae filiform, yellowish, 60.17-126.20 mm long and 0.65-1.39 mm wide. Cuticles distinctly transversely striated. Three cuticles visible in these stage four larvae: external cuticle, from the second instar, middle cuticle from the third instar, and internal cuticle from the fourth instar. Conical cephalic extremity. Oral opening small, oval, surrounded by 12 cephalic papillae arranged in two circles; each circle formed by two lateral, two subdorsal and two subventral papillae; inner circle papillae smaller, with pointed apices. Pair of small lateral amphids like somatic papillae located immediately anterior to the lateral cephalic papillae of the outer circle. Four small somatic sublateral field papillae present between the inner and outer circles of the cephalic papillae. Oral cavity 1.77 mm long; esophageal length 12.53-20.15 mm and width 0.27-0.68 mm. Nerve ring encircling esophagus 23.0-38.2 mm from anterior end. Blunt posterior extremity, with slightly elevated region of the anus. Male larvae: developed caudal sucker with a small cuticular border around the entire perimeter. A wide, thick-walled genital tube (ejaculatory duct) with circular muscles extending anteriorly from the rectum, usually with several coils. Tube curving posteriorly near the esophageal-intestinal junction and, after a short distance, expanding into a large hologonic, blunt-ended testis. Seminal vesicle ends just before the genital tube. Spicule incompletely sclerotized. Female larvae: genital primordium consisting of four regions - thick-walled vagina composed of cuboidal epithelium, wide genital tube, and narrow uterus. The genital tube consists of circular muscles and a thin, narrow lumen, curving posteriorly and expanding into a large, conical-tipped hologonic ovary.
Forty fish were collected altogether - 16 H. malabaricus (eight males and eight females) and 24 P. nattereri (14 males and ten females), with biometric data length/weight of 37.12 ± 9.99 cm / 528 ± 355.90 g, and 17.95 ± 1.64 cm / 167.83 ± 39.72 g, respectively (Fig. 2C, Fig. 2D). The larvae Eustrongylides sp. described in this study are all the third stage (L3). By the absolute numerical distribution of larvae in 27 of all the collected fish (67 %), the infection rates obtained were as follows: prevalence of 63 %, abundance of 1.77, and mean intensity of 2.81 larvae/fish. HgT concentrations found in the tissues/organs of H. malabaricus and P. nattereri and in Eustrongylides sp. larvae in hydrological periods (drought and flood) are shown in Fig. 3 and Fig. 4.
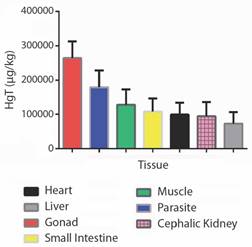
Fig. 3 Total mercury (HgT) concentrations found in the tissues/organs of Hoplias malabaricus and Pygocentrus nattereri and in Eustrongylides sp. larvae during flood and drought.
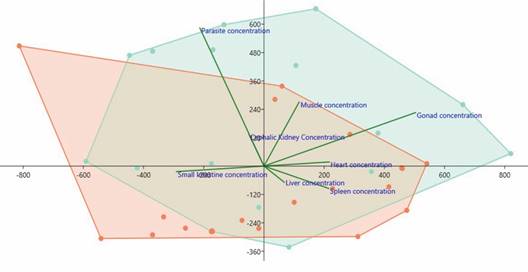
Fig. 4 Total mercury (HgT) concentrations (µg/kg) in the host/parasite system and its characterization by the Principal Component Analysis (PCA). Highlighted in green are the tissue/organ mercury concentrations of the Hoplias malabaricus components and in red are the tissue/organ mercury concentrations of the Pygocentrus nattereri components.
HgT concentrations found in the tissues/organs of the hosts H. malabaricus and P. nattereri and the parasites, Eustrongylides sp. larvae, in the hydrological periods (flood and drought) are presented in Fig. 3 and Fig. 4. The concentrations of HgT were significantly higher (P < 0.01) in the parasite species when compared with that quantified in the tissues/organs of the hosts. PCA showed that the highest concentration of parasites occurred in H. malabaricus, and the highest concentration of Hg occurred in the gonads of this species. The second highest HgT index was detected in the muscles of both fish species investigated here (Fig. 4).
Spearman’s correlation coefficient (r) was crucial to determine the significant relationships between HgT concentrations in Eustrongylides sp. larvae and the muscles of H. malabaricus and P. nattereri parasitized by them. An inversely proportional relationship was observed, demonstrating that the concentration of the metal in these larvae is always higher than that detected in the musculature of these fish (r = -0.076; P ≤ 0.001) (Fig. 5). The HgT BCF in Eustrongylides sp. larvae was higher in H. malabaricus specimens collected in the flood (BCFHg = 15 364).
Discussion
Several studies have discussed the different roles that parasitic fish species play in the aquatic ecosystem. Previously, these organisms were considered only as a threat to the health of fish, but currently they have shown several other functions, such as environmental bioindicators or sentinels of pollution of aquatic environments (Bush et al., 1997; Heckmann et al., 2015; MacKenzie, 1999; Morsy et al., 2015; Poulin, 1992; Santoro et al., 2020; Sures et al., 1999; Williams and Mackenzie, 2003). Recent studies have recorded the capacity of intestinal fish parasites to accumulate high concentrations of heavy metals in their tissues, mainly species of the phylum Acanthocephala (Sures, 2007; Sures & Siddall, 2003; Vidal-Martínez et al., 2010) and to a much lesser extent, the species of Cestoda (Sures et al., 1999) and Nematoda (Morsy et al., 2015; Sures, 2003; Thielen et al., 2004). Currently, the species of Acanthocephala are considered the most efficient bioaccumulators of heavy metals. According to Sures (2006), the performance of species from other groups, such as Nematoda parasites of fish, needs to be better known.
Studies described by Vidal-Martínez et al., (2010) analyzing the average level of lead (Pb) accumulated in the tissues of the nematode Anguillicoloides crassus (Kuwahara, Nimi and Itagaki, 1974), a parasite of the swim bladder of Anguilla anguilla (Linnaeus, 1758), recorded that this was lower than what was found in its host and realized that A. crassus could excrete heavy metals from a proteinase that degrades hemoglobin. Other studies carried out with nematodes reported that the intestinal parasite Raphidascaris acus (Bloch, 1779) accumulated more iron (Fe) and zinc (Zn) (respectively, 68.4 and 86.9 times more) than the liver of its host Esox Lucius ( Linnaeus, 1758) and that the levels of lead (Pb), chromium (Cr) and cadmium (Cd) in the nematode Philometra ovata (Zeder, 1803), an intestinal parasite, were 160, 431 and 119 times higher in the musculature of its host, respectively (MacKenzie, 1999).
Studies focusing on HgT in Amazonian fish have recently been described by Barbosa et al., (2003) and Dórea et al., (2004). Nevertheless, analyses with Hg concentration in parasites, particularly Eustrongylides sp. larvae do not exist in the scientific literature. Nematoda larvae of the Anisakidae family (Railliet and Henry, 1912) bioaccumulated lower concentrations of lead (Pb) and copper (Cu) than the host Dicentrarchus labrax (Linnaeus, 1758) (da Silva-Costa et al., 2022). The present study showed that Eustrongylides sp. larvae were all the third stage (L3) and that their HgT concentrations were higher than in the tissues/organs of the H. malabaricus and P. nattereri hosts. The study also showed that the ability of Eustrongylides sp. to bioconcentrate HgT appears to be related to the site where the host’s infection occurred. However, the Eustrongylides sp. larvae in H. malabaricus have a more evolved spoliation mechanism since they accumulated more HgT when compared to the Eustrongylides sp. larvae of P. nattereri. However, other factors must be involved, such as the development stage and the feeding aspect of the host species.
The present study detected negative correlation for the concentration of HgT, characterizing it as bioaccumulated in Eustrongylides sp. larvae when compared with muscle concentrations in H. malabaricus and P. nattereri hosts. It seems that the time factor has not decisively influenced the concentration of metals in the Eustrongylides sp. larvae in that specific case. This negative correlation observed can be attributed to the kinetics of absorption and the excretion of these metals in this species. Future investigations should reach further elucidation.
Although the Eustrongylides sp. larvae analyzed contained high concentrations of HgT, the reproducibility of individuals within the host were not affected. It may suggest that these parasites have their own detoxification mechanisms. Thus, further investigation and data analysis on free life stages and their exposure to mercury contamination is needed. The results of the present study evidenced HgT concentrations in the muscle and gonads of H. malabaricus and P. nattereri, especially in the latter organ, which had the highest concentration among all the organs analyzed. Regarding the gonads/roe of Amazonian fish, scientific articles rarely mention anything about consumption habits. When this occurs, however, there are no qualitative or quantitative details such as preferred species or ingested quantities. Eating roe is a common practice of the local population, which means a higher transfer of this heavy metal to consumer individuals.
Using parasites as bioaccumulators of heavy metals is more efficient than using their definitive hosts. Sures et al., (1999) report in their study, that the parasitic species reduce the levels of metals in the tissues of their hosts, masking the actual results. The high BCF HgT values found in the present study were similar in both collection periods - flood and drought. The highest values occurred in the dry season, possibly due to the reduction of the flooded area of the lakes and the consequent higher accumulation of metals in the area. The data obtained in the present study indicate that the Eustrongylides sp. larvae have more efficient mechanisms for capturing and accumulating heavy metals than H. malabaricus and P. nattereri. This HgT uptake and bioaccumulation kinetics by Eustrongylides sp. is still not well known, but it was evident that the parasitic species showed higher concentrations of HgT.
Thus, parasitic infections of fish must be considered when using environmental monitoring programs. Otherwise, the levels of pollutants in the hosts may, as investigated by (Sures, 2008b), be erroneously detected and therefore, can invalidate the model. This procedure is essential to use the parasite/host system in ecotoxicological studies. Data in the present work indicate the importance of considering the influence of fish parasite species in biomonitoring programs.
The present work showed the greater bioaccumulative capacity of Eustrongylides sp. compared to its host H. malabaricus and P. nattereri. This feature enabled it to be a potential sentinel organism, which could provide reliable information on pollution or the availability of heavy metals in aquatic environments.
The present study is the first in the Americas that used larvae of a parasitic nematode on fish muscle as bioindicators of HgT accumulation. The results showed the viability of its use, mainly for biomonitoring sensitive aquatic environments in the Brazilian Amazon. Other studies of HgT concentration in fish also recommend verifying that analyzed fish tissue is free of parasites since HgT concentration in the nematodes can be higher than in fish tissue. However, further studies on the kinetics of HgT absorption in Eustrongylides sp. and fish are needed.
Ethical statement: the authors declare that they all agree with this publication and made significant contributions; that there is no conflict of interest of any kind; and that we followed all pertinent ethical and legal procedures and requirements. All financial sources are fully and clearly stated in the acknowledgments section. A signed document has been filed in the journal archives.