Introduction
In aquaculture practices in Guate mala, antibiotics have primarily been uti lized for the therapeutic and prophylactic control of bacterial diseases. Many farm ers believe that administering antibiotic therapy can provide significant advantag es, such as reducing mortality rates result ing from bacterial infections. However, the repeated utilization of antibiotics has resulted in the emergence of drug-resis tant bacteria, such as Aeromonas hydroph ila (García-Pérez et al., 2021).
This bacterium can bioaccumulate in fishery products through the food chain and be consumed by the local community, causing bacteria resistance or harm to hu man health (Rigos et al., 2004; Romero al., 2012; Alanazi et al., 2021). Therefore, to manage drug-resistant bacteria, it is nec essary either to increase drug dosages or de velop a new generation of antibiotics.
To prevent increasing drug dosages, livestock practices must change to treating diseases in fish, or a new alternative must emerge as an additional tool to control both resistant and non-resistant pathogens, as well as a means to reduce antibiotic usage. Medicinal plants can be one such alterna tive (Olusola et al., 2013; Ham et al., 2020
The genus Lippia (Verbenaceae) is among the most used plants in Central and South America for this purpose (Pascual et al., 2001). This genus comprises around
200 species of herbs, shrubs, and small trees
Terblanché & Kornelius, 1996). In Guatemala, Lippia is represented by around 13 species, with L. graveolens, L. alba, L. salamensis, L. chiapasensis, L. dulcis, and L. cardiostegia being the most widely distributed (Standley & Williams, 1970).
Ethnobotanically, L. graveolens is commonly referred to as Mexican oregano. It is an aromatic perennial shrub that can grow up to 2 meters tall, with short-pilose branches and leaves on petioles typically measuring 5 mm-10 mm in length (Standley & Williams, 1970). The shrub is characterized by its axillary capitate spikes inflorescences, which consist of white, sessile, and zygomorphic flowers. These flowers are hermaphrodite and self-compatible (Ocampo-Velázquez et al., 2009).
Lippia graveolens is typically harvested from wild populations growing in a range of ecological conditions, from semi-arid to sub-humid lands. This can result in great morphological variability and chemical polymorphism, with essential oil composition being the most affected component (Tezara et al., 2014). Essential oils are found in all parts of most Lippia genus plants, but leaves or aerial parts are particularly rich in this component, as indicated by Pascual et al. (2001).
In Guatemala, researchers have identified different chemotypes of essential oils, namely carvacrol, thymol, and a minor type known as sesquiterpene (E)-caryophyllene. The latter is sometimes referred to as a mixed chemotype because it contains a variable combination of metabolites (Pérez-Sabino et al., 2012; Salgueiro et al., 2003). However, it is unknown whether the other chemotype with sesquiterpene as the major compound exists in Guatemala. To date, no research has been conducted on the antimicrobial properties of the EO chemotypes originated from wild populations of L. graveolens in Guatemala.
However, L. graveolens from various regions around the world has been investigated as a potential source of bioactive compounds possessing antibacterial and antioxidant properties, as well as for its potential use in preventing fish diseases (Bautista-Hernández et al., 2021; García-Pérez et al., 2019; Leyva-López et al., 2017). Several studies have evaluated the inhibitory effects of L. graveolens against a variety of bacteria, including Gram-positive strains such as Streptococcus agalactiae, Staphylococcus aureus, S. epidermidis, Sarcina lutea, Bacillus subtilis, and Enterococcus faecalis, as well as Gram-negative bacteria like Aeromonas hydrophila, A. sobria, Vibrio cholerae, Escherichia coli, Pantoea agglomerans, and Enterobacter aerogenes (Arana-Sánchez et al., 2010; Castellanos-Hernández et al., 2020; Hernández et al., 2009; Martínez et al., 2021).
Therefore, this study aimed to assess the antimicrobial properties of diverse chemotypes of L. graveolens grown wild in Guatemala, both individually and in combination, against the predominant bacterium in finfish aquaculture in Guatemala.
Methodology
Plant Collection
Samples of a wild population of Lippia graveolens (Verbenaceae) in Guatemala were collected from the following locations: I. El Subinal, Guastatoya, El Progreso (N14°51ˈ15 ̎ /W090°08ˈ05 ̎ (440 m.a.s.l)), II. El Carrizal, San Jacinto, Chiquimula (N14°37ˈ18 ̎ /W089°28ˈ58 ̎ (700 m.a.s.l)), and III. Casas de Pinto, Río Hondo, Zacapa (N15°01ˈ25 ̎ / W089°36ˈ35 ̎ (180 m.a.s.l)). Approximately 2-3 kg of raw materials (leaves) were collected between 7 a.m. and 10 a.m. at each site during the same week of sampling. The material was transported to the Aquatic Health Laboratory at San Carlos University for further analysis.
Its taxonomic identification was verified using Flora of Guatemala Part IX (Standley & Williams, 1970). Mr. Max Merida from San Carlos University authenticated the samples, and voucher specimens (USCG 48320, USCG 48321, and USCG 48322) were stored at the Herbarium USCG CECON-USAC.
The plant material was oven-dried at 45 °C for 48 hours, then ground into powder with an electric grinder. Then, the powder was sieved through a 300 µm-500 µm mesh and stored in a sealed bag at room temperature until further analysis.
Essential Oils Extraction and Gas Chromatography-Mass Spectrometry (GC/MS) Analysis to Determine the Chemotype of L. graveolens
A Clevenger-type device was used to extract essential oils (EO) by hydro-distillation (Majolo et al., 2018). For each extraction, 100 g of plant powder was added to a 2,000 mL volumetric flask and covered with tap water until submerged. The extraction process lasted 2 hours after the water began boiling, and the collected EO were stored at 4 °C in 10 mL amber glass bottles. This procedure was repeated three times.
The EO yield was calculated using the formula Y (%) = M/MV x 100, where M is the mass of EO obtained (g), and MV represents the amount of dried plant powder used (g). GC/MS analyses were performed using a Shimadzu 2010 Plus system coupled with a Shimadzu QP-2010 Plus selective detector (MSD), equipped with a DB5-MS capillary fused silica column (30 m, 0.25 mm I.D., 0.25 µm film thickness).
The oven temperature was set to increase from 60 ºC to 246 ºC at a rate of 3 ºC/min and then remained at 246 ºC for 20 minutes. He (99.999%) was used as carrier gas with a flow rate of 1.03 mL/min and a split ratio of 1:50.
Mass spectra were taken at 70 eV. The m/z values were recorded in the range of m/z 40-700 Da. EO components were identified by comparing their mass spectra and retention indices to values reported in the literature (Adams, 2007). Relative amounts of components were calculated based on GC peak areas without correction factors.
Antimicrobial Assay
Bacterium Strain and Culture
Aeromonas hydrophila (strain: CEMA150219) was obtained from the bacterial collection of the Centro de Estudios del Mar y Acuicultura, Universidad de San Carlos de Guatemala. This bacterial strain was previously found in sick tilapia (O. niloticus) and has shown resistance to oxytetracycline, the most widely used antibiotic in tilapia farming in Guatemala.
To activate the strain, it was processed according to the National Committee for Clinical Laboratory Standards (NCCLS) standard method M31-T and considering the changes made by Alderman and Smith (2001).
The bacterium was cultured on TSA agar (TSA: Merck®) for 18 hours at 28°C. The temperature was selected according to Noga’s (2010) criteria, which recommend conducting microbiology studies of fish pathogens at the temperature at which the organisms are naturally found, rather than 37 °C, as the standard methodology indicates. This is because tropical or subtropical fish pathogens can exhibit poor growth or fail to grow under such conditions.
Subsequently, 3-5 colonies were identified and placed in 1X phosphate-buffered saline (PBS) until reaching a bacterial concentration of 1-2 x 108 CFU/mL, which was verified with an absorbance of 1.00 at a wavelength of 600 nm, measured using a spectrophotometer.
Disk Diffusion Method
Using the disk diffusion method according to Bauer et al. (1966) and considering the changes made by Mazumder et al. (2020), the study tested the effects of different EO chemotypes from L. graveolens on A. hydrophila. The chemotypes tested were:
a) cis-Dihydro-β-terpineol (I), b) carvacrol (II), c) thymol (III)), as well as their combinations: d) (I:II), e) (I:III), f) (II:III) in a 50:50 ratio (v / v), and g) (I:II:III) in a ratio of 33:33:33 (v/v).
Within 15 minutes of preparation of the inoculum, a 100 µL aliquot of A. hydrophila strain was evenly spread on separate Muller-Hinton agar plates (MHA: Merck®). The aliquot was spread across the agar plate using an L-shaped loop, ensuring that it covered the entire diameter of the Petri dish.
Next, an empty paper disc (BBL: Sensi-Disc®) with a diameter of 6 mm was inoculated with 10 µL of each chemotype alone and in combination, and oxytetracycline discs (40 µg) were used as a positive control. Each EO and combination was tested in triplicate, with one disc placed in each Petri dish.
The plates with discs were incubated at 28 °C for 24 hours under aerobic conditions; after, the inhibition zone diameters (IZD) were measured with electronic calipers with an accuracy of 0.01 mm.
The interpretation of the antibacterial activity of EO was determined according to the criteria established by García-Pérez & Marroquín-Mora (2021), considering a specific plant extract, essential oil, or other natural product is considered to have antibacterial action when it produces IZD equal to or greater than 50% compared to the most used antibiotic. In Guatemala, oxytetracycline is the only antibiotic approved by the Guatemalan Ministry of Agriculture, Livestock and Food for tilapia aquaculture production.
Minimum Inhibitory (MIC) and Minimum Bactericidal Concentrations (MBC)
MIC and MBC determinations were conducted using the broth macrodilution assay according to NCCLS M31-T, with modifications by Alderman and Smith (2001). Two-fold serial dilutions of each essential oil chemotype of L. graveolens were prepared in the Muller-Hinton broth (MHB: Merck®) at concentrations ranging from 11.5 μg/mL to 11,825 μg/mL. Triton X (Sigma-Aldrich®) was used as the EO solubilizer at a concentration of 0.1% (v/v).
The A. hydrophila inoculum was adjusted to a concentration of 1 x 108 CFU/mL in PBS and then transferred into MHB to obtain a bacterial count of 1 x 105 CFU/mL. The tubes were then incubated at 28 °C for 24 hours under aerobic conditions. Finally, an aliquot of 100 µL from each tube was plated on TSA and incubated for 18 hours for bacterial count.
MIC and MBC were established according to Levison’s (2004) criteria. MIC was defined as the minimum concentration of antibiotic that prevents a clear suspension of 105 CFU/mL from becoming turbid after overnight incubation; turbidity generally connotes a 10-fold increase in bacterial density. MBC was determined to have the lowest concentration of antibacterial agent that reduces the viability of the initial bacterial inoculum to ≥ 99%.
Assessing Synergistic Interaction Among Chemotype of L. graveolens
The synergistic interaction among L. graveolens EO chemotypes was determined according to Nikkhah et al. (2017) and Gutierrez et al. (2008) with modifications. Four interactions were tested by combining three EO chemotypes: a) I:II, b) I:III, c) II:III, in a ratio of 50:50 (v/v) and combining the three EO chemotypes d) I:II:III in a ratio of 33:33:33 (v/v).
Once the independent MIC was determined, the fractional inhibitory concentration (FIC) was calculated as follows:
FIC of chemotype cis-Dihydro-β-terpineol (FIC_I) = MIC (cis-Dihydro-β-terpineol(a, b)) in combination/MIC (cis-Dihydro-β-terpineol) alone.
FIC of chemotype carvacrol (FIC_II)
= MIC (carvacrol(a,c)) in combination/ MIC (carvacrol) alone.
FIC of chemotype thymol (FIC_III) = MIC (thymol(b,c)) in combination/MIC (thymol ) alone.
FIC of all chemotypes (FIC_IV) = MIC (all chemotypes(d)) in combination/MIC (each chemotype) alone.
The FIC Index (FICi) was calculated as the sum of each FIC. The results were interpreted as follows: synergistic effect (FICi ≤ 0.5); additive effect (0.5< FICi ≤1); no interactive effect (1< FICi ≤4); antagonistic effect (FICIi > 4) as described by Gutierrez et al., (2008).
Data Analysis
The EO yields and inhibition zone diameters for the disk diffusion assay were statistically analyzed with the nonparametric test of Kruskal Wallis, followed by the Mann-Whitney-Wilcoxon test (p < 0.05). The major compounds of the EO chemotypes from L. graveolens identified (greater than 1%) were used to determine the chemotaxonomy through Principal Component Analysis (PCA). Statistical analysis was conducted using statistical software (R Core Team, 2022).
Analysis and Results
The EO extracted from Lippia graveolens collected in Guatemala showed a yellow-reddish color, with yields significantly differing among departments (p < 0.05): 1.42 ± 0.25 % for El Progreso, 1.64 ± 0.14% for Chiquimula, and 3.77 ± 0.28 % for Zacapa. Therefore, it may be inferred that there is a relationship between EO origin and its total production.
These findings coincided with Martínez-Natarén et al. (2014), who reported that the EO yields of different plants varied according to the edaphoclimatic gradient. Therefore, different odors, colors, and amounts of metabolites may be found.
Concerning the chemotype aroma, a notable difference was observed. The EO chemotypes from Zacapa and Chiquimula had a spicy and intense pungent odor, while El Progreso chemotype had a less intense pungent odor.
Accordingly, based on odor, color, and consistency, it is inferred that the number of phenolic compounds may be higher in the Zacapa and Chiquimula chemotypes than in El Progreso. On the contrary, El Progreso may have a higher content of non-phenolic substances (oxygenated sesquiterpenes). The chromatographic profiles of each chemotype of L. graveolens are shown in Figure 1.
According to Table 1, the content and proportion of chemical constituents of the EO (monoterpenes, sesquiterpenes, phenylpropanoids, and other compounds) varied among departments. The PCA horizontal axis explained 81.4% of the total variance, and the vertical axis explained 18.6% (Figure 2), suggesting the existence of three chemotypes (I: cis-Dihydro-β-terpineol: El Progreso, II. Carvacrol: Chiquimula, and III. Thymol: Zacapa) characterized by their different content of chemical metabolites.
In general, twenty-seven compounds were identified in the EO of L. graveolens (Table 1), accounting for 81.84%, 88.72%, and 93.65% of the total EO composition of chemotypes I, II, and III, respectively. The monoterpenes constituted the main class, with cis-Dihydro-β-terpineol (8.84%) as a major constituent of chemotype I, carvacrol (51.82%) chemotype II, and thymol (79.62%) for chemotype III. Sesquiterpenes were the second major group of compounds found in the EO, with caryophyllene oxide as the major constituent of chemotypes I (7.41%) and III (2.32%), whereas the α-Humulene (3.92%) was the major constituent of chemotype II.
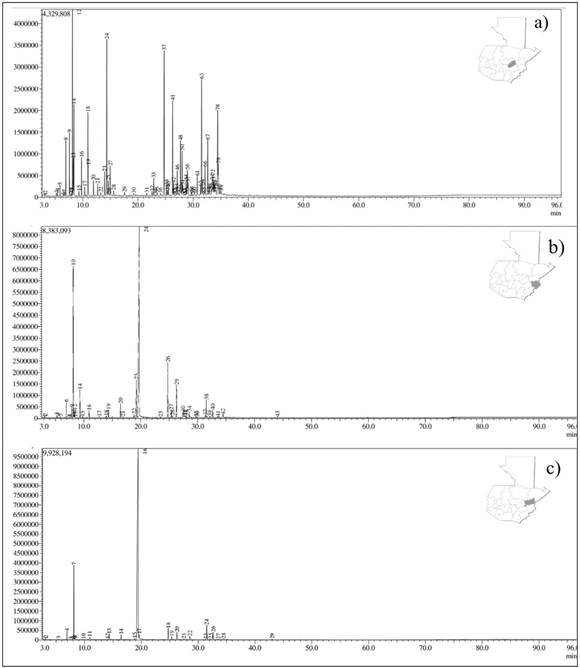
Figure 1 Derived from research. GC chromatogram of essential oils from Lippia graveolens collected from: a) El Progreso (I: cis-Dihydro-β-terpineol), b) Chiquimula (II: Carvacrol) and c) Zacapa (III: Thymol) departments.
Table 1 Chemical composition (%) of the three chemotype essential oils from Lippia graveolens Chemotypes area (%)
No. | Compounds* | Type | RT | RI | I | II | III |
---|---|---|---|---|---|---|---|
1 | Myrcene | M | 6.91 - 6.926 | 988 | 1.82 | 1.13 | - |
2 | p-Cymene | M | 8.12 8.140 | 1022 | 7.22 | 13.94 | 7.76 |
3 | Limonene | M | 8.281 | 1024 | 1.40 | - | - |
4 | 1,8-Cineole | M | 8.390 | 1026 | 3.54 | - | - |
5 | γ-Terpinene | M | 9.306 | 1054 | 2.28 | 2.38 | - |
6 | cis-Sabinene hydrate | M | 9.776 | 1065 | 1.61 | - | - |
7 | cis-β-Terpineol | M | 10.925 | 1140 | 3.66 | - | - |
8 | trans-Sabinene hydrate | M | 10.984 | 1098 | 1.18 | - | - |
9 | Isoborneol | M | 13.944 | 1155 | 1.25 | - | - |
10 | cis-Dihydro-β-terpineol | M | 14.344 | 1156 | 8.84 | - | - |
11 | δ-Terpineol | M | 14.964 | 1162 | 1.66 | - | - |
12 | Carvacrol, methyl ether | M | 16.440 | 1241 | - | 1.45 | - |
13 | Thymol | M | 19.439 | 1289 | - | 5.34 | 79.62 |
14 | Carvacrol | M | 19.750 | 1298 | - | 51.82 | - |
15 | E-Caryophyllene | M | 24.750 | 1417 | 8.74 | 6.53 | 1.65 |
16 | α-Humulene | S | 26.269-26.280 | 1452 | 5.4 | 3.92 | 1.1 |
17 | cis-Muurola-3,5-diene | S | 27.117 | 1448 | 1.43 | - | - |
18 | dehydro-Aromadendrane | O | 27.700 | 1460 | 3.38 | - | - |
19 | b-Chamigrene | S | 27.996 | 1476 | 2.72 | - | - |
20 | δ-Amorphene | S | 28.936 | 1549 | 1.58 | - | - |
21 | E-Nerolidol | M | 30.754 | 1561 | 1.07 | - | - |
22 | Caryophyllene oxide | S | 31.515 - 31.526 | 1582 | 7.41 | 2.21 | 2.32 |
23 | Hinesol | S | 32.140 | 1640 | 1.95 | - | - |
24 | Citronellyl pentanoate | O | 32.636 - 32.640 | 1624 | 3.3 | - | 1.2 |
25 γ-Eudesmol | S | 33.544 | 1630 | 1.76 | - | - | |
26 | α-Eudesmol | S | 34.444 | 1652 | 6.82 | - | - |
27 | epi-α-Cadinol | S | 34.556 | 1638 | 1.82 | - | - |
Total amount of monoterpene (%) | 44.27 | 82.59 | 89.03 | ||||
Total amount of sesquiterpene (%) | 30.89 | 6.13 | 3.42 | ||||
Total amount of other compounds (%) | 6.68 | 0 | 1.2 | ||||
Total amount of volatile compounds different c graveolens (%)hemotype of Lippia | 81.84 | 88.72 | 93.65 |
Note: derived from research. Type M: monoterpene, S: sesquiterpene, O: other compound, RT: retention time, RI: retention index, *Only compounds with a chromatographic area greater than 1% are listed in this table.
Chemotypes I: cis-Dihydro-β-terpineol (El Progre pia graveolens.so), II: carvacrol (Chiquimula), III: thymol (Zacapa) of Lip-
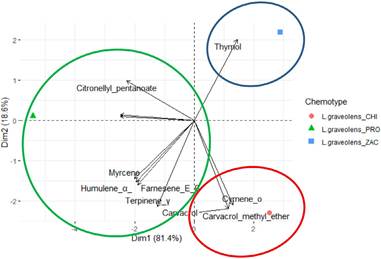
Figure 2 Derived from research. PCA of the 15 major components of the chemotypes among the departments PRO: El Progreso, CHI: Chiquimula and ZAC: Zacapa, of Lippia graveolens.
These results evidenced the EO chem ical polymorphism of this species as men tioned previously for Guatemala (GT) and Mexico (MX): thymol (GT: 67.4%-73.5% / MX: 84.76%-91.77%), carvacrol (GT: 50.3%-51.8% / MX: 72.20%-83.49%), (E)-caryophyllene (GT:10.3%-12.3 % / MX: 0%), and caryophyllene oxide (GT: 2.7%-4.9% / MX: 4.94%-11.34%) (Martínez-Natarén et al., 2011; Pérez-Sabi no et al., 2012; Salgueiro et al., 2003; Senatore & Rigano, 2001).
Pérez-Sabino et al. (2012) classified chemotype I as mixed because its hetero geneous compounds summed no more than 15% of the chemical structure. In this study, chemotype I had cis-Dihydro-β-terpineol (8.84%), E-Caryophyllene (8.74%), and p- Caryophyllene oxide (7.41%) as major constituents of the EO. These proportions differ from those reported previously from EO obtained in the same location. The differences in some compounds could be attributed to the genetic plasticity of L. graveolens, soil composition, climate variability, harvest time, and extraction method, among other factors (Benjemaa et al., 2022; Martínez-Natarén et al., 2014).
The results indicate that three EO chemotypes of L. graveolens provide promising alternatives for controlling the growth of Aeromonas hydrophila isolated from tilapia. Chemotypes III and II were the most effective, presenting IZD ranging from 28.46 mm to 34.03 mm (Table 2). Chemotype I exhibited the lowest IZD (9.95 mm). However, it is important to note that even though the IZD is the lowest, it still indicates an effect based on the criteria established in this study.
Table 2 Antibacterial activity of the three chemotype essential oils from L. graveolens against A. hydrophila
Chemotype combination (Ratio v/v) | Inhibition zone diameter means ± standard deviation (mm) * | MBC μg/mL | MIC μg/mL | FICi |
---|---|---|---|---|
I | 09.95 ± 0.05 e | 2,956.3 | 1,478.1 | - |
II | 28.46 ± 0.45 c | 369.5 | 184.8 | |
III | 34.03 ± 0.30 a | 184.8 | 92.4 | |
I : II (50:50) | 24.82 ± 0.40 d | 739.1 | 369.5 | 2.25 |
I : III (50:50) | 30.34 ± 0.48 b | 369.5 | 184.8 | 2.13 |
II : III (50:50) | 33.43 ± 0.58 a | 184.8 | 92.4 | 1.50 |
I : II : III (33:33:33) | 29.19 ± 0.20 c | 369.5 | 184.8 | 3.13 |
OXI | 10.32 ± 0.52 e |
Note: derived from research. I: cis-Dihydro-β-terpineol (El Progreso), II: carvacrol (Chiquimula), III: thymol (Zacapa), OXI: oxytetracycline (positive control), MIC: Minimum Inhibitory Concentrations, MBC: Minimum bactericidal concentrations, FICi: FICi ≤ 0.5: synergic effect, 0.5 < FICi ≤1: additive effect, 1 < FICi ≤ 4: no interactive effect, FICi > 4: antagonistic effect. * Means in the same column with different superscripts are significantly different (p <0.05).
For the combination of EO chemotypes, the highest IZD (29.19 mm-33.43 mm) was for II:III, followed by I:III and I:II:III. The lowest IZD (24.82 mm) was obtained for the combination of chemotype I:II. The positive control (OXI) had an IZD of 10.32 mm, indicating the resistance of the A. hydrophila to oxytetracycline.
The MIC and MBC values for the three EO chemotypes ranged from 92.4 µg/mL to 2,956.3 µg/mL (Table 2). The lowest MIC and MBC values were found for chemotype III (92.4 µg/mL-184.8 µg/ mL), followed by chemotype II (184.8 µg/ mL-369.5 µg/mL), and finally chemotype I (1,478.1 µg/mL-2,956.3 µg/mL). Although chemotype I obtained the highest MIC and MBC, it still may be considered effective. According to Bussmann et al. (2010), MIC values below 5,000 µg/mL are considered to exert strong antimicrobial activity.
Results indicated that the three EO chemotypes mixture from L. graveolens did not show a synergistic or additive effect. However, the greatest effect was observed when chemotype III was combined with any of the other chemotypes (I and II), even with chemotype I (with the lower inhibition capacity), thus exhibiting an antibacterial effect.
The EO extracted from chemotype III (thymol) exhibited better antibacterial properties than the EO components of chemotypes II and I, with significantly greater inhibition zone diameters and lower concentrations for MBC and MIC. Therefore, it may be inferred that the properties of the thymol metabolite could be responsible for the antibacterial activity observed in this study.
It is well documented that L. graveolens have an antibacterial effect against a broad group of pathogens, especially against Gram-negative bacteria (Arana-Sánchez et al., 2010; Bautista-Hernández et al., 2021; Hernández et al., 2009; Leyva-López et al., 2017; Salgueiro et al., 2003). However, this is the first report analyzing the effect of different EO chemotypes from L. graveolens growing in Guatemala against aquaculture oxytetracycline-resistant bacteria, such as A. hydrophila. It showed that secondary metabolites of L. graveolens may have a direct effect as antimicrobial agents, as stated by several authors for other plant species (Höferl et al., 2020; Koba et al., 2009; Nwanosike et al., 2016).
According to the results, most of the antimicrobial effects of the EO tested may be attributed to the presence and proportions of thymol and carvacrol in the three chemotypes, both acting independently and probably having the same action pathway, since there was no proven synergic or additive effect.
Although the antimicrobial effects of L. graveolens are well established, the mechanism of action is mostly unknown. Based on observations from the study by Lambert et al. (2001), it is inferred that the essential oil of L. graveolens can rupture the external membrane and may induce ATP permeability. This inference stems from the presence of thymol or carvacrol as the main components in L. graveolens. Furthermore, it was found that thymol and carvacrol could disintegrate the outer membrane of bacteria of A. hydrophila, isolated from different sources, and other types of bacteria, such as Escherichia coli and Salmonella typhimurium (Helander et al., 1998).
On the other hand, it is deduced that the components of chemotype I showed lower antibacterial activity due to their relative amount and type of sesquiterpenes, which have weak antimicrobial activity. This weak antimicrobial activity has been attributed to the fact that most sesquiterpenes do not have the appropriate hydrophobicity to break down the bacterial membrane (Bassole et al., 2005).
Conclusions
The results suggest that the essential oil from L. graveolens offers a promising alternative for controlling the growth of Aeromonas hydrophila. Three chemotypes were identified: I: cis-Dihydro-β-terpineol located in El Progreso Department, II: Carvacrol in the Chiquimula Department, and III: Thymol in the Zacapa Department. All EO exhibit antibacterial activities, albeit in different proportions. However, there was no synergistic or additive effect when combining different chemotypes.
The results suggest that the EO chemotype thymol can be used for treating bacterial infections in aquaculture. However, further studies are needed to assess the toxicity of L. graveolens EO on tilapia, as certain EO chemicals may directly impact the target bacteria, and larger amounts could potentially become toxic to fish and other organisms. Furthermore, it is recommended to investigate the in vivo efficacy of EO treatment for Aeromonas infections in finfish production in Guatemala.
Acknowledgments
This research constitutes a chapter of the first author’s doctoral thesis in the program ‘‘Doctorado en Ciencias Naturales para el Desarrollo (DOCINADE), Instituto Tecnológico de Costa Rica’’. It was funded by the Universidad de San Carlos de Guatemala cohort 2017. I want to express my deep appreciation to Carolina Marroquín for the accurate review of this document.
Author contribution statement
All authors declare that they have read and approved the final version of this paper. The contribution percentages for this paper’s conceptualization, preparation, and correction are as follows: J.R.G.P 50%, J.F.P.S 10%, S.E.M.E 10%, A.R.S. 10%, and J.B.U.R. 20%.
Data availability statement
The data supporting the results of this study will be made available by the corresponding author, J.R.G.P., upon reasonable request.
Preprint
A preprint version of this article was deposited at: https://doi.org/10.1101/2023.07.24.549066