Introduction
High altitude rocky complexes represent important centers of plant endemism in South America (i.e., tepuys, campo rupestre); mainly driven by high environmental heterogeneity at small spatial scales (e.g., Barthlott, Mutke, Rafiqpoor, Kier, & Kreft, 2005; Fernandes, 2016). Rocky complexes, known as campo rupestre, are important terrestrial hotspots (Alves Silva, Oliveira, & Medeiros 2014; Silveira et al., 2015), and are found on the high peaks of the main mountain ranges, that mainly occur in the Espinhaço Range mountain in Southeastern Brazil (Giulietti, Pirani, & Harley, 1997; Alves et al., 2014). These singular landscapes occur mainly on quartzite and ironstone formations, mostly above 900 m (a.s.l.) and up to 2 033 m (Benites, Schaefer, Simas, & Santos, 2007; Silveira et al., 2015). They can occur in association with different surrounding vegetation types, such as Cerrado (Neotropical savanna) and Atlantic Forest, as well as in Caatinga, Cerrado and Atlantic Forest transition (central and Northern of the Espinhaço Range). The study of plant communities in these rocky complexes is becoming increasingly relevant since they have been recently proposed as one of the world’s Old Climatically-Buffered Infertile Landscapes (OCBILs), and due to its high local endemism (e.g., Echternacht, Trovó, Oliveira, & Pirani, 2011; Silveira et al., 2015; Pontara, Bueno, Rezende, Oliveira-Filho, Gastauer, & Meira-Neto, 2018).
Campo rupestre is described primarily as a more or less continuous grassy-shrubby vegetation mosaic with scattered rocky outcrops of quartzite that harbor small shrub and sub-shrub patches (Giulietti, Pirani, & Harley, 1997). Patches of transitional vegetation such as cerrado, and relictual montane forests also occur within the campo rupestre landscape, also known as capão florestal (montane forest) and scrub (Alves et al. 2014). Thus, some studies have shown that high environmental heterogeneity (i.e., local topography and soils) determine plant communities assembly forming patches mosaic (Giulietti et al., 1997; Conceição & Giulietti, 2002; Fernandes, 2016). For instance, the vegetation cover at the Espinhaço Range consists of a of mosaic phytophysiognomies, that encompasses i) forest formations associated with water catchments or lowland areas, ii) distinct savannah phytophysiognomies; and iii) grassland formations (Silveira et al., 2015). However, there are still a limited number of studies that evaluate changes in floristic diversity and composition between phytophysiognomies distributed along the same environmental gradient at a local scale.
The high altitude Braziliam rocky complexes comprises the largest areas of campos rupestres, which is the centre of diversity for many angiosperm groups and houses almost 15 % of the Brazilian vascular flora in an area corresponding to only 0.78 % of the country’s land surface (Silveira et al., 2015). For intance, approximately 30 % of plant species of the Espinhaço Range are endemic to campos rupestres (Giulietti et al., 1997). Furthermore, one of the main issues raised when addressing rupestrian vegetation research is its biodiversity, which is not homogeneously distributed, with disproportionately high levels of diversity and endemism at local and regional scales (Fernandes, 2016; Silveira et al., 2015). In this heterogeneous environment the floristic spectra change in the different habitats, where the grasslands had a higher percentage of hemicryptophytes followed by chamaephytes; and the scrub boundaries are more phanerophytic (Caiafa & Silva, 2005; Conceição & Pirani, 2007).
In this context, understanding how the species diversity and species composition patterns of campo rupestre are distributed throughout different phytophysiognomies represents an important challenge for improving the methods of conservation, restoration and management in high altitude rocky complexes. For example, most studies that discuss the premises for campo rupestre conservation are mainly based on grasslands, and we believe that conservation strategies should be planned along the phytophysiognomical gradient, and a first step is describing patterns of diversity. For this reason, we analyzed the changes in the diversity and life forms of the woody-herbaceous community along a phytophysiognomical gradient on the quartzite rocky complexes in the Brazilian Iron Quadrangle. In order to evaluate the ecological patterns of species diversity along the phytophysiognomical gradient, we asked the following research questions: i) How does floristic composition, species diversity and life forms change between phytophysiognomies? ii) Is there some decoupling in the richness and floristic composition between lower and upper strata of montane forest and scrub phytophysiognomies? iii) What are the changes for community dissimilarity between phytophysiognomies in quartzite rocky complexes, and iv) What are the changes for strata dissimilarity of montane forest and scrub phytophysiognomies?
Materials and Methods
Study area: The study was conducted in a quartzite rocky complex area located in a conservation unit (20°10’10.98’’ - 20°10’34.45’’ S & 43°34’07.95’’ - 43°36’50.39’’ W). The area is located in the Eastern boarder of the Iron Quadrangle and encompasses the municipalities of Santa Bárbara and Ouro Preto, Minas Gerais State, Brazil. Climate in the region is (Cwa) according to Köppen classification, subtropical with dry winters and hot summers. Mean annual precipitation is 1 300 mm and mean annual temperature is 20 °C (Silva, 2013).
Three main phytophysiognomies were studied along the pedogeomorphological gradient: i) herbaceous campo rupestre on Typical Dystrophic Regolithic Neosol; ii) scrubs on Umbric Dystrophic Tb Haplic Cambisol; and iii) montane forest patch (capão florestal) on Typical Dystrophic Tb Fluvic Neosol.
Vegetation sampling: Sampling was performed from July 2011 through March 2012. Since the gradient is characterized by the presence of several phytophysiognomies, we stratified the landscape, using plot sizes that were adequate to each predominant life form in each area. In the herbaceous campo rupestre we established eighty plots (1 × 1 m) for abundance sampling. For woody vegetation 20 plots (5 × 5 m) were randomly distributed along the scrub, and 15 plots (10 × 10 m) along the montane forest. In the scrub, all individuals presenting circumference at soil height (CSH) ≥ 3 cm were sampled, while in the montane forest sampling was performed of all individuals presenting circumference at 1.30 m from the soil (CBH) ≥ 10 cm. The number of plots in the montane forest was low because it was restricted in the only patch with limited area within the analyzed gradient. In scrub and montane forest we sampled the upper and lower vegetation strata separately (for more detail see Silva, 2013). Within each plot in the scrub and montane forest we delimited 20 and five plots (1 × 1 m) respectively to sample the lower stratum vegetation (Braun-Blanquet, Lalucat, & de Bolòs, 1979). In each plot, individuals per species were counted and identified to the species level and tagged for measurement. For species and family classification, the Angiosperm Phylogeny Group III system (APG III, 2009) was used.
Life form: The sampled species were classified according to Raunkiaer’s life-form (Raunkiaer, 1934). The results were then graphically illustrated representing biological and vegetational spectra. When the number of individuals of each life-form is counted, instead of species, each class can be weighted by its abundance, resulting in the vegetation’s biological spectrum (Raunkiaer, 1934).
Quantification of biodiversity indices: Measures of taxonomic diversity were calculated for each plot from the three phytophysiognomies. Measures included species richness, Pielou’s evenness and Shannon-Wiener’s diversity index (Magurran, 2004). All diversity indices were calculated using the ‘vegan’ package (Oksanen et al., 2017) in the software R 3.2.2. (R Core Team, 2016).
Data and statistical analyses: Variables were tested for normal distribution with the Shapiro-Wilk test by evaluating the Q-Q plot (Crawley, 2013). To compare the means of the variables between phytophysiognomies (i.e. richness, diversity indices, abundance) we used Kruskal-Wallis’ test (for non-normally distributed data) followed by Dunn’s test (Dinno, 2017). All these analyses were performed with the “stats” and “dunn.test” packages (Dinno, 2017). To verify whether the biological spectra differed among areas, we used the χ2 (chi-square) test (Campos, Villa, Nunes, Schaefer, Porembski, & Neri, 2018).
We analyzed differences in species richness between the three phytophysiognomies by using both sampled-based and individual-based rarefaction and extrapolation curves with the first Hill number (species richness, q = 0) (Chao et al., 2014; Colwell et al., 2012; Jost, 2007). We analyze the difference in individual-based rarefaction and extrapolation curves between vegetation of the lower and upper strata of both montane forest and scrub phytophysiognomies. Extrapolations were made from presence/absence data, being higher than twice the sample size (Colwell et al., 2012). Sample and individual-based rarefaction/extrapolations were computed using the ‘iNEXT’ package (Hsieh, Ma, & Chao, 2016). Rarefaction was estimated as the mean of 100 replicated bootstrapping runs to estimate the 95 % confidence intervals. Whenever the 95 % confidence intervals did not overlap, species numbers differed significantly at P < 0.05 (Colwell et al., 2012).
Non-metric multidimensional scaling (NMDS) was performed to examine differences between the sampled phytophysiognomies in terms of species composition, by assessing Euclidean dissimilarities. We performed the NMDS using the metaMDS function of the ‘vegan’ package (Oksanen et al., 2017). We also performed a permutational multivariate analysis of variance (PERMANOVA, 9 999 permutations) to determine the differences in species composition, by using the ‘adonis’ routine from the ‘vegan’ package (Oksanen et al., 2017). We examined the species composition similarity between phytophysiognomies, such similarity being represented by the mean pairwise similarity between plots in the same phytophysiognomy, and between strata (lower and upper) of montane forest and scrub phytophysiognomies. We furthermore used the abundance-weighted Bray-Curtis dissimilarity index between different phytophysiognomies plots (Villa et al., 2018a). To compare the means of the dissimilarities between strata by phytophysiognomy (i.e., montane forest and scrub) we used Student’s T-Test (Crawley, 2013).
Results
Floristic composition: Overall, 4 446 individuals were sampled, among 218 species, 112 genera, and 55 families. The richest families were: Asteraceae (27 species), Myrtaceae (22 species), Melastomataceae (17 species), Poaceae (13 species), Fabaceae and Rubiaceae (12 species each), Cyperaceae (eight species), Erythroxylaceae (six species), Convolvulaceae, Lauraceae, Malpighiaceae and Verbenaceae (five species each). Altogether, these families represented 62.79 % of the species sampled.
The NMDS showed that species composition varied considerably among phytophysiognomies, mainly among herbaceous campo rupestre with montane forest and scrubs (Permanova, P < 0.01, F2,131 = 3.43; stress = 0.15, Fig. 1). The NMDS ordination of species composition allowed for the distinction of three different groups, with the two principal dimensions explaining most of the variance among plots. On the other hand, we observed that species dissimilarity among campos (Bray-Curtis index ~ 0.30) and scrub (Bray-Curtis index ~ 0.70) phytophysiognomies plots was different. Montane forest presented intermediate values (Bray-Curtis index ~ 0.50) without difference with relation to campos and scrub (Fig. 2A). Species composition dissimilarity presented differences (P < 0.05) between strata in both montane forest and scrub phytophysiognomies (Fig. 2B, Fig. 2C).
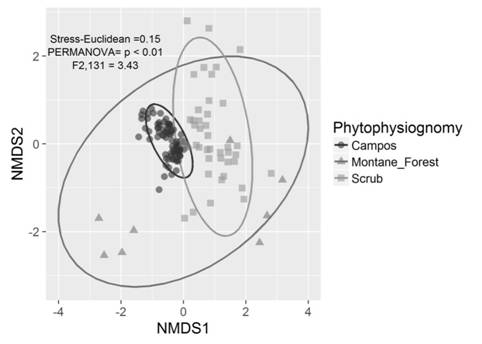
Fig. 1 Non-metric multidimensional scaling (NMDS) based on species composition on the quartzite rocky complexes in the municipalities of Santa Bárbara and Ouro Preto, Minas Gerais State, Brazil. Herbaceous campo rupestre is identified as Campos.
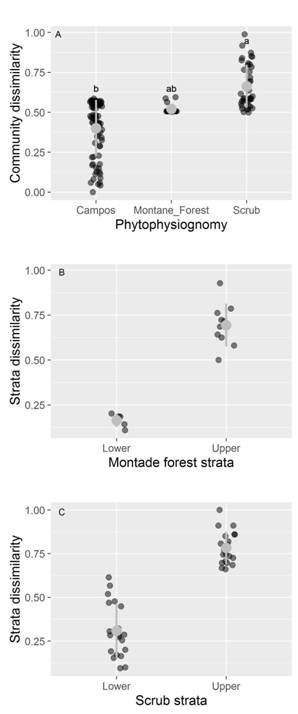
Fig. 2 Plant community dissimilarity of the species in different phytophysiognomies of the quartzite rocky complexes, Iron Quadrangle, Minas Gerais State, Brazil. The dark gray circles represent the plots in A. different phytophysiognomies, and in different strata, B. montane forest and C. The gray point and range indicates mean values and standard deviations. Different letters indicate significant differences (p < 0.05) among the sampled phytophysiognomies.
In herbaceous campo rupestre 3 413 individuals were sampled, among 107 species, 56 genera and 31 families. Asteraceae (22 species), Poaceae (nine species), Fabaceae and Cyperaceae (seven species each), Myrtaceae and Rubiaceae (five species each) were the richest families, representing 51.04 % of the species sampled in this phytophysiognomy. In the upper scrub stratum, 625 individuals were sampled, distributed among 52 species, 35 genera and 25 families. Myrtaceae was the richest family (10 species), followed by Melastomataceae (six species) and Fabaceae (five species). In the lower scrub stratum, 229 individuals were sampled, covering 73 species, 35 genera and 25 families. In this sampling, Poaceae (nine species), Melastomataceae (nine species), Hypericaceae (nine species), Rubiaceae (six species), Myrtaceae (four species), Asteraceae (eight species) and Fabaceae (three species) were the main families.
In the upper montane forest stratum, 141 individuals were sampled, encompassing 40 species, 25 genera and 24 families. The richest families were Myrtaceae (14 species) and Anacardiaceae (two species). In the lower montane forest stratum, 38 individuals were sampled, 13 species, 13 genera and 11 families. The richest families were: Fabaceae (eight species), Melastomataceae (six species), Aspleniaceae and Poaceae (five species each), Peraceae and Rubiaceae (four species each).
Species diversity and abundance pattern: We observe contrasting richness pattern between phytophysiognomies with differences using both sample-based and individual-based rarefaction and extrapolation curves (Fig. 3). Thus, rarefaction and extrapolation curves showed higher species richness in scrubs than in herbaceous campo rupestre with montane forest phytophysiognomies. Likewise, species richness curves showed significant differences between forest strata of montane forest and scrubs (Fig. 3).
Species richness (x2 = 34.36, df = 2, P < 0.001), Shannon diversity index (x2 = 24.62, df = 2, P < 0.01), Pielou evenness (x2 = 16.21, df = 2, P < 0.01), and abundance (x2 = 9.03, df = 2, P < 0.01) showed differences between phytophysiognomies (post hoc Dunn’s test P < 0.05, Fig. 4).
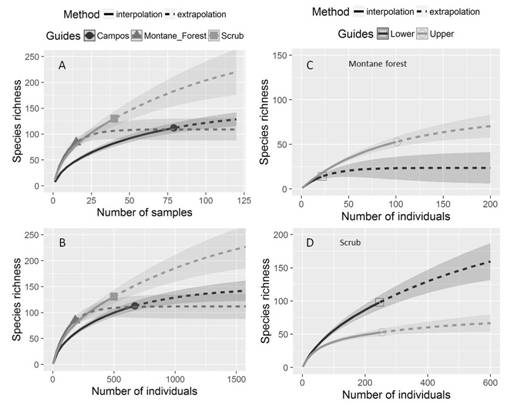
Fig. 3 Individual-based and sampled-based rarefaction (solid lines) and extrapolation curves (dashed lines) for the sampled areas in three phytophysiognomies (A-B), and Individual-based rarefaction and extrapolation curves in vertical richness distribution (lower and upper stratum) of diversity in C. montane forest and D. scrub. Herbaceous campo rupestre is identified as Campos. Rarefaction and extrapolation curves present the lines that represent the mean values and the bands the standard deviation with 95 % confidence intervals. The filled circles represent the plots of herbaceous campo rupestre, triangles represent the montane forest patches and squares represent the scrubs. The empty circles and squares represent upper and lower respectively for both montane forest and scrub.
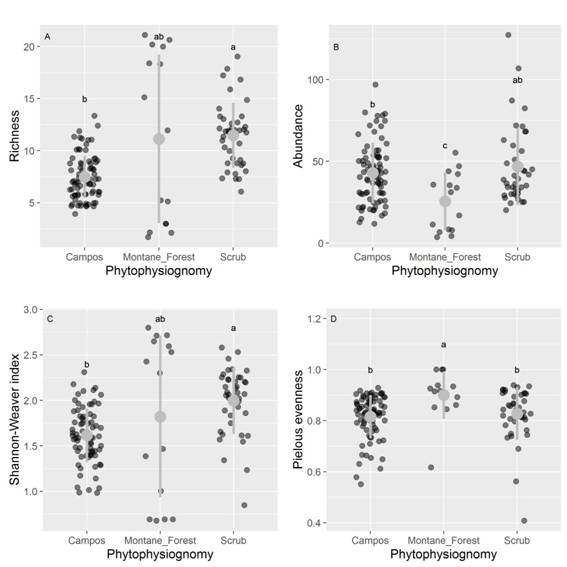
Fig. 4 A. Differences in species richness, B. abundance, C. Shannon-Weaver diversity and D. Pielou’s evenness along different phytophysiognomies of the quartzite rocky complexes, Iron Quadrangle, Minas Gerais State, Brazil. Herbaceous campo rupestre is identified as Campos. The dark gray circles represent the plots in different phytophysiognomies. The gray point and range indicates mean values and standard deviations. Different letters indicate significant differences (p < 0.05) among the sampled phytophysiognomies.
Life forms: There were significant differences in the proportion of the number of species (df = 8; x2 = 342.99; p < 0.001) and individuals (df = 8; x2 = 1 157.59; P < 0.001) in all the life form between phytophysiognomies (Fig. 5). Among the sampled species, 140 were considered phanerophytes, 41 hemicryptophytes, 29 camaephytes, five therophytes and three geophytes. Phanerophytes were the most abundant species and individuals ones, with 2 364 individuals, followed by hemicryptophytes (1 443 individuals), therophytes (265 individuals), geophytes (213 individuals) and chamaephytes (161 individuals).
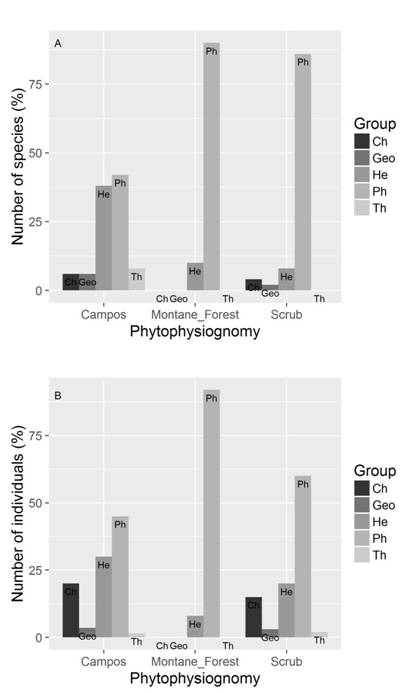
Fig. 5 A. Biological spectrum and B. vegetation spectrum sampled along different phytophysiognomies of the quartzite rocky complexes, Iron Quadrangle, Minas Gerais State, Brazil. Herbaceous campo rupestre is identified as Campos. Phanerophytes (Ph), hemicryptophytes (He), camaephytes (Ch), therophytes (Th) and geophytes (Geo) are indicated.
Discussion
Floristic composition: Our results showed that species composition varied considerably among phytophysiognomies. Asteraceae, the richest families in our study, were consistent with other floristic surveys performed in campos rupestres of the Iron Quadrangle and Espinhaço range (Conceição & Pirani, 2007; Mourão & Stehmann, 2007). Poaceae and Fabaceae was also among the most important ones. The success of these families may be due to their ability to colonize poor and shallow soils under high insolation, which are important environmental filters in these rocky complexes (e.g., Silveira et al., 2015; Zappi, Moro, Meagher, & Lughadha, 2017). Regarding distant ironstone, such as the Carajás Mountain, in the state of Pará, Northern Brazil, Fabaceae was reported to be the most important family in the shrubby ironstone campo rupestre (Nunes, 2009). In the Iron Quadrangle flora assessed in the present study, Fabaceae also appears frequently among the richest families. This species diversity pattern might be due to the genetic diversity, which results in morphological adaptations to several different environmental stresses (Oliveira, Souza, & Loiola, 2012), e.g. tolerance to desiccation and the ability to grow in dry open areas (Kellogg, 2001).
We observed that species similarity among herbaceous campo rupestre phytophysiognomies plots was low in comparison to montane forest and scrubs. The biophysical drivers associated with the high environmental specificity of plant species probably explains the great differences in floristic similarity between rupestrian grasslands within the Espinhaço Mountains (Alves et al., 2014; Silveira et al., 2015). In this sense, recent studies show how both lack of nutrients and shallow soil substrate determine vegetation physiognomy (Alves et al., 2014), and are of critical importance to plant distributions in campo rupestre (Alves et al., 2014; Fernandes, 2016; Zappi et al., 2017). They also conclude that the use of environmental contrasts (geologically different substrates, open versus forested vegetation) provides a useful framework for investigating diversity patterns in campo rupestre.
Species diversity and abundance pattern: Our study area showed high species richness in relation to a small spatial scale, coinciding with similar patterns observed in other Braziliam highlands (Tinti, Schaefer, Nunes, Rodrigues, Fialho, & Neri, 2015; Neri et al., 2016; Campos et al., 2018). Thus, despite a smaller sample area, the montane forest showed high diversity (richness and Shannon index) compared with the other phytophysiognomies. This pattern is probably due to a more heterogeneous vertical distribution of vegetation (lower and upper strata) compared to herbaceous campo rupestre that present a homogenous distribution, suggesting stability in the abundance of their species (Battilani, Scremin-Dias, & Souza, 2005). Likewise, according to the extrapolation using an individual-based approach for the montane forest, it is presumed that the species richness could be much higher in that same spatial scale.
Conversely, Shannon and Pielou’s evenness indices showed no regular pattern, which does not differ between phytophysogmies, despite the marked differences in species richness. This pattern of diversity may presumably be related to a greater relative uniformity in the species abundance distribution due to resource constraints (i.e., water, nutrients) and spatial distribution. Furthermore, this diversity pattern has recently been demonstrated for highlands in Southeastern Brazil, where it is inferred that communities are relatively stable regarding the distribution of their abundances (Campos et al., 2018). This assumption is based on the premise that recently campo rupestre was considered one of the world’s old climatically-buffered infertile landscapes with high levels of local endemism (Silveira et al., 2015).
Phytosociological analysis in the upper scrub strata showed Eremanthus erythropappus to be the predominant species. In the ecological succession process, E. erythropappus is considered a pioneer species, as it is the precursor of field invasions and colonizes poor, sandy and even rocky soils (Messias, Leite, Neto, & Kozovits, 2012). In the montane forest some of these species are frequently found in semi-deciduous seasonal forest formations in the Iron Quadrangle region (Spósito & Stehmann, 2006).
Our result showed that the lower and upper strata diversity and similarity of the scrub and montane forest were decoupled. The low diversity found in these phytophysiognomies may be related to low light availability, because the montane forest presents a closed canopy and irregular height. Plants with high emergence velocity and high initial growth rates have advantages in using environment resources (Gustafson, Gibson, & Nickrent, 2004). Thus, we expect there to be low light availability in the understory of a closed canopy (Nicotra, Chazdon, & Iriarte, 1999), and consequently low species richness and abundance under such conditions. Other studies have found that the compositional decoupling between canopy and recruitment communities is primarily associated with soil factors (e.g., Anderson, Morrison, Rugemalila, & Holdo, 2015). On the other hand, some studies have shown that plant coverage increases species richness and diversity but not species evenness (e.g., Sanaei, Ali, Chahouki, & Jafari, 2018), which may be related to the high canopy density of the vegetation through niche differentiation, and hence may promote species coexistence through niche facilitation (Grytnes & Birks, 2002; Sanaei et al., 2018). An alternative and complementary way to analyze these diversity patterns in rocky complexes, could be through species abundance distribution and species-area relationship analysis to elucidate the processes that maintain diversity (Villa et al., 2018b).
Life forms: Phanerophytes were the most representative life form in our study, which is consistent with other studies on Brazilian quartzite (Conceição & Giulietti, 2002; Conceição & Pirani, 2007), granite (Caiafa & Silva, 2005; Neri et al., 2016; Campos et al., 2018), and ironstone (Mourão & Stehmann, 2007). The high number of phanerophytes found in our study area was mainly due to sampling the upper stratum of the montane forest and scrub. In the herbaceous campo rupestre, the hemicryptophytes stood out, mainly represented by Poaceae and Cyperaceae. This life form is can survive under unfavorable climatic conditions (e.g. winter; dry season), since their buds are protected by scales or by dry leaves from the previous season.
In the montane forest and scrub, phanerophytes represented a significant number of species and individuals. Among the phanerophytes observed in these phytophysiognomies, there are some that are also frequent in forest phytophysiognomies, in both the Cerrado and the Atlantic Rainforest; e.g. E. erythropappus, E. incanus, Guatteria vilosissima, Myrcia splendens, Tapirira guianensis and Vismia brasiliensis (Messias et al., 2012). The dominance of this life form in these environments can be explained by the greater soil depth, the occurrence of fissures in the rocks which enable rooting of higher species, and also by the slightly higher amounts of certain nutrients in these microhabitats (Vincent & Meguro, 2008). Additionally, the higher water retention capacity enables a higher intake of the small amounts of nutrients available.
Our study reveals marked differences in floristic composition, richness, and life form spectra between phytophysiognomies on the quartzite rocky complexes in the southeast Brazilian Iron Quadrangle. Such differences probably indicate that there is a high environmental heterogeneity at small spatial scales. We suggest future studies evaluate the effect of environmental drivers on the diversity changes along campo rupestre gradients, beyond a floristic evaluation.