Introduction
Aedes aegypti is one of the most important vectors in the maintenance and transmission of viruses such as chikungunya, Zika, yellow fever, Mayaro and dengue (Paniz-Mondolfi, Rodriguez-Morales, Blohm, Marquez, & Villamil-Gomez, 2016), the latter producing the most prevalent and dangerous emerging arboviral disease (Patterson, Sammon, & Garg, 2016). The Pan American Health Organization (Pan American Health Organization, 2019) has reported an increase in dengue cases in the Americas over the last four decades, and nearly 500 million people are at risk of contracting the disease. More than 1.6 million cases were recorded in the Americas in 2020 alone, of which 9,438 were reported in Costa Rica as of October of that year (Gonzalez, 2021; Pan American Health Organization, 2020).
This increase in the number of cases impacts public health and presents a high economic cost for countries because productivity and household income are reduced by disability or death, investment in vector control equipment (human resources and infrastructure) and the follow-up and treatment of sick people (World Health Organization, 2017).
Vector control is a preventive and effective way of reducing the transmission of diseases by arthropods and promoting human and economic development (World Health Organization, 2004, 2017). The main control method implemented in the Americas has been the use of pesticides based on different chemical groups, such as organochlorines, organophosphates, carbamates and pyrethroids. However, excessive pesticide use, the absence of surveillance of doses used and the susceptibility of the vector to insecticides have resulted in the emergence of resistant A. aegypti populations (Lounibos & Kramer, 2016; Manjarres-Suarez & Olivero-Verbel, 2013). The indiscriminate use of these chemicals also has harmful effects on ecosystems and public health (World Health Organization, 2017). Costa Rica does not have an insecticide susceptibility surveillance program. However, the results of sporadic studies have shown no resistance in A. aegypti to temephos or deltamethrin but resistance to cypermethrin in a strain in Orotina (Bisset et al., 2013; Calderón & Troyo, 2016; Calderón-Arguedas & Troyo, 2014; Vargas Miranda, Troyo, & Calderón Arguedas, 2019).
Considering the current situation regarding vector control, it is important to develop natural chemical alternatives that help reduce the vector population, while generating minimal resistance, and less toxic to mammals, less harmful to the environment and more affordable (Muñoz V., Staschenko, & Ocampo D., 2014; Silvério, Espíndola, Lopes, & Vieira, 2020).
Research studies based on natural active principles in the Americas have mainly focused on the larvicidal activity and repellency of essential oils (Pilon et al., 2022). However, such information is not available for Costa Rica (Hasan, Jaal, Ranson, & McCall, 2015; Leyva-Silva et al., 2017; Manjarres-Suarez & Olivero-Verbel, 2013; Muñoz V. et al., 2014; Rey & Lounibos, 2015; Vargas Miranda et al., 2019).
Therefore, the aim of the present study is to determine the larvicidal activity against A. aegypti of ethanolic extracts of plants commonly found in Costa Rica.
Methodology
This study was carried out at the Phytochemistry Laboratory in the School of Chemistry of the National University of Costa Rica.
Plant samples
The 13 selected plants fulfilled at least two of the following inclusion criteria: being a diverse source of secondary metabolites; having a known ethnomedical use for the biological control of bacteria and/or arthropods; and being widely distributed and/ or affordable in Costa Rica (Table 1).
Samples of stems, leaves and, in some cases, seeds, were collected at the Quinta Diamante Sociedad de Responsabilidad Limitada Experimental Station in Pérez Zeledón, San José. The Ipomoea cairica sample was collected in the San Miguel de Santo Domingo sector of Heredia. All the samples were collected and processed under CONAGEBIO permit no. R-012-2020-OT-CONAGEBIO. The samples were dried in an oven at 40 °C and ground in a knife mill with a 1-mm sieve.
Table 1 Plant species used by family and habit
Species | Family | Habit |
---|---|---|
Ipomoea cairica | Convolvulaceae | Vine |
Piper umbellatum | Piperaceae | Herb |
Piper marginatum | Piperaceae | Shrub |
Zanthoxylum setulosum | Rutaceae | Tree |
Argemone mexicana | Papaveraceae | Erect herb |
Bocconia frutescens | Papaveraceae | Shrub to small tree |
Quassia amara | Simaroubaceae | Shrub to small tree |
Neurolaena lobata | Asteraceae | Epiphyte |
Solanum mammosum | Solanaceae | Shrub |
Erythroxylum macrophyllum | Erythroxylaceae | Shrub |
Croton draco | Euphorbiaceae | Herb or Shrub |
Pimenta dioica | Myrtaceae | Tree |
Rosmarinus officinalis | Lamiaceae | Shrub |
Kalanchoe sp. | Crassulaceae | Herb-shrub |
Note: derived from research.
Extraction
Three extractions of dry and ground material were performed using 95% ethanol in an ultrasonic bath at 40 °C. The extract was filtered using gravity filter paper. The collected filtrate was concentrated using a vacuum rotary evaporator at a temperature not higher than 40 °C. The extracts were then freeze dried to produce dry and stable samples. The dry samples were weighed, and homogeneous solutions of known concentrations of each extract were prepared and applied to the larvae, as described in the following section.
Evaluation of larvicidal activity
The larvicidal activity was determined using a method described in the WHO/VBC/81.807 report of the World Health Organization (World Health Organization, 1981). The third to fourth instar larvae of the Rockefeller strain of A. aegypti susceptible to insecticides were used. To ensure physiological homogeneity for each trial, the larvae were obtained from eggs that were hatched simultaneously and raised under the same feeding and environmental conditions. Four replicates per sample concentration were used, with approximately 20 larvae each and a total volume of 25 mL of the mixture methanol: water 2:98 v:v. A positive control containing 50 ppm (mg/L) temephos and a negative control containing a mixture of methanol : chlorinated tap water (2:98) were used. Mortality was read at 24 hours. The extracts were initially evaluated at a concentration of 100 ppm. As this study was exploratory, the six extracts with the highest mortality were analyzed. For plant extracts in which a mortality percentage ≥7.9% was obtained, a response evaluation was performed at concentrations ranging from 50 ppm to 400 ppm. The LC50 and LC90 were obtained by a PROBIT analysis using XLSTAT version 2021.2 software19.
Analysis and results
The evaluation results for 100-ppm extracts of the 13 plant species are shown in Table 2. Ten of the species showed a nonzero percentage of larvicidal activity at 24 hours. Ipomoea cairica, Zanthoxylum setulosum, Piper umbellatum, Rosmarinus officinalis and Kalanchoe sp. exhibited a mortality greater than or equal to 7.9%. I. cairica had the highest mortality at 100%.
In all the tests, both the positive control containing temephos and the negative control containing water (or the corresponding mixture of solvents) exhibited the expected mortality rates of approximately 100% and 0%, respectively, indicating optimal conditions for all the tests.
Figure 1 is a regression plot of the A. aegypti larval mortality versus the logarithm (base 10) of the natural insecticide concentration. The slope of the regression plot indicates the degree of homogeneity of the population response to the stimulus, that is, the higher the slope is, the higher the homogeneity of the population response to the stimulus (Leyva et al., 2008). The highest slope was obtained for I. cairica at 2.7727, followed by Z. setulosum at 2.5495. The slope obtained from the regression equation can be used to determine the concentration range over which a substance with larvicidal activity can exert a well-defined mortality effect. The slopes determined in this study were lower in comparison with literature values (Amariles Barrera, García Pajón, & Parra Henao, 2013; Leyva et al., 2008).
Table 2 Larvicidal effect produced by ethanolic extracts of plants at 100 ppm on A. aegypti Rockefeller strain larvae
Species | Plant part* | Dead larvae | Total larvae | Mortality percentage at 24 h |
---|---|---|---|---|
Ipomoea cairica | L | 32 | 80 | 40.0 ± 0.0 |
S | 80 | 80 | 100.0 ± 0.0 | |
Piper umbellatum | L | 7.0 | 76.0 | 9.2 ± 2.6 |
S | 8.0 | 81.0 | 9.9 ± 0.2 | |
Zanthoxylum setulosum | L | 8.0 | 81.0 | 9.8 ± 3.8 |
S | 2.0 | 80.0 | 2.5 ± 5.0 | |
Argemone mexicana | SD | 0.0 | 76.0 | 0.0 ± 0.0 |
L | 4.0 | 76.0 | 5.3 ± 0.1 | |
S | 2.0 | 76.0 | 2.6 ± 3.0 | |
Bocconia frutescens | M | 1.0 | 76.0 | 1.3 ± 2.6 |
L | 0.0 | 76.0 | 0.0 ± 0.0 | |
S | 0.0 | 76.0 | 0.0 ± 0.0 | |
Quassia amara | L | 0.0 | 76.0 | 0.0 ± 0.0 |
S | 0.0 | 76.0 | 0.0 ± 0.0 | |
Neurolaena lobata | L | 0.0 | 76.0 | 0.0 ± 0.0 |
S | 2.0 | 76.0 | 2.6 ± 3.0 | |
Solanum mammosum | L | 4.0 | 76.0 | 5.3 ± 0.1 |
S | 0.0 | 76.0 | 0.0 ± 0.0 | |
Erythroxylum macrophyllum | L | 1.0 | 76.0 | 1.3 ± 2.6 |
S | 0.0 | 76.0 | 0.0 ± 0.0 | |
Croton draco | L | 0.0 | 76.0 | 0.0 ± 0.0 |
S | 0.0 | 76.0 | 0.0 ± 0.0 | |
Pimenta dioica | L | 0.0 | 76.0 | 0.0 ± 0.0 |
S | 0.0 | 76.0 | 0.0 ± 0.0 | |
Rosmarinus officinalis | L | 6.0 | 76.0 | 7.9 ± 3.0 |
Kalanchoe sp. | L | 2.0 | 76.0 | 2.6 ± 3.0 |
+ Control (temephos) | 80.0 | 80.0 | 100.0 ± 0.0 | |
- Control (water) | 1.0 | 80.0 | 1.3 ± 0.5 |
*Legend: L = leaves; S = stems; M = midrib; SD = seeds. The uncertainty in the mortality data are expressed as the standard deviation for four replicates. Note: derived from research.
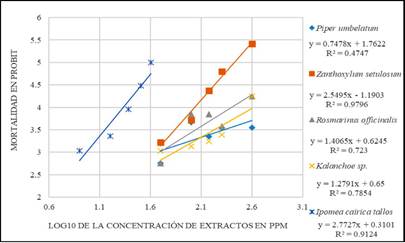
Note: derived from research
Figure 1 PROBIT plots of the logarithmic (base 10) concentration (ppm) of the ethanolic extracts of the evaluated plants versus mortality (PROBIT units).
The LC50 and LC90 values for the evaluated ethanolic extracts are shown in Table 3. The species I. cairica species exhibited the lowest LC50, indicating that this extract elicited the highest mortality response at low concentrations.
The results of the larvicidal study revealed a diverse mortality response in the third and fourth instar larvae of the A. aegypti Rockefeller strain to 100 mg/mL (ppm) ethanolic extracts of the selected plants. The mortality rates observed for the ethanolic extracts of I. cairica were 40.0% for leaves and 100% for stems. By contrast, the mortality rates observed for the ethanolic extracts of P. dioica, C. draco and Q. amara were 0.0% for both leaves and stems at the same concentration in all cases. This result was expected because differences in the chemical compositions of the plants affect the presence of substances with larvicidal effects in the extracts, which generates variations in larval mortality (Jirovetz et al., 2007; Mora, Castro, Chavarría, Murillo, & Poveda, 2011; Patel & Patel, 2018; Setzer et al., 2007). The ethanolic extracts with the highest larvicidal activity were those of I. cairica, followed by P. umbellatum, R. officinalis and Kalanchoe sp. Other ethanolic extracts produced mortality for only one part of the studied plant: for example, a mortality of 5.3 ± 0.1% was observed for the ethanolic extract of the leaves of S. mammosum, whereas no mortality was observed for at the same concentration of the ethanolic extract of the stems. This result suggests that secondary metabolite compositions differ among plant parts, in terms of both concentration and type (Araya, Carvajal, Alvarez, Orozco, & Rodriguez, 2017; Leal et al., 2020; Rangiah & Gowda, 2019). It is notable that, despite the extract method used that does not concentrate volatile fraction of the compounds present in the plant, it was possible to find a highly lethal components int the extract with LC50 values like the ones reported in studies where evaluate the toxicity of volatile fractions of plants such as essential oils (Pilon et al., 2022).
A significant degree of toxicity was previously reported for Z. setulosum, mainly against Artemia salina, with an LC50 of 3.9 mg/mL(Boehme, Noletto, Haber, & Setzer, 2008). Hydro distillation was used to extract terpenes, terpenoids and derivatives in the respective study, because the aim was to
Table 3 LC50 and LC90 data expressed as parts per thousand (mg/mL) for the ethanolic extract evaluated against A. aegypti larvae
Plant | LC 50 in mg/mL (*95% CI) | LC90 in mg/mL (*95% CI) | Diagnostic dose in mg/mL (*95% CI) |
---|---|---|---|
Ipomoea cairica | 0.0341 | 0.0578 | 0.978 |
(stems) | (0.0293-0.0393) | (0.0496-0.0674) | (0.840-1.140) |
Ipomoea cairica | 0.121 | 0.337 | 3.707 |
(leaves) | (0.089-0.166) | (0.246-0.462) | (2.52-4.89) |
Zanthoxylum setulosum | 0.268 | 0.854 | 24.21 |
(leaves) | (0.185-0.386) | (0.592-1.232) | (16.78-34.93) |
Rosmarinus officinalis (leaves) | 3.41 | 36.03 | 2709.79 |
(1.12-10.36) | (11.85-109.54) | (891.29-8237.12) | |
Kalanchoe sp. | 11.14 | 320.26 | 54445 |
(leaves) | (2.405-51-605) | (69.15-1483.2) | (11755-252153) |
Piper umbellatum (stems) | 39.60 | 3885.69 | 1797603 |
(6.86-228.52) | (673.30-22424.70) | (311483-10374157) |
*CI: Confidence interval. Note: derived from research.
extract mainly essential oils, and therefore, the volatile fraction of the plant. The aim of the present study was to obtain mainly the nonvolatile fraction of plants because multiple bioactivities have been reported (Araya et al., 2017; Gutierrez, Arenas, Barrera, & Martínez, 2007; Parra et al., 2018) for a large number of secondary metabolites that are not necessarily volatile, such as polyphenols, nitrogenous compounds and alkaloids (Pilon et al., 2022). Thus, there was an important methodological difference between the two studies. In addition, toxicity was measured in the two studies using different species, both belonging to the phylum Arthropoda, but to different orders.
The significant LC50 of the ethanolic extract of Z. setulosum was lower than that of the other evaluated species; however, the ethanolic extract of I. cairica exhibited an even lower LC50. I. cairica had a relatively high diagnostic dose (DD) of 0.978 mg/mL compared to those reported for commercial insecticides, such as temephos, which can reach 1 mg/L (World Health Organization, 2009). However, as a crude extract of I. cairica was used in this study, either the compounds producing larvicidal activity were present in small proportions in the extract or a combination of various metabolites generated synergistic activity (Keziah, Nukenine, Yingyang Danga, & Esimon, 2016; Liu et al., 2002).
The slope of the PROBIT plot is higher for the ethanolic extract of P. umbellatum leaves than for the extracts of R. officinalis and Kalanchoe sp. However, the low linearity of the fit (R2 = 0.4747) reflects a weak correlation in the P. umbellatum data, that is, a poor fit between the concentration and mortality compared to that for the R. officinalis and Kalanchoe sp. extracts. The slope of the PROBIT plot is an important consideration because a large slope shows greater effects, i.e., higher mortality, at low concentrations, as shown in Figure 1.
The ethanolic extract of I. cairica was expected to exhibit lower LC50 and LC90 values than the other evaluated ethanolic extracts because the largest slope of the PROBIT curves was obtained for I. cairica. Thus, I. cairica produced similar mortalities at low extract concentrations, and the aforementioned factors made the highest contribution to the linear regression analysis. Alkaloids, carbohydrates, tannins, phenolic compounds, proteins, amino acids, terpenoids, sterols, saponins, lignans and coumarins have been found in I. cairica 34,35. The two simple coumarins that have been found in I. cairica are scopoletin (7-hydroxy-6-methoxychromen-2-one) and umbelliferone 7-(hydroxychromen-2-one) (Olga & Braz-Filho, 1997). This result is important because the toxic activity of natural extracts has been correlated with the presence of coumarins in many studies and may explain the activity reported in the present study (Ahbirami, Zuharah, Thiagaletchumi, Subramaniam, & Sundarasekar, 2014; Prabha & Nagarajan, 2016; Wang, Kim, Wang, Shu, & Ahn, 2012).
Conclusions
The plants that produced ethanolic extracts with the highest larvicidal responses (I. cairica, P. umbellatum, Z. setulosum, R. officinalis and Kalanchoe sp.) were evaluated in the last stage of the study. With the exception of R. officinalis, these plants are not used for culinary or other commercial purposes and therefore have no significant commercial value.
Natural extracts with larvicidal activity are an alternative to synthetic products in commercial use. Synthetic insecticides that do not degrade easily exert pressure on biological systems, whereas a product of natural origin is incorporated back into an ecosystem after completing its function (Greenop, Cook, Wilby, Pywell, & Woodcock, 2020).
The use of field samples, in addition to the Rockefeller strain, for testing has epidemiological utility for assessing cross-resistance to the active principles of I. cairica in Costa Rica (Haziqah-Rashid et al., 2019). The results of this study are an important contribution in the exploitation of natural extracts with larvicidal activity. As no studies have been performed to evaluate the larvicidal activity of nonvolatile plant extracts, the main approach used in this study was to evaluate essential oils instead of compounds that cannot be extracted by conventional hydro distillation methods and for which bioactivity has been reported in various cases(Boehme et al., 2008; Muñoz V. et al., 2014; Pinto, Menezes, Melo, & Feitosa, 2016). The results of this study show larvicidal activity for ethanolic extracts of different plants, with an emphasis on the larvicidal potential of I. cairica as an environmentally friendly alternative for the control of A. aegypti.
Funding
The translation of this study was funded by the ''Fund to support the dissemination of knowledge generated at National University (Fondo para apoyo a la divulgación del conocimiento generado en la UNA)''according to resolution UNA-VI-RESO-116-2021.
Universidad Nacional, Costa Rica.
Acknowledgements
Special mention to Dr. Marco Herrero Acosta who was consulted during the execution of this project, M.Sc. Allan González Herrera, who facilitated working space during part of the project. Additionally, extend to the Universidad Nacional program SIA code 0568-14 name: Laboratorio de Fitoquímica an special mention in the collaboration of this project.
Author contribution statement
All the authors declare that the final version of this paper was read and approved.
The total contribution percentage for the conceptualization, preparation, and correction of this paper was as follows: V.A.V. 60 %., G.R.R. 20 % and S.A.G. 20 %.
Data availability statement
The data supporting the results of this study is available as ''supplementary files''on the Uniciencia website