The southern Pacific region of Costa Rica comprises landscapes of extraordinary environmental diversity, with coastal environments, islands, and large alluvial valleys bounded by mountain ridges that cross the territory in different directions. These landscapes have been occupied since 1500 BCE by people who developed practices of marine harvesting, fishing in the ocean and inland waters, hunting, and later the cultivation of crops, becoming specialized farmers in early times (Corrales, 2000; Sánchez & Rojas, 2002; Anchukaitis & Horn, 2005; Horn, 2006; Baldi, 2011; Sánchez, 2013).
To understand and interpret the consumption of plants and animals in the past requires the investigation of diverse lines of evidence. Identifiable macroscopic remains of plants and animals, for example seeds and bones, are often found at archaeological sites and provide key data on food resources. Their analysis is complemented by the study of pollen grains or phytoliths of cultivated plants within archaeological horizons (Bryant & Holloway, 1983; Piperno, 2006), or in sediment cores recovered from lakes and wetlands near archaeological sites (Northrop & Horn, 1996; Clement & Horn, 2001; Horn, 2006; Kennedy & Horn, 2008). Another important source of information on human diets in the past consists of food residues preserved in or on artefacts excavated from archaeological sites (Heron & Evershed, 1993).
Here we focus on isotopic analyses of carbonized food residues preserved on the inner surfaces of ceramic pots, plates, and other earthenware from the southern Pacific region of Costa Rica. Our study represents the first comparative analysis of surface food residues from Costa Rican pottery and indicates the potential for studies of this type to strengthen our understanding of indigenous subsistence patterns through time and across Costa Rican archaeological regions.
Some additional analyses of the isotopic composition of food residues in Costa Rican pottery from individual sites were presented at the XI Congreso de la Red Centroamericana de Antropologia, held at the University of Costa Rica in March 2017, in a session on the analysis of stable isotopes in archaeology organized by Sánchez and Horn. These other analyses, as yet unpublished, grew from the present study; they are collaborations that also involve Horn and Lane, with University of Tennessee Ph.D. candidate Matthew Kerr, and Costa Rican archaeologists. Other studies of stable isotopes in Costa Rica with application to archaeology include work on stable carbon and nitrogen isotopes in collagen in human bones from prehistoric burials (Norr, 1991, 1996), and studies of stable carbon and nitrogen isotopes in lake-sediment cores (Lane, Horn, & Mora, 2004; Lane, Horn, Taylor, & Mora, 2009; Taylor, Horn, & Finkelstein, 2013, 2015; Kerr, 2014; Horn & Haberyan, 2016; Johanson, 2016) and soils (Kerr, Boehm, Lane, & Horn, 2014) at archaeological sites.
Isotopic analysis of surface food residues on pottery relies on the fact that the ratios of heavy to light stable isotopes of carbon (13C vs. 12C) and nitrogen (15N vs. 14N) in potential foods differ. To convert these ratios into large, whole numbers and for comparability between studies, carbon and nitrogen isotope values are reported in δ-per mille notation relative to standards. Plants that photosynthesize using the C3 photosynthetic process (all trees and most species of shrubs, herbs, and vines in Costa Rica) produce plant tissue with lower (more negative) δ13C values (-35 to -20‰) than plants that photosynthesize using the C4 pathway (maize and other grasses, and several agricultural weeds), which are relatively enriched in 13C (-14 to -10‰) (Bender, 1971; O’Leary, 1981). Plants that are associated with nitrogen-fixing bacteria (beans and other legumes) have δ15N values similar to the atmosphere (~0‰), while other plants typically have higher δ15N values (>2‰; Morton & Schwarcz, 2004). Nitrogen isotope signatures in animals are affected by trophic level, with each increase in trophic level associated with a 1 or 2‰ increase in δ15N values (Peterson & Fry, 1987; Heron & Evershed, 1993). Stable carbon isotope values in animals are similar to that of their collective diet, but are sometimes slightly enriched in 13C compared to their diet (Morton & Schwarcz, 2004). Hastorf and DeNiro (1985) and DeNiro (1987) were the first to investigate the stable isotope compositions of carbonized residues in ceramics as a method for characterizing plant foods prepared and eaten in prehistory. Working with pottery from the Upper Mantaro Valley in the central Peruvian Andes, they showed it was possible to use δ13C and δ15N in charred residues to identify three groups of plants: leguminous C3 plants (beans), non-leguminous C3 plants (quinoa or tubers), and C4 plants (maize) (Hastorf & DeNiro, 1985). To develop and confirm their method, they investigated δ13C and δ15N values in modern crops and carried out laboratory analyses of the effects of burning on isotope ratios and their stability. They found that when a plant is burned onto a ceramic pot the δ13C and δ15N values of the residues were within 3‰ of their original values. They also demonstrated that the process of carbonization in some way stabilizes the plant residues, preventing further isotopic change following burial (DeNiro & Hastorf 1985; DeNiro 1987).
Morton and Schwarcz (2004) examined food residues in ceramics from Ontario, Canada. They identified isotopic signatures of animal as well as plant foods in carbonized residues. Their samples were from both before and after 600 CE, the time of the introduction of agriculture, largely maize-based, to the region. Most of the residue samples reflected a mixture of C3 plants and the flesh of herbivores and fish. Only a low importance of maize was found in the post-600 CE samples, and the researchers speculated that the δ13C values in the food residue could be from the meat of animals (such as dog or bear) that had fed on maize, rather than the preparation of maize. No post-agricultural shift in δ15N was apparent following the initiation of agriculture, showing low importance of beans; however, fish were an important protein source both before and after the introduction of agriculture.
Hart, Lovis, Schulenberg and Urquhart (2007) carried out laboratory simulations to test whether the low δ13C values in the post-agricultural pottery from Ontario might have another explanation. In a series of experiments they demonstrated a nonlinear relationship between the amount of maize cooked in a pot and the δ13C values of the residue. They suggested that Morton and Schwarcz (2004) may have underestimated the importance of maize at their sites, and that stable isotope analyses of food residues should be not be used in isolation to infer paleodiet.
A second study by Craig et al. (2007) echoed the theme of the Hart et al. (2007) study by referring to stable carbon isotope analysis of charred food residues as a “blunt tool” for distinguishing complex mixtures of food. Craig and collaborators supplemented stable carbon and nitrogen analyses of food residues in pots from coastal and inland sites in NW Europe with structural and molecular analyses of extracted lipids, to chart possible dietary changes following the adoption of agriculture. They were able to show that pots from both the Mesolithic and Neolithic were used to process aquatic organisms, demonstrating continued reliance on fish during the agricultural period.
The objective of our research was to examine food residues in archaeological pottery from coastal and interior sites in the southern Pacific region of Costa Rica. Our study, the first in the country comparing residues from multiple sites and time periods, complements archaeological and paleoecological research in the region, and sets the stage for later, more specialized analyses of food residues, including characterization of a variety of lipids and other specific compounds that can be extracted from visible or absorbed remains on/in ancient pottery (Hall, Tarka, Hurst, Stuart, & Adams, 1990; Reber & Evershed, 2004; Reber, Dudd, Van der Merwe, & Evershed, 2004; Reber & Hart, 2008; Hurst, 2009; Crown, Emerson, Gu, Hurst, Pauketat, & Ward, 2012). Here we report the stable carbon and nitrogen isotope ratios in charred food residues from prehistoric (and one historic) ceramic vessels from diverse contexts, and interpret the results in light of archaeological, historical, and paleoenvironmental evidence from the sites and their surroundings.
MATERIALS AND METHODS
The School of Anthropology at the University of Costa Rica has investigated several archaeological sites in the southern Pacific region of Costa Rica (Fig. 1). For this study, we sampled charred food residues adhered to the surface of thirteen ceramic vessels from six archaeological sites.
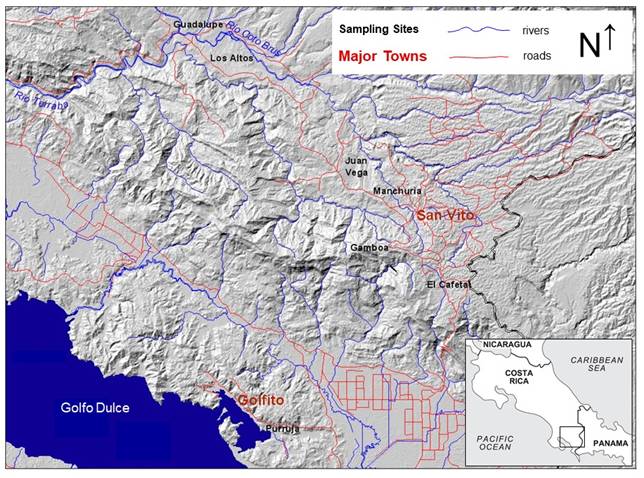
Fig.1. Locations of archaeological sites in southern Pacific Costa Rica selected for the study of stable isotopes of food residues in ceramics. Purruja refers to the site Alto Purruja. Guadalupe refers to the Iglesia de Guadalupe.
Twelve of the thirteen samples are prehistoric pottery vessels that range chronologically from 700 to 1500 AD (Table 1). One sample comes from a colonial site and corresponds to the period from 1571 to 1660 AD. Most of the samples came from defined contexts and were recovered in controlled excavations; however, to enlarge the data set a few vessels from surface reconnaissance were included.
The material selected for analysis is associated with varied environments that characterize the southern Pacific zone: coastal sites (Alto Purruja), piedmont (El Cafetal), alluvial valleys (Iglesia de Guadalupe), savannas (Los Altos), and mountain ridges (Gamboa, Juan Vega, and Manchuria). The functionality of the selected sites corresponds to households at Los Altos, Gamboa, Juan Vega and El Cafetal. Alto Purruja is a coastal site associated with the consumption of resources from shoreline and offshore habitats. The Iglesia de Guadalupe is a colonial seat of the Spanish crown, where a church was constructed that controlled the indigenous population.
TABLE 1: Material Selected for Analysis
Sample Number | Site | Source of Material | Age | Reference |
---|---|---|---|---|
1 | Los Altos | Sample from surface of. Mound XXV, Quadrant C3 | Probably 1300-1500 CE | Sánchez & Novoa, 2005 |
2 | Gamboa | Sample from test pit 16-N1 | Chiriquí Period (700-1500 CE) | Sánchez & Rojas, 2002 |
3 | Gamboa | Sample from test pit 1g N4 | Chiriquí Period (700-1500 CE) | Sánchez & Rojas, 2002 |
4 | Gamboa | Sample from Surface A | Chiriquí Period (700-1500 CE) | Sánchez & Rojas, 2002 |
5 | Gamboa | Sample from Surface B | Chiriquí Period (700-1500 CE) | Sánchez & Rojas, 2002 |
6 | Alto Purruja | Sample UCR-455 Op 3-S1-CB1 from shell mound | Aguas Buenas Period probably 600 CE | Rojas, 2000 |
7 | Alto Purruja | Test pit sample PZ1-4 from shell mound | Aguas Buenas Period probably 600 CE | Rojas, 2000 |
8 | Manchuria | Surface sample P-653 Ma. | Chiriquí Period (700-1500 CE) | Sánchez & Rojas, 2002 |
9 | Juan Vega | Sample from Profile UCR-495 Op. 4 N3 | Chiriquí Period (700-1500 CE) | Chávez, 2000 |
10 | El Cafetal | Sample from test pit P-642 | Chiriquí Period (700-1500 CE) | Sánchez & Rojas, 2002 |
11 | El Cafetal | Sample from test pit P-642 | Probably 1300-1500 CE | Sánchez & Rojas, 2002 |
12 | Alto Purruja | Sample 455-3-1-A2 | Aguas Buenas Period probably 600 CE | Rojas, 2000 |
13 | Iglesia de Guadalupe | Surface sample of 2 fragments of a ceramic vessel | Colonial (1571- 1660 CE) | Sánchez, 2013 |
Analysis of charred pottery residues followed the protocol of Morton and Schwarcz (2004). Pottery residues were removed from potsherds by manually scraping with a spatula. All residue particles were then rinsed with distilled water three times to remove any adhering soil or other organic contaminants. The rinsed samples were then soaked in a 0,1 N NaOH solution at 20°C overnight to remove humic acids. Minimal discoloration of the NaOH solution for all samples indicated minor humic acid contamination potential. All samples were then dried at 50°C overnight, ground to a fine powder using a mortar and pestle, and loaded into Sn capsules for isotopic analysis on a Costech 4010 Elemental Analyzer coupled to a Thermo-Finnigan XL+ Mass Spectrometer. Carbon and nitrogen isotopic data are reported in the delta (δ) per mille (‰) notation that relates to the Vienna Pee Dee belemnite (VPDB) and atmospheric nitrogen (AIR) standards, respectively, using the equation: δX= 1000*[(Rsample/Rstandard)-1)], where X represents 13C or 15N, and R represents 13C/12C or 15N/14N, respectively. Relative contributions of C3 vs. C4 plant-derived carbon to charred residues were estimated using the two end-member mixing model approach of Phillips and Gregg (2001) and assumed δ13C values of -27‰ and -12‰ for C3 and C4 plants, respectively.
Ethical, conflict of interest and financial statements: the authors declare that they have fully complied with all pertinent ethical and legal requirements, both during the study and in the production of the manuscript; that there are no conflicts of interest of any kind; that all financial sources are fully and clearly stated in the acknowledgements section; and that they fully agree with the final edited version of the article. A signed document has been filed in the journal archives.
RESULTS
The food residues analyzed reveal a wide variety in isotopic signatures (Fig. 2). The δ13C values of the residues range from -24,9 to -10,5‰ and the δ15N values range from 1,7 to 4,7‰. Fig. 2 consists of two panels. In the upper panel, the 12 food residue samples are plotted by their δ13C and δ15N values. The labeled boxes represent likely food resources and are based on values in the literature (Keegan & Deniro, 1998; Morton & Schwarcz, 2004; Tykot, 2004). The plotted positions of the samples relative to these boxes allow us to infer the composition of the food residue examined. A food residue sample could be composed of a single food source, in which case plotting the δ13C values against their δ15N values should result in the sample falling within the individual box. Or, the food residue could consist of a mixture of food sources with different expected isotopic signatures. In that case, the sample will plot between boxes.
Application of the mixing model to the δ13C data yields C4 contributions to the residues ranging from 14 to 100% (lower panel in Fig. 2), with the greatest apparent C4 plant contributions at El Cafetal and Los Altos and the lowest at Gamboa.
Stable isotope analysis of sample 1 from Los Altos yielded isotopic values that indicate that plant foods were exclusively from C4 plants, most likely maize. The δ15N value falls slightly below the expected value for the flesh of herbivores that consumed C4 plants, but close enough to allow the possibility that the food residue analyzed contained maize along with meat from C4 herbivores.
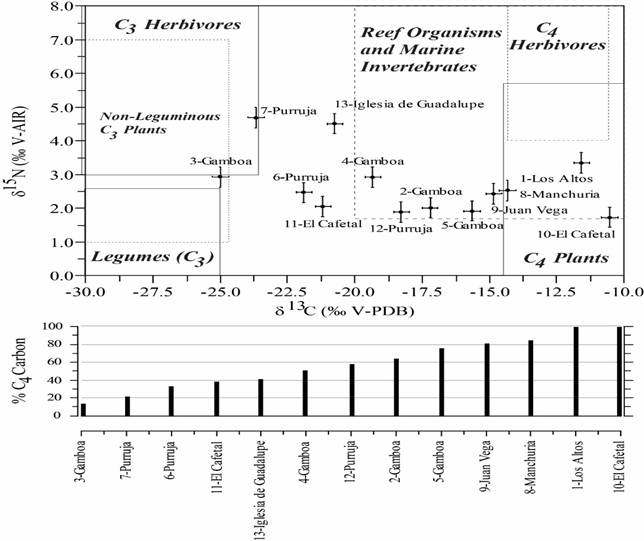
Fig.2. Bivariate crossplot of pottery residue δ13C and δ15N values for the pottery residues analyzed in this study (top panel). Boxes represent likely isotopic values of potential food resources in the region and are based on values presented in the literature (Keegan & Deniro, 1998; Morton & Schwarcz, 2004; Tykot, 2004). Parruja refers to the site Alto Purruja. Estimated C4 plant contributions to the residues at each site (bottom panel) based on a two end-member mixing model applied to the δ13C values.
The food residues in sample 2, 3, 4, and 5, all from the Gamboa site, showed some diversity in isotope signatures. The Gamboa site samples could potentially contain residues derived from reef or marine invertebrates, but they fall at the very low end of δ15N values typical of higher trophic level (herbivorous and carnivorous) organisms typically consumed from these environments. More likely, values for sample 2 indicate a mixture of C3 (most likely legumes) and C4 (maize), but mostly maize. Samples 3 indicates primarily C3 plants, likely a mixture of legumes and other C3 plants, and/or meat from C3 herbivores. Sample 4 is split evenly between C3 and C4 plants. Sample 5 indicates a mixture of C3 (most likely legumes) and C4 plants (maize), but mostly maize.
Food residue samples 6 and 7 were both collected from ceramics in shell mounds at the Alto Purruja site. Sample 6 indicates a mixture of C3 (most likely legumes) and C4 (maize) plants, but mostly C3 sources. Plant foods in sample 7 are mainly from C3 sources. In this sample, high δ15N values indicate potential consumption of C3 herbivores. Although these two samples of food residues were from pottery collected from shell mounds, the food residues do not show the high δ13C and high δ15N endmember values typical for reef or marine invertebrates and just barely fall within expected values of organisms from these environments.
Samples 8 and 9, from pottery from the Juan Vega and Manchuria sites, show almost exclusive C4 plant sources, likely maize. Sample 10, one of two food residue samples from the El Cafetal site, shows an exclusively C4 signature. Sample 11, from the same site, is evenly mixed between C4 and C3 plants.
Sample 12, from a third piece of ceramic from the Alto Purruja site, shows an even split between C3 plants and C4 plants, and again no evidence reef or marine invertebrates. Sample 13, a food residue sample from the colonial church of Guadalupe, suggests a composition split between C3 and C4 plants, along with possible herbivore flesh as indicated by the high δ15N values.
DISCUSSION
The identification of carbon isotopes in the selected samples allows us to conclude a consumption of C4 plants (maize) along with legumes (beans) and other C3 plants. Archaeobotanical, ethnobotanical, and ethnographic studies in Costa Rica (Ibarra, 1990; Sánchez, 1992; Blanco & Mora, 1994; Solórzano & Quirós, 2006) indicate that C3 plant foods other than legumes at these sites can include fruits, seeds, and shoots of palms (family Arecaceae) including Acrocomia vinifera (coyol) and Bactris gasipaes (pejibaye); fruits of Hymenaea coubaril (guapinol), Persea americana (avocado), Brysonima crassifolia (nance), Spondias sp. (jobo), Brosimum sp. (ojoche), Sechium edule (chayote), and other curcurbits (family Cucurbitaceae); Theobroma cacao (cacao) and other seeds; and a variety of tubers including Manihot esculenta (yuca), Ipomea batatas (camote), and Xanthosoma sagittifolium (malanga or tiquizque).
Our findings in all cases coincide with the geographical environment of the archaeological sites, which have suitable soil and climate conditions for the cultivation of plants detected in food residues. The very high δ13C values at several of our sites indicate a high reliance on maize during the Chiriquí phase, in keeping with archaeological and paleoecological evidence of maize in the region (Horn, 2006). The suggestion by Hart et al. (2007) that charred remains in pottery are likely to underestimate maize abundance makes our high values even more noteworthy.
The cases that indicate consumption of herbivorous animal-at the sites Gamboa, Alto Purruja, and the Iglesia de Guadalupe-indicate consumption of rodents such as tepezcuintle, or perhaps rabbit or tapir. At the Guadalupe church, a colonial settlement located on a wide alluvial terrace of the General River, the habitat suggests the possibility of whitetail deer, rabbit, rodents, and tapir. Here one can also think of the possibility of cattle introduced by the Spaniards.
It is important to keep in mind that these data do not reveal all of the plant or animal consumed by people who made and used the pottery fragments we studied. Rather, our data reveal the composition of burned foods stuck on pot bottoms. Foods prepared without cooking pots are not reflected in our data. Such foods might include fish or shellfish wrapped in leaves and put on a fire, meat cooked on sticks or a rock, or tubers buried under a fire. Similarly, foods cooked in a pot that did not burn to the bottom will also not be revealed by our isotopic analysis of food residues. The selective view of past diets provided by stable isotope analyses of burned food residues probably explains why none of the three samples collected from shell mounds at the Alto Purruja site showed strong evidence of marine or reef invertebrates.
This first broad look at the isotopic composition of food residues in Costa Rican ceramics from across the southern Pacific region sets the stage for more detailed future work at individual sites in this region, and in other regions of the country from which archaeologists have recovered earthenware with burned food residues. Future work might involve comparison of food residues in different households, potentially highlighting difference in diet by social status, or over time at sites of long occupation with well-dated samples. The latter study could potentially reveal shifts in food consumption brought about by cultural changes or shifts in climate. The study of the stable isotopic signatures of charred residues in cooking pots would be enhanced by obtaining AMS 14C dates on charred residues, and by examining other potential proxies in the food residues, such as phytoliths (Staller & Thompson, 2002; Thompson, 2006), and biomarkers (Hall et al., 1990; Heron & Evershed, 1993; Evershed, 2008; Hurst, 2009; Reber & Kerr, 2012). The study of food residues on pottery would be complemented by the paired study of residues from food and beverages absorbed within pottery and those in the associated burial matrix (Reber et al., 2004; Reber & Kerr, 2012; Reber, Kerr, Whelton, & Evershed, 2018).