Costa Rica has hundreds of lakes, ranging in size from less than 1ha to over 8 000ha, and occupying diverse climate zones from sea level on both coasts to the country’s highest peaks (Horn & Haberyan, 1993, 2016; Haberyan, Horn & Umaña, 2003; Umaña, Haberyan, & Horn, 1999). These lakes have been created by volcanism, stream dynamics, faulting, landslides, glaciation, and human actions. Costa Rican and foreign researchers have been investigating lakes in Costa Rica since the 1960s, with studies focusing on the physical and chemical properties of lakes, lake biology, and evidence of lake and ecosystem history preserved in sediments (Umaña et al., 1999; Horn & Haberyan, 2016). Government agencies have carried out lake inventories in collaboration with international partners (Córdoba Muñoz, Romero Araya & Windevoxhel Lora, 1998; PREPAC, 2005) that have included some field visits. However, the limnology of many lakes remains to be characterized.
Here we describe the limnology and setting of five lakes in the southern Pacific region of Costa Rica. We collected basic limnological data from these lakes and compiled environmental and archaeological data for their surroundings as a contribution to the documentation of lake diversity in Costa Rica, and to support our investigations of biological and geochemical indicators of environmental history preserved in the sediments of these lakes.
Material and Methods
The five lakes we investigated are located in the cantón of Buenos Aires in the Valle del General in southern Pacific Costa Rica (Fig. 1). Four lakes are located south of the Río General, three (Laguna Los Mangos, Laguna Danta, and Laguna Ojo de Agua) in the Pilas district, near the settlements of Pilas and Concepción de Pilas, and one (Laguna Carse) located to the east in the Boruca district, roughly halfway between Pilas and Térraba. The fifth lake (Laguna Junquillo) is located north of the Río General, in the Volcán district, near the town of Volcán.
Lake names, where available, were taken from the 1:50,000-scale topographic maps published by the Instituto Geográfico Nacional (IGN), or from nearby communities, following PREPAC (2005); we named Laguna Junquillo after a plant common along its shore. We used both the topographic maps and a hand-held GPS to estimate lake elevations. We inferred processes of lake formation from field observations, imagery available on the Google EarthTM map server, and published literature. We compiled information on the archaeological context of the lakes from archaeological site reports and field observations, and estimated distances to lake shores using the measuring tool in the Google EarthTM server. We determined Holdridge life zone classifications using the map by Tosi (1969).
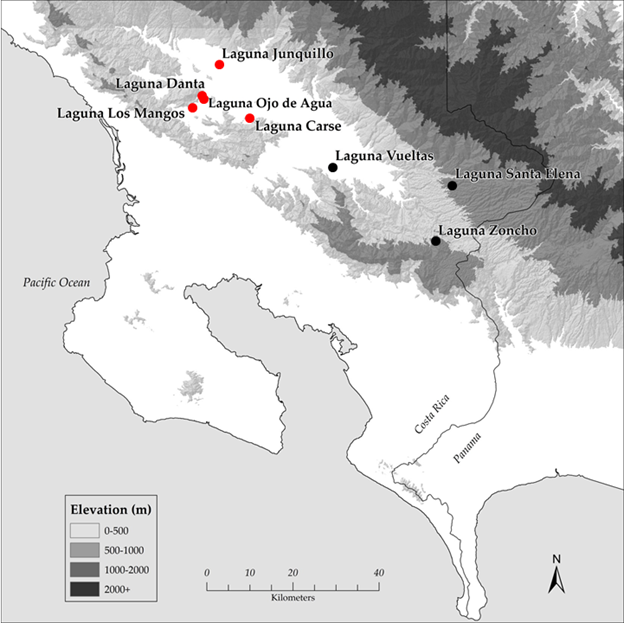
Fig. 1 Location of lake sites investigated in the southern Pacific region of Costa Rica. Red dots indicate the five lakes that are the focus of the present paper. Elevation data from CIAT-CSI SRTM (http://srtm.csi.cgiar.org).
We collected water and sediment samples and made depth and temperature soundings from small boats during visits in June 2013 and March and July 2014. We used a Speedtech portable depth sounder to measure water depths to the nearest 0.1 foot and created bathymetric maps of three of the lakes using a spline function in ArcGIS calculated from our field measurements. Lake areas and perimeters were determined using ArcGIS based on satellite and aerial imagery. We measured temperature with a field meter (YSI model 55), and transparency using a Secchi disk. Surface sediments were collected using a plastic scoop attached to a string of coring rods. From three of the lakes we also collected sediment cores 3-8 m in length using a Colinvaux-Vohnout locking-piston corer (Colinvaux, DeOliveira & Patiño, 1999). Surface water samples for chemical analyses were collected without air and analyzed for major elements and anions at the University of Wisconsin Soil and Plant Analysis Lab using ICP-OES and Ion Chromatography, respectively. We also collected whole water surface water samples to characterize phytoplankton, adding Lugol’s solution as a preservative.
We returned surface sediments in 250mL Nalgene bottles and sediment cores in their original aluminum coring tubes to the Laboratory of Paleoenvironmental Research at the University of Tennessee, where they were stored in a cold room at 4-5°C until analysis. To characterize the surface sediments of the five lakes, we carried out loss on ignition analysis following Dean (1974). Dry sediment samples were placed in pre-weighed crucibles, weighed, and ignited in a furnace for one hour at 550°C to estimate organic content based on mass loss at that temperature. Following cooling and reweighing, the crucibles with sediment were placed in the furnace at 1 000°C for one hour to estimate carbonate content based on mass loss between 550 and 1 000°C. We opened core tubes on a modified wood shaper and described and sampled sediments for radiocarbon dating and other analyses. We present basal radiocarbon dates from our cores in this paper as they are relevant to lake formation; other results will appear in subsequent papers focused on the paleoenvironmental data.
Phytoplankton samples were examined at Northwest Missouri State University using fixed slides. Samples were settled for at least four days, then the supernatant was siphoned off (Sukhanova, 1978). The remaining sample was mixed and 0,035mL was placed on a slide and covered with a cover slip. Clear nail polish was applied to the edge to delay drying. Community composition was characterized with an Olympus CH-2 microscope at 150x, and specimens were identified at 1000x. Taxonomic references used for identification included Thompson (1959), Bold and Wynne (1985), Krammer and Lange-Bertalot (1991), and Dillard (1999).
Results
Lagunas Carse, Los Mangos, Danta, and Ojo de Agua are shown on the 1976 IGN topographic map (1:50,000-scale General sheet, Hoja 3543 III, edition 2-IGNCR). These maps were based on aerial photographs that were made in 1973, and indicate that these lakes were present at that time. However, the map shows two lakes at the Ojo de Agua site, where we found only one in our field visit and on imagery available on the Google EarthTM file server. The four lakes south of the Río General range in elevation from 365-490m, and appear to owe their formation to landslides in folded and faulted Tertiary sedimentary and volcanic rocks (Table 1).
The 1976 1:50,000-scale Buenos Aires sheet (Hoja 3543 IV, edition 2-IGNCR), based on air photos taken by the IGN in 1972, shows no lake at the site of Laguna Junquillo (360m elevation). That the lake was not included on the map and has a very straight shoreline on one side suggested it was an artificial impoundment, and we encountered only a small depth of organic sediment on top of hard packed soil when we attempted to core the lake. Laguna Junquillo sits on the lower end of an alluvial fan composed of sediments eroded from the Cordillera de Talamanca (Obando & Kussmaul, 2009a, 2009b).
Table 1 Lakes and their settings
Lake | Lake No. 1 | Coordinates (WGS 84) | Elev. (m) 2 | Life Zone 3 | Geology 4 |
---|---|---|---|---|---|
Carse | 92 | 9.0669° N, 83.3472° W | 365 | Tropical moist forest, near boundary with premontane wet forest | Grifo Alto formation (Upper Miocene and Pliocene plutonic rocks and breccias) |
Los Mangos | 93 | 9.0895° N, 83.4665° W | 475 | Premontane wet forest | Curré formation comprised of Upper Miocene conglomerates, sandstones, and shale |
Danta | 91 | 9.1122° N, 83.4480° W | 470 | Premontane wet forest | Boundary between the Curré formation (Upper Miocene conglomerates, sandstones, and shale) and the Grifo Alto formation (Upper Miocene and Pliocene plutonic rocks and breccias) |
Ojo de Agua | 94 | 9.1072° N, 83.4421° W | 490 | Premontane wet forest | Same as Laguna Danta |
Junquillo | 95 | 9.1767° N, 83.4124° W | 390 | Tropical moist forest, near boundary with premontane wet forest, basal belt transition | Quaternary alluvial fan |
1Lake numbers continue the numbering system in Horn and Haberyan (2016) and correspond to numbers in a database of limnological observations from 95 Costa Rican lakes.
2Elevation based on GPS measurements made in the field and the 1:50,000 scale topographic maps published by the Instituto Geográfico Nacional.
3Life zone from the 1:750,000-scale map by Tosi (1969).
4Geology from Alvarado, Taylor, Barquero, López, Cerdas and Murillo (2009a, 2009b) and Obando and Kussmaul (2009a, 2009b).
Laguna Carse and Laguna Junquillo, the lakes at lowest elevation, both fall within the Holdridge tropical moist forest life zone as mapped by Tosi (1969); the other three lakes are located in the premontane wet forest life zone, where mean annual temperatures are expected to be slightly lower, and annual precipitation slightly higher. These life zone classifications are based on annual temperature and rainfall, not vegetation, but they provide an indication of the types of forest that would have occupied areas surrounding the lakes prior to historic or prehistoric forest clearance. Areas surrounding or adjacent to the lakes are used today for crop cultivation and cattle grazing.
More than two dozen pre-Columbian archaeological sites are located within 1-3 Km of the four natural lakes we surveyed (Fig. 2 and Tables 2, 3, 4). Because we are interested in relationships between archaeological sites and natural lakes, we have not compiled information on sites near the modern artificial impoundment of Laguna Junquillo. Many of the archaeological sites recorded currently have an undefined chronology; however, some structures and artifacts were determined to be associated with the Aguas Buenas (500 BCE-700 CE) and Chiriquí (700-1500 CE) archaeological periods. During our field visits we observed ceramic potsherds near Laguna Danta and a stone axe near Laguna Ojo de Agua. The ceramics we observed correspond to the Aguas Buenas period.
Alvarado et al. (2009a) mapped multiple scars from complex landslide events near our lake sites, and a deposit of colluvial sediment at Laguna Danta. We identified additional evidence of landslides at various scales on imagery from the Google EarthTM map server, especially at Laguna Carse and Laguna Danta (Figs. 3, 4). At Laguna Carse the different orientations of the hummocky debris visible in the images indicate multiple large slides in the vicinity of the lake. (Fig. 3).
Radiocarbon dates on plant remains in our sediment cores provide evidence of the timing of the landslides that formed the lake basins (Table 5). The deepest macrofossils we have dated in these cores provide minimum ages for the lake sediment records, and for the landslide events that formed the basins. These ages indicate lake formation prior to ca. 400 cal yr BP (calibrated years before CE 1950) at Laguna Carse, that is, before the year 1550 CE in the CE/BCE time scale. Laguna Danta formed before 630 cal yr BP (1320 CE). Laguna Los Mangos, which also appears to have formed in connection with land slumping or sliding, formed much earlier than the other lakes investigated. The lowest plant macrofossil we dated in the sediment core yielded a radiocarbon date that corresponds to 4120 cal yr BP (2170 BCE). Laguna Ojo de Agua, which we did not core, has a basin morphology and setting that also suggests formation associated with a landslide event.
Table 2 Selected pre-Columbian archaeological sites near Laguna Carse1
Site | No. in Fig. 2 | Elev (m) | Area (m 2 ) | Function | Chronology | Distance from Laguna Carse (m) |
---|---|---|---|---|---|---|
Ceibón 20 P-927 Cb-20 | 1 | 450 | 21,211 | Funerary | 700-1500 CE Chiriquí Period. | 2 600 |
Ceibón 21 P-928 Cb-21 | 2 | 339 | 361 | Petroglyph and possibly habitational | 700-1500 CE Chiriquí Period. | 1 060 |
Bijagual 1 P-915 Pg-1 | 3 | 389 | 2332 | Petroglyph | Undefined. | 710 |
Bijagual 2 P-916 Bg-2 | 4 | 505 | 1 | Petroglyph | 500 BCE-1500 CE | 440 |
Chamancragua P-920 Cg | 5 | 400 | 1 | Petroglyph | 500 BCE-1500 CE | 390 |
Bijagual 3 P-917 Bg-3 | 6 | 453 | 1 | Petroglyph | 500 BCE-1500 CE | 1 820 |
1All sites were described based on unsystematic inspection. Additional archaeological sites near Laguna Carse were registered by ICE archaeologists in the Orígenes data base of the Museo Nacional de Costa Rica (http://origenes.museocostarica.go.cr/busquedaGeneral.aspx).
Table 3 Selected pre-Columbian archaeological sites near Laguna Los Mangos1
Site | No. in Fig. 2 | Elev (m) | Area (m 2 ) | Function | Chronology | Distance from Laguna Los Mangos (m) |
---|---|---|---|---|---|---|
Fila Murciélago P-963 FM | 7 | 605 | 56077 | Domestic | Undefined | 1 100 |
Fila Silencio 01 P-964 FS-1 | 8 | 411 | 57535 | Domestic- Funerary | Undefined | 1 200 |
Fila Silencio 02 P-965 FS-2 | 9 | 447 | 1108 | Domestic | Undefined | 580 |
Fila Silencio 03 P-966 FS-3 | 10 | 367 | 181726 | Domestic- Funerary | Undefined | 900 |
La Gloria 1 P-981 LG-1 | 11 | 419 | 787 | Funerary | Undefined | 970 |
La Gloria 2 P-982 LG-2 | 12 | 447 | 5883 | Domestic | Undefined | 1 100 |
La Gloria 3, P-983 LG-3 | 13 | 379 | 1310 | Undefined | Undefined | 450 |
La Gloria 4, P-984 LG-4 | 14 | 470 | 11441 | Undefined | Undefined | 850 |
La Gloria 9, P-1430 LG-9 | 15 | 402 | 280 | Undefined | Undefined | 1 000 |
Pilas 47, P-1475 Pl-47 | 16 | 421 | 1 | Undefined | Undefined | 390 |
Pilas_34, P-1070 Pl-34 | 17 | 267 | 2174 | Domestic- Funerary | Undefined | 1 200 |
1All sites were described based on unsystematic inspection. Additional archaeological sites near Laguna Los Mangos were registered by ICE archaeologists in the Orígenes data base of the Museo Nacional de Costa Rica (http://origenes.museocostarica.go.cr/busquedaGeneral.aspx).
Table 4 Selected pre-Columbian archaeological sites near Laguna Danta and Laguna Ojo de Agua1
Site | No. in Fig. 2 | Elev (m) | Area (m 2 ) | Function | Chronology | Distance from Laguna Danta (m) | Distance from Laguna Ojo de Agua (m) |
---|---|---|---|---|---|---|---|
Danta 1 P-950 Dt-1 | 18 | 375 | 1204 | Funerary | 500 BCE -1550 CE | 2 100 | 1 950 |
Danta 2 P-951 Dt-2 | 19 | 495 | 1774 | Petroglyph and possibly habitational | Undefined | 1 010 | 1 000 |
Danta 3 P-952 Dt-3 | 20 | 512 | 5 934 | Petroglyph | Undefined | 1 150 | 1 680 |
Concepción 24 P-948 Cp-24 | 21 | 511 | 576 | Petroglyph | Undefined | 1 540 | 2 310 |
Laguna Danta P-1001 LD | 22 | 579 | 2 679 | Petroglyph | Undefined | 340 | 560 |
El Oso 1 P-957 EO-1 | 23 | 471 | 7 695 | Petroglyph | Undefined | 960 | 460 |
El Oso 2 P-958 EO-2 | 24 | 373 | 6 221 | Petroglyph | Undefined | 1 500 | 770 |
Ojo de Agua P-1033 OAg | 25 | 566 | 71 133 | Petroglyph | Undefined | 1 600 | 890 |
1Site Danta 1 was described based on a systematic investigation; all other sites were described based on unsystematic inspection. Additional archaeological sites near Laguna Danta and Laguna Ojo de Agua were registered by ICE archaeologists in the Orígenes data base of the Museo Nacional de Costa Rica (http://origenes.museocostarica.go.cr/busquedaGeneral.aspx).
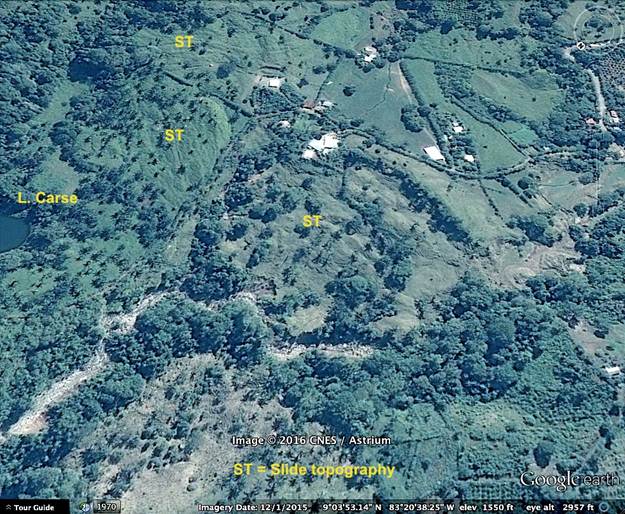
Fig. 3 Image from Google EarthTM map server showing slide topography near Laguna Carse. The conspicuously bouldery streambed of the Quebrada Moracho has picked up debris from the slides.
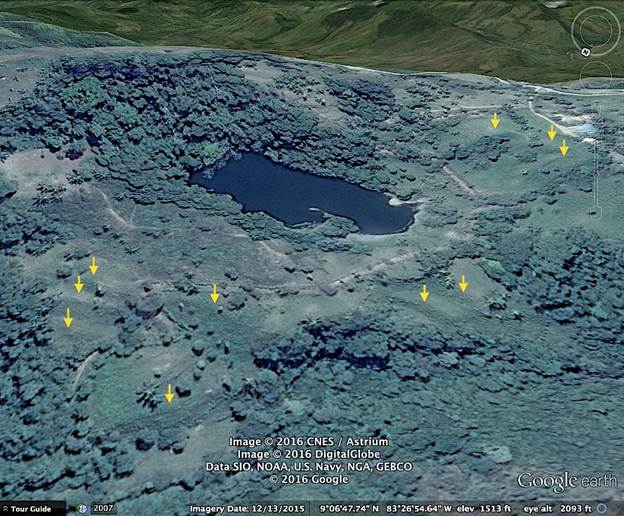
Fig. 4 Image from Google EarthTM map server showing topography suggestive of landslides near Laguna Danta.
Table 5 Lengths of sediment cores from lakes and basal radiocarbon dates
Lake | Core Length | Depth of basal 14 C date and material dated | Lab No. 1 | Uncalibrated Age ( 14 C yr BP) | Calibrated Ages 2 | ||
---|---|---|---|---|---|---|---|
95% Range (cal yr BP) | Weighted mean ages | ||||||
BP scale | BCE/CE scale | ||||||
Laguna Carse | 7.14m | 6.96m; wood | UGAMS 19460 | 350 ± 20 | 487-316 | 398 cal yr BP | 1552 CE |
Laguna Los Mangos | 7.60m | 7.34m; bark | UGAMS 19461 | 3760 ± 25 | 4 231- 3 997 | 4 122 cal yr BP | 2172 BCE |
Laguna Danta | 4.34m | 3.53m; leaf | D-AMS 014531 | 677 ± 25 | 676-563 | 629 cal yr BP | 1321 CE |
1Analyses were performed by the Center for Applied Isotope Studies at the University of Georgia (UGAMS) and by Direct AMS.
2Calibrations were made using CALIB version 7.0.2 (Stuiver & Reimer, 1993) and the IntCal 13 calibration dataset (Reimer et al., 2013). The weighted mean age was calculated from CALIB output.
Wet season water depths varied from less than 0.5 m at Laguna Los Mangos to 5 m at Laguna Danta and Laguna Carse (Table 6; Fig. 5). From our repeat photography and observations along shorelines we estimate that water levels in March 2014 were at least 0.2 m lower at Laguna Los Mangos and ca. 0.3-0.4 m lower at Laguna Danta and Laguna Junquillo than in July 2014. We do not have repeat photographs adequate to compare water levels at Laguna Carse in March 2014 with our measurements in July 2014. Water level at Laguna Danta in June 2013 was ca. 10 cm lower than in July 2014, based on comparison photographs.
We made temperature readings only in July 2014 and our results (Fig. 6) showed that none of the lakes was stratified at this time. Ojo de Agua was completely covered in floating aquatic plants (mainly Lemna), and showed the lowest temperatures and lowest transparency. Water samples from July 2014 showed that all five lakes were circum-neutral in pH (Table 7). In these lakes the most abundant elements are Ca, Na, Mg, and K, and the most abundant anions are chloride and sulfate, with sulfate levels especially high at Laguna Los Mangos and Laguna Danta. Laguna Los Mangos showed the highest alkalinity.
Table 6 Physical properties of lakes
- | Laguna Carse | Laguna Los Mangos | Laguna Danta | Laguna Ojo de Agua | Laguna Junquillo |
---|---|---|---|---|---|
Area (ha) | 1,5 | 0,3 | 1,7 | 0,2 | 0,5 |
Volume (m3) | 31 224 | 441 | 34 190 | ||
Shoreline length (m) | 463 | 208 | 699 | 163 | 309 |
Shoreline development1 | 1,07 | 1,07 | 1,51 | 1,03 | 1,23 |
Depth (m)2 | 5,0 | 0,5 | 5,0 | 2,6 | 3,5 |
Mean depth (m)3 | 2,08 | 0,15 | 2,01 | ||
Secchi depth (m) | 1,41 | >0,50 | 1,04 | 0,62 | 1,19 |
Stratification | no | no | no | no | no |
Surface temperature (°C) | 31,0 | 30,7 | 30,5 | 27,2 | 30,0 |
1Shoreline development is the ratio of the length of the shoreline to the circumference of a circle with an area equal to the area of the lake. The value provides an indication of the potential for the development of littoral plant communities. The formula is L/(2 π A) in which L is shoreline length and A is lake area in corresponding units, for example, meters and square meters.
2Depth at Laguna Danta was measured in June 2013; all other depths are from July 2014.
3Mean depth corresponds to lake volume divided by lake area.
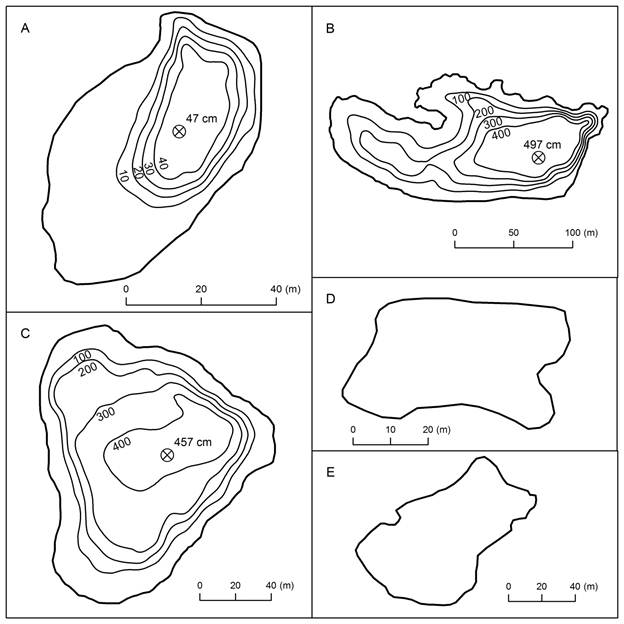
Fig. 5 Bathymetric maps of A. Laguna Los Mangos, B. Laguna Danta, and C. Laguna Carse, and outlines of D. Laguna Ojo de Agua and E. Laguna Junquillo. Depths are in centimeters. Positions and depths of sediment coring locations are shown by circles with Xs. We measured a maximum depth of 263 cm near the center of Laguna Ojo de Agua, and a maximum depth of 350cm near the southwestern end of Laguna Junquillo.
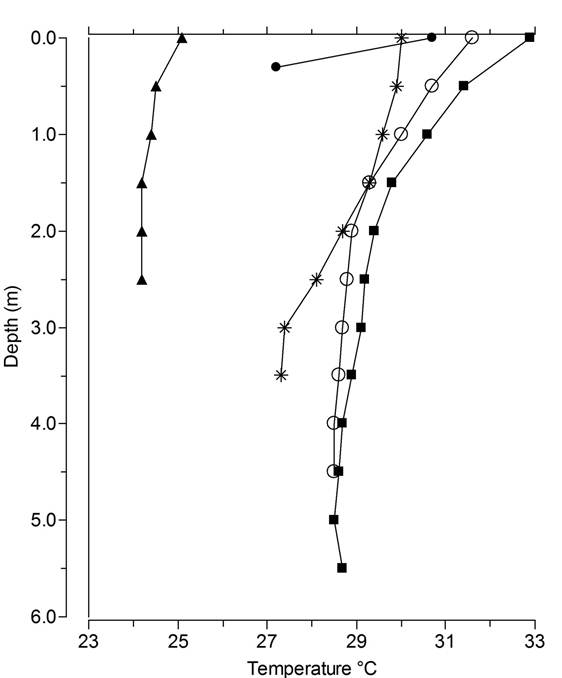
Fig. 6 Lake temperature profiles. Data were collected between July 20 and July 25, 2014 from Lagunas Ojo de Agua (solid triangle symbol), Los Mangos (solid circle), Junquillo (stars), Carse (open circles), and Danta (solid squares).
Table 7 Chemical properties of lakes
- | Laguna Carse | Laguna Los Mangos | Laguna Danta | Laguna Ojo de Agua | Laguna Junquillo |
---|---|---|---|---|---|
Cations (ppm) | - | - | - | - | - |
P | <0,05 | 0,3 | 0,2 | <0,05 | <0,05 |
K | 3,30 | 3,19 | 5,29 | 8,14 | 0,55 |
Ca | 29,93 | 65,91 | 26,70 | 24,13 | 2,26 |
Mg | 8,90 | 8,23 | 3,32 | 3,93 | 0,49 |
S | 0,46 | 4,98 | 3,72 | 0,58 | 0,12 |
Zn | <0,001 | 0,01 | <0,001 | <0,001 | <0,001 |
B | <0,02 | <0,02 | 0,04 | <0,02 | <0,02 |
Mn | < 0,001 | < 0,001 | < 0,001 | 0,06 | < 0,001 |
Fe | 0,001 | 0,004 | < 0,001 | 0,16 | 0,68 |
Cu | <0,005 | 0,03 | 0,01 | 0,02 | <0,005 |
Al | <0,05 | <0,05 | <0,05 | 0,07 | 0,05 |
Na | 6,50 | 26,70 | 24,31 | 16,15 | 2,37 |
Cd | <0,004 | <0,004 | <0,004 | <0,004 | <0,004 |
Co | <0,003 | <0,003 | <0,003 | <0,003 | <0,003 |
Cr | <0,001 | <0,001 | <0,001 | <0,001 | <0,001 |
Mo | <0,004 | <0,004 | 0,004 | <0,004 | <0,004 |
Ni | <0,003 | <0,003 | <0,003 | <0,003 | <0,003 |
Pb | <0,02 | <0,02 | <0,02 | <0,02 | <0,02 |
Li | < 0,001 | 0,002 | 0,002 | 0,002 | < 0,001 |
As | <0,03 | <0,03 | <0,03 | <0,03 | <0,03 |
Se | <0,03 | <0,03 | <0,03 | <0,03 | <0,03 |
Ba | 0,05 | 0,04 | 0,01 | 0,07 | 0,01 |
Anions (ppm) | |||||
F- | 0,45 | <0,01 | <0,01 | <0,01 | <0,01 |
Cl- | 4,63 | 3,00 | 3,47 | 1,72 | 1,71 |
NO2 - | <0,01 | <0,01 | <0,01 | <0,01 | <0,01 |
Br- | <0,01 | <0,01 | <0,01 | <0,01 | <0,01 |
NO3 - | 2,07 | 1,63 | <0,01 | <0,01 | <0,01 |
PO4 3- | <0,02 | <0,02 | <0,02 | <0,02 | <0,02 |
SO4 2- | 1,08 | 10,09 | 10,60 | 0,32 | <0,02 |
- | |||||
Alkalinity(ppm CaCO3) | 118,8 | 212,5 | 112,5 | 125,0 | 12,5 |
pH | 7,4 | 7,3 | 7,8 | 7,1 | 7,3 |
Our analysis of phytoplankton in whole water samples revealed diversity in composition across the five lakes (Table 8, Fig. 7). The single taxon estimated to have the highest biomass differed at each site, but there was overlap among the top three taxa, which were identical for Laguna Danta and Laguna Carse (Peridinium, Trachelomonas, Cryptomonas).
Table 8 Phytoplankton in lakewater samples*
Taxon | Laguna Los Mangos | Laguna Danta | Laguna Ojo de Agua | Laguna Carse | Laguna Junquillo |
---|---|---|---|---|---|
Cryptophyta | - | - | - | - | - |
Cryptomonas | - | 3 | - | 3 | 2 |
Diatoms | - | - | - | - | - |
Pinnularia | + | - | - | - | - |
Gomphonema | + | - | - | - | - |
Nitzschia | + | - | - | - | - |
Naviculaceae | - | - | + | - | - |
Aulacoseira | + | - | - | - | - |
Dinoflagellates | - | - | - | - | - |
Peridinium | - | 1 | - | 2 | - |
Chlorophytes | - | - | - | - | - |
Closterium | + | - | - | - | |
Desmidaceae | - | - | - | - | 3 |
Pediastrum obtusum | - | + | - | - | - |
Ankistrodesmus | - | - | - | - | 1 |
Scenedesmus | - | + | - | - | - |
Protozoa | - | - | - | - | - |
Trachelomonas | - | 2 | - | 1 | - |
Phacus | 1 | - | 3 | - | - |
Cyanobacteria | - | - | - | - | - |
Aphanizomenon | 3 | - | - | - | - |
Merismopedia | - | - | 1 | - | - |
Oscillatoria | 2 | - | 2 | - | - |
*Numbers 1, 2, and 3 indicate relative biomass for the three most abundant taxa in each lake.
+ denotes taxa also observed in samples.
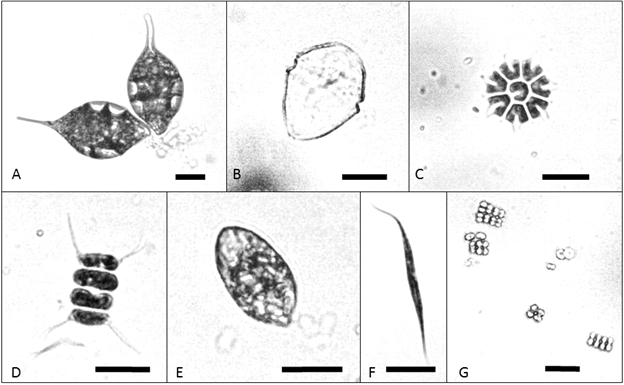
Fig. 7 Selected plankton at study sites: Phacus (A) in sample from Laguna Los Mangos; Peridinium (B), Pediastrum obtusum (C), Scenedesmus (D), and Cryptomonas (E) from Laguna Danta; Ankistrodemis (F) from Laguna Junquillo; and Merismopedia (G) from Laguna Ojo de Agua. All scale bars are 10 micrometers.
Loss on ignition analysis revealed similar organic matter content in the surface lake sediments (range 22,1-28,8%, mean 24,7%, calculated on a dry mass basis), with highest values at Laguna Danta (Fig. 8). Estimated carbonate values were low and also similar, ranging from 2,0-4,3% (mean 2,8%).
Discussion
Our original intent was to sample only natural lakes in the cantón of Buenos Aires, to build on the lake-sediment studies of Holocene environmental history that we have carried out at other lakes in the region (Lagunas Vueltas, Zoncho, and Santa Elena: Fig. 1; and Horn & Haberyan (2016) and references therein). We were introduced to Laguna Junquillo by a local researcher, who understood the lake to be natural and referred to it as Laguna Tadeo. We subsequently discovered Laguna Tadeo to be the name associated with a seasonal lake shown on the IGN Buenos Aires topographic map approximately 1 Km southeast of Laguna Junquillo. Both sites are on the Río Cañas alluvial fan, interpreted to have formed following deglaciation of the Cordillera de Talamanca (Obando & Kussmaul, 2009a), which occurred between 12,360 and 9700 cal yr BP based on radiocarbon dates on basal sediments in glacial lakes on Cerro Chirripó (Orvis & Horn, 2000). We were unable to visit the site of the real Laguna Tadeo during our field work at area lakes, but imagery on the Google EarthTM server suggests the lake may still exist. If so, it may preserve sediments useful for studying past climates and environments, possibly for a significant period of time, if the lake is as old as the fan on which it sits.
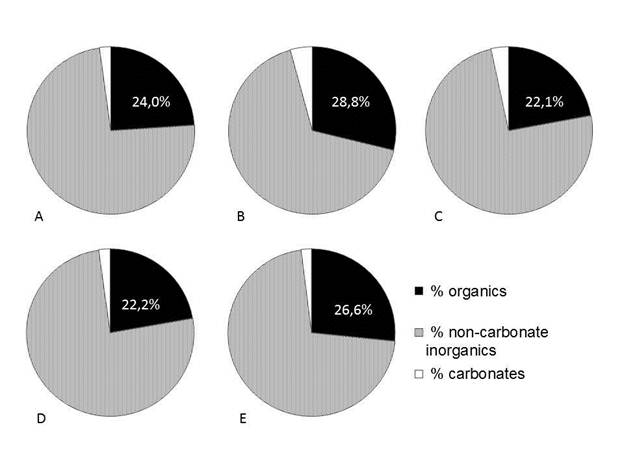
Fig. 8 Composition of surface lake sediment samples based on loss on ignition analysis. Percentages expressed on a dry mass basis. A. Laguna Los Mangos. B. Laguna Danta. C. Laguna Ojo de Agua. D. Laguna Carse. E. Laguna Junquillo.
Our interpretation that landslides created the four natural lakes we investigated is in keeping with local geomorphology and geology (Alvarado et al., 2009a, 2009b) and with the formation of other lakes we have investigated in the region. Sitchler, Fisher, Gardner and Protti (2007) tied the existence of very large landslides in the southern Pacific region to deformation of the Fila Costeña caused by the subduction of the Cocos Plate. Repeated landslides in the watershed of Laguna Carse may have contributed to the anomalously rapid infilling of the lake by disturbing forests and exposing mineral soil. Based on the age of the wood we radiocarbon-dated near the base of our sediment core, Laguna Carse has accumulated 7m of sediment since it formed near the time of the Spanish Conquest, indicating an average accumulation rate of ca. 1,4cm/yr. This rate is far higher than those we have found in dozens of cores from widespread locations in Costa Rica. Infilling was also rapid at Laguna Santa Elena, a much smaller lake (0,13ha) 50Km east southeast of Laguna Carse (Horn & Haberyan, 2016). A sediment core from Laguna Santa Elena revealed the position of the Spanish Conquest to be at a sediment depth of ca. 2,7m (Anchukaitis & Horn, 2005), indicating a post-Conquest sedimentation rate of 0,6 cm/year, which is less than half the rate at Laguna Carse. Multiple cores from Laguna Zoncho (50Km southeast of Laguna Carse; Clement & Horn, 2001; Taylor, Horn & Finkelstein, 2015) indicate an average post-Conquest sedimentation rate of ca. 0,2 cm/yr. The extreme rate of sediment accumulation in Laguna Carse, which was 2,5-7 times faster than at other lakes in the southern Pacific region of Costa Rica, likely also reflects post-Conquest deforestation and agricultural use of the lake basin, including by indigenous Terraba people who were relocated to the vicinity of Laguna Carse by the Spanish in the late 17th century (Stone, 1962).
Our compilation and examination of archaeological site reports confirms the presence of archaeological structures, petroglyphs, and funerary and habitational sites near the four natural lakes. Archaeological evidence predates the formation of Laguna Danta during the latter part of the Chiriquí archaeological period, and of Laguna Carse near the time of the Spanish Conquest. When these lakes formed they would have become resources for people already living nearby, and perhaps some people moved closer to the new lakes in response to the availability of this resource. But these lakes formed on a landscape already inhabited by people, as shown by the ages of artifacts and sites near the lakes. At Laguna Los Mangos, however, the weighted mean age of 4122 cal yr BP (2172 BCE) for a piece of tree bark near the base of our 7,6m long sediment core exceeds the age of the earliest known archaeological sites in the region. In this case, the lake formed prior to human presence in the area, allowing us to infer the timing and nature of initial prehistoric human settlement and land-use through the study of preserved pollen grains, charcoal fragments, stable isotope signatures, and other indicators in the lake sediments, as we have done at other sites in Costa Rica (Horn & Haberyan, 2016).
Three of the natural lakes we sampled, Lagunas Danta, Ojo de Agua, and Carse, were mentioned in a 2005 inventory of water bodies in Costa Rica that emphasized the development of fishing and aquaculture (PREPAC, 2005), and in an earlier compilation by government agencies (Córdoba Muñoz et al., 1998). These sources list the area of Lagunas Danta and Ojo de Agua as 5 and 0,5ha, respectively, values two to three times higher than we determined in our analysis using ArcGIS, but the area they list for Carse (1,5ha) matches our determination. While the PREPAC inventory team visited both lakes, they did not collect limnological data or samples. The earlier lake inventory (Córdoba Muñoz et al., 1998) seems to have included very little field work, but did list a depth for Laguna Danta of 3m. The source of this datum is not clear; we measured a maximum depth of 5m in June 2013 and at least 4.7m in March 2014. Whether the depth of 3m represents an actual measurement or an estimate based on conversations with locals is unknown. Citing the 1998 report, PREPAC (2005) also reported 3m as the depth of Laguna Danta, but presented the value as a mean depth. This is a typographical error because in limnology mean depth is not an observation, but a variable calculated by dividing the volume of a lake by its surface area. From our GIS and bathymetric analyses we calculate the mean depth at Laguna Danta to be 2m (Table 5).
During their visit to Laguna Ojo de Agua during August-October 2004, the PREPAC team found the lake surface to be fully covered by ‘lechuguilla de agua.’ This common name is sometimes used for the aquatic plant Pistia stratioites, but more typically Pistia stratioites is called ‘lechuga de agua’. Pistia has much bigger leaves than Lemna and the photograph from 2005 suggests a smaller-leaved plant. The dominant plant in 2005 may have been Lemna, as it was in 2014.
Lake water temperatures at the five sites are in keeping with observations at lakes throughout Costa Rica (Horn & Haberyan, 1993, 2016; Umaña et al., 1999; Haberyan et al., 2003). All five of these lakes are small, and are probably polymictic lakes that turn over frequently. The water chemistry data are also similar to previous surveys. These five lakes in the Buenos Aires cantón showed undetectable concentrations of many elements, providing benchmarks to assess any future changes in lake chemistry due to changes in forest cover, land use, human population density, air pollution, or climate change.
Phytoplankton samples from the five lakes included genera that were previously reported in other shallow, low-altitude Costa Rican lakes (Haberyan, Umaña, Collado, & Horn, 1995), but not as dominant genera. For example, we found Phacus to dominate Laguna Los Mangos (Table 8), but in the wet-season survey of 30 lakes by Haberyan et al. (1995) this genus was uncommon in the four lakes in which it occurred, ranking as the fifth most dominant taxon in only one lake. Similarly, Ankistrodesmus was a widespread, subdominant (rank 2-4) genus in the earlier survey, but we found it to dominate Laguna Junquillo. With the notable exception of the detailed weekly sampling of phytoplankton at several lakes by Umaña (2010, 2014, 2016), most sampling of phytoplankton in Costa Rican lakes has been episodic or limited to a single visit, hampering understanding of phytoplankton ecology. Sampling at higher frequency and across multiple seasons at more lakes will be required to document relationships between phytoplankton and lake characteristics across climate and ecological zones of Costa Rica.
Our loss on ignition analyses showed low apparent carbonate content in the surface sediments of the five lakes, an expectation where carbonate bedrock is rare or absent. The low values we found may have been affected by clay minerals in the sediments. Dean (1974) noted that most clays contain up to 5% lattice water, which is driven off between 550°C and 1 000°C. Loss on ignition analysis of sediment samples that are high in clay can show 3-4% mass loss between 550°C and 1 000°C, even if no carbonate is present. X-Ray diffraction and other geochemical methods could provide more accurate measurements of the carbonate content of the inorganic sediments, but from our loss on ignition data we know that values are low.
The organic matter content of the surface sediments of the five lakes falls near the middle of the range of a set of 57 Costa Rican lakes (Fig. 9). The amount of organic matter in surface lake sediments is determined by many factors. These include the productivity of aquatic organisms and littoral plants; the input of organic matter produced from outside of the lake; and inorganic inputs, which dilute organic matter percentages in sediments (Shuman, 2003). Organic productivity and mineral input are affected by soils and climate. Natural disturbances such as landslides and wildfires, and human actions such as forest clearance, the establishment and maintenance of agriculture fields, and the creation of footpaths, house yards, or other features with hard-packed surfaces can increase soil erosion and transport of mineral sediments to lakes by exposing mineral soil, reducing soil infiltration capacity, and enhancing surface runoff (Harden, 1992; Wallin & Harden, 1996).
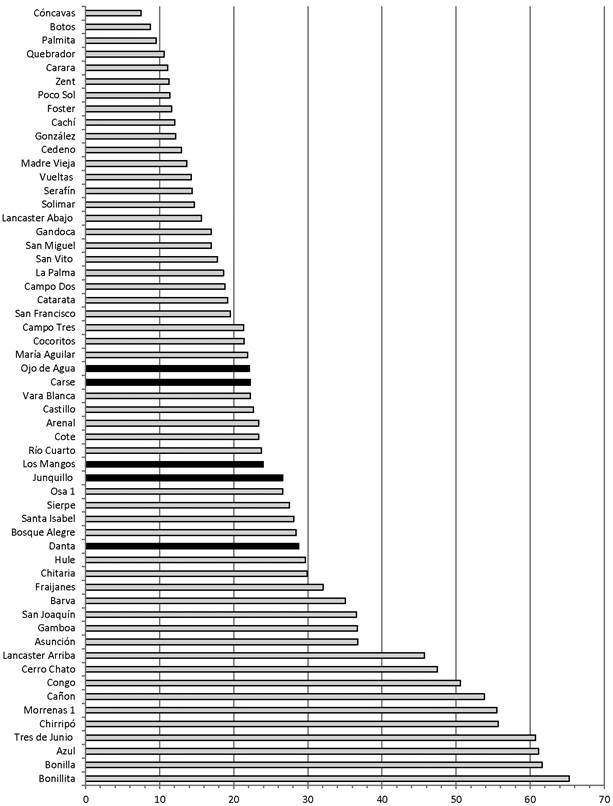
Fig. 9 Organic matter content (percent dry mass) of surface sediments of the five lakes (black bars) compared to other lakes in Costa Rica (light gray bars). Data are from Rodgers and Horn (1996) and Horn (unpublished).
Other processes that can influence sediment organic content include decomposition of organic matter and sediment focusing, a limnological process that can lead to increased rates of sediment accumulation in central portions of lake basins, potentially altering sediment composition (Shuman, 2003). Because multiple watershed characteristics and processes within and outside of lakes influence the organic content of sediments, it is possible for two lakes with similar sediment composition to have arrived at that condition in different ways. Lake A may have much higher aquatic productivity than Lake B, but similar sediment composition if Lake A also exceeds Lake B in the input of mineral matter from surface runoff and inflowing streams.
Without additional study it is premature to read too much into the central position of these new five lakes in the larger dataset of organic matter content shown in Figure 9. However, as a broad generalization, we can conclude that the present balance between fluxes and preservation of organic and inorganic matter is somewhat similar across the five lakes, perhaps driven by the similar climate and land use at the sites. The geomorphic setting of the four natural lakes, each associated with one or more landslides, is also similar. For the three lakes from which we have sediment cores, marked variations in loss on ignition downcore show that these balances have changed over time, leading to intervals in which accumulating sediments have organic contents much higher or lower than surface values. These changes were likely influenced by changes in climate, natural disturbance regimes, human activity, and lake levels over the histories of the lakes (Shuman, 2003).
Our investigation of the limnology and setting of four natural lakes and one artificial impoundment in southern Pacific Costa Rica adds to knowledge of lake characteristics and diversity in Costa Rica. Our observations provide context for our analyses of sediment records from these lakes. Lakes and wetlands in Costa Rica are an important resource for humans and wildlife, and contribute to overall biodiversity across all ecosystem regions. Our study provides benchmarks for assessing future changes in these lakes and their watersheds resulting from human activity, natural disturbances, and regional and global climate change.