Introduction
Animal feed has a direct impact on human nutrition; better quality animal feed means better quality human food too (Téllez et al., 2015). Indeed, there is a strong correlation between the quality of livestock feed and the quality of animal products offered for human consumption (Simeanu & Radu-Rusu, 2023). The livestock feed industry continuously seeks to develop strategies that utilize agri-food by-products to address nutrient deficiencies (such as certain vitamins and minerals) and enhance the physiological and antioxidant potential of food products (Lee et al., 2017). The aim is to formulate feed supplements that rebalance nutrient requirements and improve animal performance (Nath et al., 2023).
In Algeria, carob trees are abundant and widely distributed along the Tell region, offering significant potential for carob production. However, a portion of this potential is lost due to harvesting difficulties, as carob often grows in rugged terrain (Boublenza et al., 2019).
Algeria was classified ninth in rank as a carob producer in the world by its average production of 3577 t per year (The Statistics division of the Food and Agriculture Organization of the United Nations, 2021). Carob beans are rich in carbohydrates, particularly hydrolysable sugars and fiber, making them an excellent energy source (Lagha-Benamrouche et al., 2023). Orange peels contain bioactive compounds with high antioxidant potential, such as carotenoids, phenolic compounds and vitamin C (Lagha-Benamrouche et al., 2018). Hen eggshells are inexpensive and abundant waste products, primarily composed of mineral elements, particularly calcium carbonate in the form of crystallized calcite (Neunzehn et al., 2015).
Motivated by the availability and benefits of these raw materials, this study aimed to characterize food waste (carob molasses residue, carob seeds, bitter orange peels and brown chicken eggshells) with a view to their use as a value-added food supplement for animals based on their biologically active compounds.
Materials and methods
Sampling and preparation of biological material
The biological material used in this study included category “A” brown chicken eggshells, belonging to the industrial farming method (battery farming) supplied from the Boumerdès market, bitter orange peel (Citrus aurantium L. cv. Bigarade), and carob molasses residue (Ceratonia siliqua L. cv. Bouzegza). These plant materials were collected in Boumerdès, a region located in northeastern Algeria characterized by fertile soil and a Mediterranean climate, with relatively cold, rainy winters and hot, dry summers. Harvesting was conducted randomly from several trees of the same variety in August 2021 for carob, and in December 2021 for Bigarade, based on the ripening stage of each species.
Upon collection, the fruits were washed in tap water (drinkable water). The peels were then cut and dried in an oven at 40 ºC for one week, following the method described by Lagha-Benamrouche and Madani (2013). The dried plant materials were ground to a particle size smaller than 500 µm, and the resulting powder was stored in sealed glass jars, labeled, and kept away from light.
To obtain carob molasses residue, 500 g of cleaned carob pods, with seeds removed, were soaked in one liter of water overnight. The mixture was then cooked for 2 to 3 hours with continuous stirring. After filtration through muslin, the filtrate was concentrated over low heat to obtain molasses. The residue from filtration was air-dried, ground, sieved, and stored for subsequent analysis. The eggshells were rinsed with water and then dried rapidly in an oven at 40 °C.
Determination of chemical composition and overall energy value
Total sugars were determined following the method described by Lagha-Benamrouche et al. (2018). In this method, 1 g of calcium carbonate was mixed with 20 ml of sample diluted at a ratio of 1:100 and 20 ml of water. After shaking and allowing it to stand for 15 minutes, 1 ml of 15 % potassium ferrocyanide and 1 ml of 30 % zinc acetate were added. The solution was then diluted to 100 ml with distilled water and filtered.
To determine reducing sugars, 5 ml of diluted Fehling’s solution was added to a test tube and brought to a boil for 3 minutes. The defecated and diluted sample was then added drop by drop until the blue coloration disappeared, without the appearance of yellow coloration.
For non-reducing sugars determination, the solution underwent hydrolysis in an acid medium at high temperature. Specifically, 5 ml of hydrochloric acid were added to 10 ml of the solution, followed by heating in a boiling water bath for 15 minutes. After cooling, the solution was neutralized with sodium hydroxide to pH 6. A volume of this solution was then taken, and the procedure for determining reducing sugars was followed. The total sugars were determined by hydrolysis, and the level of non-reducing sugars was deduced by subtracting the reducing sugars from the total sugars (Chidan Kumar et al., 2014).
Crude protein content was determined using the Kjeldahl method described by Jean Constant et al. (2020). This method involves mineralization, distillation, and sulfuric acid titration phases. The total protein content is expressed as a percentage by mass using specific equations (equations 1 and 2).


Where,
V0: Volume (mL) of sulphuric acid solution (0.1 N) added for the blank test.
V1: Volume (mL) of sulphuric acid solution (0.1 N) added for the test (sample).
N: Normality of the sulphuric acid solution.
Me: Mass (g) of sample.
6.25: Conversion factor.
Fat extraction was conducted using hexane as the organic solvent with a Soxhlet apparatus, following the method outlined by Jean Constant et al. (2020). Initially, 5 g of powder were accurately weighed and placed into a tared Whatman cartridge. A pre-weighed extraction flask was then filled with 60 mL of hexane (M1). The flask, containing the solvent, was mounted on the heating cap of the Soxhlet apparatus, set at 110 °C, and left for 6 hours. Subsequently, the flask was removed from the Soxhlet apparatus and placed in an oven at 60 °C for 1 hour to evaporate all the solvent.
After complete evaporation, the flask was reweighed (M2). The fat content was calculated using the equation 3.

The energy value is calculated using the thermal coefficients of Atwater, with 4 kcal for 1 g of protein, 9 kcal for 1 g of lipids, and 4 kcal for 1 g of carbohydrates. The overall energy value (E) is determined by the following relationship (equation 4) (Lagha-Benamrouche et al., 2023).

Where,
E: total energy value in kcal.
L: total lipid content in g per 100 g sample
C: total carbohydrate content in g per 100 g sample.
P: total protein content in g per 100 g sample.
Determination of ash and mineral content
The ash content is determined by subjecting the sample to high temperatures (550 °C for 3 to 5 hours), resulting in the destruction of all organic substances and the formation of a white or gray-white powder (Lagha-Benamrouche et al., 2018).
The mineral content was assessed using an atomic absorption spectrophotometer following the method described by Guirrou et al. (2022). Initially, 0.1 g of ash obtained after mineralization of the sample was dissolved in 6 mL of nitric acid (65 % v/v). The resulting mineralisate was transferred to a volumetric flask and diluted to 50 mL with demineralized water. Standard solutions of each mineral (Mn, Mg, Fe, Cu, Zn, K, P, Ca, Na, Cd, Pb) at a concentration of 100 mg/L were prepared to calibrate the flame atomic absorption spectrophotometer. Subsequently, 2 mL of a 3 % lanthanum solution was added to a 5 mL solution of the mineralisate in a 50 mL volumetric flask, and the volume was adjusted with nitric acid. The minerals were quantified using an atomic absorption spectrophotometer (SPECTRAA-5, VARIAN) at specific wavelengths, with quantification based on external calibration using the standard solution (100 mg/mL) of each mineral (Guirrou et al., 2022).
Determination of bioactive compounds
Ascorbic acid extraction was performed following the method outlined by Lagha-Benamrouche et al. (2018). Briefly, 1 g of orange peel was mixed with 15 ml of an acetonitrile/acetic acid/water mixture (75:2:33 v/v). The mixture was homogenized and then incubated for 10 minutes in the dark at room temperature. Subsequently, centrifugation was carried out at 9,000 rpm for 10 minutes at 4 °C. The resulting supernatant was adjusted to a volume of 25 ml with the extraction solvent, filtered through a 0.45 µm pore size membrane, and analyzed immediately. Vitamin C content was determined using high-performance liquid chromatography (HPLC) with DAD detection. The HPLC system (Series LC-10A HPLC, Shimadzu, Kyoto, Japan) was equipped with a UV/Visible detector (SPD-20A) set to 210 nm, and an Aminex HPX-87 H column (300 mm × 7.8 mm, Bio-Rad), maintained at 55 °C. The eluent comprised sulfuric acid 0.045 N-acetonitrile (6 % v/v) with a flow rate of 0.3 ml/min. The chromatographic peak corresponding to vitamin C was identified by comparing the retention time with that of the reference standard. A calibration curve was constructed using ascorbic acid at concentrations ranging from 0.5 µg/ml to 200 µg/ml (Borba et al., 2020).
Carotenoid content determination followed the method described by Lagha-Benamrouche and Benkaci (2022). Briefly, 1 g of sample was homogenized for 1 minute with 3 ml of the extraction solvent mixture (hexane/acetone/ethanol, 8:7:7). Then, 200 µl of potassium hydroxide (1 M prepared in methanol) was added, and the mixture was vigorously shaken. After incubation for 16 hours, 3 ml of hexane was added, and a second extraction was performed for 1 minute. Subsequently, 3 ml of Na2SO4 (1 %) was added, and the mixture was stirred for 1 hour. The upper phase was recovered and protected from light with aluminum foil. Carotenoid content was determined by measuring the absorbance of the hexane extract at 470 nm. Carotenoid concentrations were estimated by reference to a calibration curve obtained using ß-carotene as the standard.
Extraction of phenolic compounds was conducted according to the method outlined by Lagha-Benamrouche (2022). Specifically, 3 g of dried powder were extracted with 30 ml of methanol-water (80 %) at room temperature for 22 hours using a magnetic blender. The extract was then vacuum-filtered, and the residue was re-extracted with 30 ml of acetone-water (70 %). The resulting filtrates were combined, centrifuged at 3060 g for 5 minutes, and vacuum-filtered using Whatman No. 1 paper. The obtained aqueous organic extract was concentrated under reduced pressure at 40 °C using a rotary evaporator until complete evaporation and then reconstituted in pure methanol. The total phenolic compound content in the extract was determined using the Folin-Ciocalteu reagent and gallic acid as a standard, following the procedure described by Lagha-Benamrouche & Madani (2013). Specifically, 100 µL of extract was mixed with 750 µL of sodium carbonate (7.5 %) and 750 µL of Folin-Ciocalteu reagent (diluted 1:10). After 2 hours of incubation at room temperature in the dark, absorbances were measured at 750 nm. The calibration curve was prepared in the same manner as the extract, using gallic acid as the standard.
Determination of fiber content
To determine the different fiber contents, the dietary fiber analyzer (FIBERTEC™8000: FOSS) was employed. The samples underwent treatment with various solvents. The undissolved residue was dried, weighed, and then incinerated. The mass loss observed during combustion corresponded to the crude fiber, Acid Detergent Fiber (ADF), Neutral Detergent Fiber (NDF), or Acid Detergent Lignin (ADL) content of the sample. The total fiber content was determined using the Weende method. The determination of crude fiber content can be outlined in 5 steps: the sample is boiled in 1.25 % sulfuric acid to extract the sugar and starch. The residues in the crucibles are washed with water to eliminate acid residues and neutralize the pH. The second digestion involves alkaline digestion with 1.25 % sodium or potassium hydroxide, which removes the proteins and some of the hemicellulose and lignin. The final stage is the defatting of the samples, where the final washes are conducted with acetone (Debnath et al., 2022).
Regarding NDF, ADF, and ADL, estimation was performed following the Van Soest protocol. The portion of substance that remains undissolved in an acidic detergent solution is referred to as ADF. The ADF content measures the proportion of cellulose, lignin, and N-lignin compounds. NDF pertains to the proportion of substances that cannot be dissolved with a neutral detergent solution. This NDF content provides information about the content of cellulose, hemicellulose, lignin, and N-lignin compounds. To determine ADL, the ADF method is employed as a pretreatment. The cellulose and lignin components are not removed from the ADF solution. The cellulose is then dissolved with 72 % sulfuric acid to obtain the crude lignin (ADL) (Debnath et al., 2022). The NDF, ADF, and ADL solutions utilized in the experimental protocol are commercially prepared and ready-to-use solutions.
Pectin was determined according to Hasem et al. (2019). 100 g of sample were stirred into a 900 mL hydrochloric acid aqueous solution adjusted to the desired pH of 2.5. Subsequently, the solutions were extracted at 85 °C for 60 minutes. The slurries were filtered through cheesecloth and allowed to cool to room temperature at 25 °C. Acidified ethanol, comprising 4 % hydrochloric acid added to 95 % ethanol in the ratio of 1:4 (v/v), was then incubated for 60 minutes at 25 °C. The mixture underwent centrifugation at medium speed for 15 minutes using a benchtop centrifuge (Kubota 5100, Fujioka, Japan). Afterward, the solutions were rewashed twice with 95 % ethanol (1:2, v/v) and centrifuged for 15 minutes. The precipitate was collected and dried in a hot-air oven until a constant weight was achieved. The percentage of pectin yield collected was calculated using the equation 5.

The extraction of the gums was conducted following the protocol outlined by Lagha-Benamrouche et al. (2023). 100 g of seeds were macerated in a 60 ml H2SO4/H2O solution with a ratio of 60/40 v/v at 60 °C for 1 hour. This acid treatment at an elevated temperature carbonized the hull, which was subsequently removed through extensive washing and rubbing in water, and passing through a metallic sieve with a 2 mm mesh size. The dehusked seeds were then dried at 100 °C for 30 minutes and briefly crushed (10 seeds dehusked/3s) using a laboratory mill (model MF 10, IKA, Staufen, Germany) to separate the two endosperms (albumen) and release the germ, which detached in pieces due to its friability. The germ fractions were sifted off from the unbroken endosperm halves using a 2 mm sieve. The pieces of endosperm were then ground and sifted with a 0.125 mm sieve to produce flour, resulting from the acid dehulling pre-treatment. The yield was determined using the following equation 6.

Statistical analysis
Experiments were conducted in three parallel measurements, and results were expressed as mean ± standard deviation. Statistical analysis was carried out using STATISTICA 5.5 software. The ANOVA test (one-factor variance) was used to establish the differences in the means of the variables and to determine the significance of the variables at the p ≤ 0.05 threshold. When a significant difference was observed, multiple Tukey comparison tests were performed to determine homogeneous groups, and the significant difference was indicated using subscription letters (a, b, c, d).
Results
Chemical composition of the by-products studied and energy value
The content of phytochemical compounds varied significantly among the samples (p ≤ 0.05) (Table 1). The by-products were found to be low in fat and protein but moderately high in sugars. Protein contents were ranked in the following descending order: Carob seed > Carob Molasses Residue > Eggshells > Bigarade peels. The highest sugar content was observed in carob seeds, which was twice as high as that of carob molasses residue and Bigarade peels. Regarding fat content, carob seeds exhibited the highest level, being 30 times higher than that of carob molasses residue and 17 times higher than that of Bigarade peels. The overall energy value of the supplement was estimated at 478.19 kcal/100 g, with carob seeds contributing to 70 % of the overall energy value.
Table 1 Chemical compound content and estimated energy value of the studied by-products (carob molasses residues and carob seeds (Ceratonia siliqua L. cv. Bouzegza), eggshells, and bitter orange peels (Citrus aurantium L. cv. Bigarade)) collected in Boumerdés, Algeria, 2021.
CMR | Carob seed | Eggshells | Bigarade peels | |
Total sugars (g GE/100 g FM) | 12.81b | 28.08a | Nd | 11.04c |
Reducing sugars (g/100 g FM) | 10.15a | 07.35b | Nd | 2.42c |
Non-reducing sugars (g/100 g FM) | 2.5c | 14.76a | Nd | 4.92b |
Proteins (g BSA E/100 g FM) | 6.46b | 29.56a | 2.81c | 1.66d |
Fat (g/100 g FM) | 0.38c | 11.04a | Nd | 0.64b |
Overall energy value (kcal/100 g FM) | 80.50b | 329.92a | 11.24d | 56.57c |
Total energy value of the supplement (kcal/100 g FM) | 478.19 |
BSA E: Bovine serum albumin equivalent. GE: Glucose equivalent. FM: Fresh matter. Nd: Not determined. CMR: Carob molasses residue. / BSA E: Equivalente de albúmina de suero bovino. GE: Equivalente de glucosa. FM: Materia fresca. Nd: No determinado. CMR: Residuo de melaza de algarroba.
Values with the same letter in each row indicate no significant difference (p > 0.05). The results are listed in descending order: a > b > c > d. / Valores con la misma letra en cada fila no muestran diferencias significativas (p > 0,05). Los resultados se presentan en orden descendente: a > b > c > d.
Mineral composition of the by-products studied
The ash contents varied significantly among the samples (p ≤ 0.05), with the highest rate observed in eggshells. The mineralogical study revealed the predominance of calcium, magnesium, and phosphorus in eggshells. Carob residues exhibited dominance in calcium, magnesium, sodium, and potassium. Bigarade peel was characterized by its richness in copper, potassium, calcium, magnesium, and phosphorus (Table 2).
Table 2 Ash and mineral content of the studied by-products (carob molasses residues and carob seeds (Ceratonia siliqua L. cv. Bouzegza), eggshells, and bitter orange peels (Citrus aurantium L. cv. Bigarade)) collected in Boumerdès, Algeria, 2021.
CMR | Carob seed | Eggshells | Bigarade peels | |
Ash (g/100 g FM) | 0.78d | 6.65b | 95.37a | 2.46c |
Minerals | (µg/g) | (mg/g) | (mg/g) | (µg/g) |
Manganese (Mn) | 3.93a | 0.06 c | Nd | 0.15b |
Iron (Fe) | 6.54a | 0.09 c | Nd | 0.53b |
Cadmium (Cd) | Nd | Nd | Nd | Nd |
Lead (Pb) | Nd | Nd | Nd | Nd |
Calcium (Ca) | 1733±16c | 10.16b | 374a | 400.41d |
Copper (Cu) | Nd | Nd | Nd | 2700 |
Magnesium (Mg) | 230c | 2.89b | 4a | 230c |
Sodium (Na) | 396b | 2.01a | Nd | 10.49c |
Zinc (Zn) | 2.68 c | 0.06 a | Nd | 6.17b |
Phosphorus (P) | 820b | 0.07d | 3.82a | 189.01c |
Potassium (K) | 1040b | 0.97c | Nd | 1540a |
RMC: Carob molasses residue. Nd: Not determined. / RMC: Residuo de melaza de algarroba. Nd: No determinado.
Values with the same letter in each row indicate no significant difference (p > 0.05). The results are listed in descending order: a > b > c > d. / Los valores con la misma letra en cada fila no muestran diferencias significativas (p > 0,05). Los resultados se presentan en orden descendente: a > b > c > d.
Bioactive compound content
The content of bioactive compounds (vitamin C, carotenoids, and polyphenols) varied significantly between samples (p ≤ 0.05) (Table 3). Vitamin C was only determined in the peel of Bigarade, and at a notably high level. The carotenoid content of Bigarade greatly exceeds that of other by-products. Bitter orange peels constitute a reservoir of polyphenols, with their content being twelve to seventeen times higher than that of carob molasses residues and carob seeds.
Table 3 Vitamin C, carotenoids, and polyphenols content of the by-products studied (carob molasses residues and carob seeds (Ceratonia siliqua L. cv. Bouzegza), eggshells, and bitter orange peels (Citrus aurantium L. cv. Bigarade)) collected in Boumerdès, Algeria, 2021.
CMR | Carob seed | Eggshells | Bigarade peels | |
Vitamin C (mg AAE/100 g FM) | Nd | Nd | Nd | 57 |
Carotenoids (mg EBc/100 g FM) | 0.21c | 6.11b | Nd | 100. 85a |
Total phenol (mg GAE/g FM) | 3.70b | 2.84 c | Nd | 35.82a |
AAE: Ascorbic acid equivalent. βCE: β-carotene equivalents. GAE: Gallic acid equivalent. FM: Fresh matter. Nd: Not determined. CMR: Carob molasses residue. / AAE: Equivalente de ácido ascórbico. βCE: Equivalentes de β-caroteno. GAE: Equivalente de ácido gálico. FM: Materia fresca. Nd: No determinado. CMR: Residuo de melaza de algarroba.
Values with the same letter in each row indicate no significant difference (p > 0.05). The results are presented in descending order: a > b > c. / Los valores con la misma letra en cada fila no muestran diferencias significativas (p > 0,05). Los resultados se presentan en orden descendente: a > b > c.
Fiber content of the by-products studied
Insoluble fiber (crude cellulose, NDF, ADF and ADL) and soluble fiber (pectin and gums) contents depended on the type of by-product (Table 4). Insoluble fiber contents are similar (p > 0.05) between carob molasses residue and Bigarade peel, and significantly higher (p ≤ 0.05) than those of carob seeds. Based on the results obtained, we note that for the pectin content, the orange peels are significantly richer (p ≤ 0.05), followed by the residue of molasses and carob seeds. Crude gums were determined only in carob seeds.
Table 4 The dietary fiber composition of the by-products studied (carob molasses residues and carob seeds (Ceratonia siliqua L. cv. Bouzegza) and bitter orange peels (Citrus aurantium L. cv. Bigarade)) collected in Boumerdès, Algeria, 2021.
CMR | Carob seed | Egg shells | Bigarade peels | |
Crude cellulose (% FM) | 11,72a | 07.33b | Nd | 10.92a |
Neutral detergent fiber (NDF) (% FM) | 35,71b | 31.54c | Nd | 38.06a |
Acid detergent fiber (ADF) (% FM) | 25,32a | 21.07b | Nd | 25.14a |
Acid detergent lignin (ADL) (% FM) | 13.38a | 09.13c | Nd | 12.21b |
Pectins (% FM) | 10.17b | 2.82c | Nd | 35.67a |
Yield of crude gums (% FM) | Nd | 38.94 | Nd | Nd |
Total carbohydrate (%FM) | 36.63b | 50.82a | Nd | 29.23c |
Available carbohydrate (%FM) | 24.91b | 43.49a | Nd | 18.31c |
CMR: Carob molasses residue. FM: Fresh matter. Nd: Not determined. / CMR: Residuo de melaza de algarroba. FM: materia fresca. Nd: No determinado.
Values with the same letter in each row indicate no significant difference (p > 0.05). The results are presented in descending order: a > b > c. / Los valores con la misma letra en cada fila no muestran diferencias significativas (p > 0,05). Los resultados se presentan en orden descendente: a > b > c.
Total carbohydrate (by difference) = 100 − (proteins + ash + water + fat). Available carbohydrate (by difference) = 100 − (proteins + ash + water + lipids + crude cellulose). / Hidratos de carbono totales (por diferencia) = 100 − (proteínas + ceniza + agua + grasa). Hidratos de carbono disponibles (por diferencia) = 100 − (proteínas + ceniza + agua + lípidos + celulosa bruta).
The higher the crude fiber content in a food, the lower the available energy it provides, expressed as available carbohydrate. This relationship can be visualized using a spider diagram. Carob seeds, with their lower insoluble fiber content, provide more available energy. Conversely, carob molasses residue and Bigarade peel, with higher insoluble fiber content, provide less available energy (Figure 1).
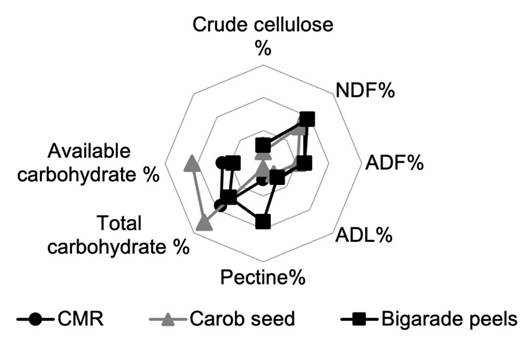
Figure 1. Comparison of available energy content, expressed as available carbohydrates, and fiber content of by-products (carob molasses residues and carob seeds (Ceratonia siliqua L. cv. Bouzegza), eggshells, and bitter orange peels (Citrus aurantium L. cv. Bigarade)) collected in Boumerdès, Algeria, 2021. Acid detergent fiber (ADF), acid detergent lignin (ADL), carob molasses residue (CMR), fresh matter (FM), neutral detergent fiber (NDF).
Discussion
The by-products analyzed were found to be low in fat and protein, and moderately high in sugar. Determining the levels of their macronutrients provides information, according to Gillespie et al. (2023), on the calorific value of the supplement (easily available energy), its palatability, and its digestive capacity by promoting efficient fermentations in the rumen. The total sugar content in carob seeds and Bigarade peel agrees with those reported by Lagha-Benamrouche et al. (2023) (27.36 %) and Lagha-Benamrouche et al. (2018) (10.77 %), respectively.
For carob molasses residue, the sugar contents are lower compared to bibliographic data, and the reducing sugar content is four times higher than that of non-reducing sugars. Ibrahim et al. (2020) recorded total sugar contents of 54.29 %, including 15.6 % reducing sugars and 39.15 % non-reducing sugars. This difference in results can be explained by the effect of fruit variety, ripening rate, acidity, and, above all, by molasses cooking conditions and their influence on the conversion of non-reducing sugars into reducing sugars under the effect of heat and acidity (Lagha-Benamrouche & Benkaci, 2022).
The protein contents of the carob molasses residue, carob seeds and Bigarade peel are in agreement with the contents given by Lagha-Benamrouche et al. (2023) for carob pulp (6.38 %-6.56 %) and carob seeds (30 %), and Lagha-Benamrouche et al. (2018) (1.67 %) for Bigarade peel (1.68 %). Regarding the protein content of the shells, it is twice lower compared to bibliographical data (Neunzehn et al., 2015; Rosnah et al., 2021). This difference in results can be explained by the fact that the shells were washed, thus removing certain membranes attached to the shell.
The fat content of carob seeds is close to the value found by Lagha-Benamrouche et al. (2023) (9.37 %), but the content found for bitter orange peels is lower than those reported by Thiviya et al. (2022) (1.4 %). This difference in results is due to various parameters that influence quantification, including particle size, humidity, the nature of the solvent and the extraction method used (Grundy et al., 2008).
The total energy value of the food supplement is estimated to be 478.19 kcal/100 g. This value surpasses that of corn (313.4 to 323.6 kcal/100 g) and wheat (306.7 to 317.6 kcal/100 g), which are commonly used in livestock feed due to their high starch and protein content (Barzegar et al., 2019). According to the results obtained in this study, 80 % of the supplement’s energy comes from carob seeds, which are rich in proteins, sugars and fats compared to other by-products.
The ash content was determined to guarantee compliance with human and animal consumption thresholds that should not be exceeded. According to Lagha-Benamrouche et al. (2018), a high ash content in a co-product may result from an accumulation of mineral materials of exogenous origin, such as contamination by soil due to insufficient washing. The mineral content of carob molasses residue (0.78 %) is lower than that of the pod (3.9 to 4.57 % according to Fadel et al. (2020)). This difference can be explained by the entrainment of mineral salts in molasses, which depletes the residue. When comparing the obtained results with bibliographical data, it appears that the ash content of eggshells, Bigarade peel and carob seeds are close to those reported by Rosnah et al. (2021) for eggshells (95 %); M’Hiri et al. (2015) (2.52 %) for Bigarade, and Lagha-Benamrouche et al. (2023) for seeds (5.66 %).
The mineralogical study revealed the predominance of calcium, magnesium and phosphorus in eggshells; calcium, magnesium and sodium in carob residues, and copper, potassium and magnesium in Bigarade peel. The mineral compositions of the samples tested align with those reported by Lagha-Benamrouche and Hezil (2023), Lagha-Benamrouche et al. (2023), Rosnah et al. (2021), and Abdelazem et al. (2021) for carob pulp, carob seeds, eggshells, and Bigarade peel, respectively.
Minerals are indispensable for life, each playing a specific role in the body (Rosnah et al., 2021). Magnesium acts as a crucial cofactor in numerous cellular metabolic reactions, including protein synthesis, nerve impulse transmission, and muscle movements. Calcium and phosphorus contribute to muscle contraction, nerve impulse transmission, and bone and teeth formation (Habraken et al., 2016). Sodium and potassium form a pump that aids in restoring membrane permeability (Jean Constant et al., 2020). Copper participates in multiple enzymatic reactions, including collagen and neurotransmitter synthesis (Jean Constant et al., 2020; Rosnah et al., 2021). These by-products are recommended to maintain a healthy balance and ensure proper bodily functions.
Bioactives are micronutrients present in small quantities in raw materials, essential for metabolic functioning and overall well-being (Versha et al., 2023). Vitamin C, carotenoids, and polyphenols possess antioxidant properties, scavenging free radicals generated by the body or in response to environmental aggressors (e.g., pollutants, infections) (Lagha-Benamrouche, 2022). Ingesting these compounds with food reinforces the body’s natural defenses against oxidative stress, aiding in the prevention of various chronic pathologies (Lagha-Benamrouche & Madani, 2013).
Orange peel serves as a rich source of vitamin C, carotenoids, and phenolic compounds (Lagha-Benamrouche et al., 2018). The estimated contents of ascorbic acid and polyphenols in Bigarade peel are 57 mg/100 g and 35.82 mg/g, respectively (Table 3), aligning closely with findings by Lagha-Benamrouche et al. (2018) (56.9 mg/100 g for vitamin C) and Lagha-Benamrouche and Madani (2013) (35.82 mg/g for polyphenols). However, the carotenoid content of Bigarade peel is half of that reported by Lagha-Benamrouche et al. (2018) (100.85 mg βCE/g MF vs. 218 mg βCE/g MF). Carotenoid levels are influenced by growing conditions and fruit ripeness, with the transition from green to orange-yellow corresponding to the unmasking of carotenoids due to chlorophyll degradation (Hulkko et al., 2022). Additionally, factors such as assay methods and plant material storage conditions can affect quantification accuracy (Lagha-Benamrouche et al., 2018).
Bigarade peel stands out for its high fiber content, particularly in water-soluble fiber like pectin. Orange peel, comprising around 45 % of the total citrus fruit, is a prime source for extracting pectin, with approximately 30 % pectin content on a dry matter basis, consistent with the findings of this study (35 % vs. 30 %) (Fakayode & Abobi, 2018; Kute, 2020). Additionally, crude gums were exclusively identified in carob seeds, with a raw gum yield estimated at 38.94 % (Table 4). This result closely aligns with the findings of Lagha-Benamrouche et al. (2023) (39.44 %).
Pectins and gums, being soluble fibers, undergo fermentation by intestinal microbiota, yielding beneficial compounds for the body. Upon contact with liquids, they become viscous, facilitating the passage of residues (Debnath et al., 2022).
Comparing the crude fiber content of the analyzed carob samples with existing literature, it’s evident that seeds provide less fiber than the pulp (Boublenza et al., 2019; Dahmani et al., 2023), consistent with this investigation’s findings. Crude fiber encompasses plant cell wall components (cellulose, hemicellulose, lignin) that are indigestible, constituting the portion of feed not energetically utilized by animals. Despite being indigestible, crude fiber is vital for stimulating digestion and maintaining gut health in animals (Debnath et al., 2022).
The by-products examined displayed moderate to low levels of neutral detergent fiber, acid detergent fiber and acid detergent lignin. Higher neutral detergent fiber percentages correlate with reduced dry matter consumption, while increased acid detergent fiber levels correlate with decreased forage digestibility (Arelovich et al., 2008). Consequently, Bigarade peels and carob residue represent valuable additions to animal diets.
Conclusions
This study endeavors to characterize food waste meticulously to devise a dependable and precise formulation of food supplements, ensuring they effectively fulfill the requirements of farm animals. These food by-products boast an array of bioactive compounds, including vitamin C, carotenoids, phenolic compounds, and essential minerals, while also providing readily available energy. By combining these by-products, a value-added food supplement can be formulated, recommended for enriching livestock feed and ensuring optimal organismal function. This valuation could benefit the environment, the national economy, as well as human health. It could help limit the impact of the processing industry on environmental pollution and thus reduce the high costs of treating industrial waste. In addition, it might enable the exploitation of bioactive molecules and incorporate them into diets in order to develop functional foods rich in nutritional quality and with high antioxidant potential.