Introducción
Los agroecosistemas productivos de cafeto (Coffea arabica L.), son de importancia económica y ecológica a nivel mundial, debido a su impacto financiero y ambiental en especial en países en vías de desarrollo (Siu Palma et al., 2023; Villalta-Villalobos & Gatica-Arias, 2019). Este rubro en Nicaragua constituye el 25 % de las exportaciones del país. La producción se concentra en los departamentos de Jinotega (35 %), Matagalpa (25 %), Nueva Segovia (13 %) y Madriz (8 %) (Ministerio Agropecuario, 2019).
El estudio de la composición de los sistemas productivos de cafeto es de importancia. Los componentes que se encuentran por encima y por debajo de la superficie del suelo, conforman redes complejas y dinámicas, en donde el productor con sus decisiones de manejo influye en las interacciones bióticas y abióticas (Quiroz Guerrero et al., 2021).
Dentro de los componentes de los sistemas productivos se encuentra la macrofauna edáfica, los macroorganismos son de fácil detección (Cabrera Dávila et al., 2022; Cabrera-Dávila et al., 2017). La macrofauna edáfica es un componente de mucha importancia en el suelo al tener funciones que mejoran la estructura y regulan el comportamiento de otros organismos (Gedoz et al., 2021; Scaglione et al., 2023). El estudio de la composición de las comunidades bióticas constituye una herramienta fundamental para comprender las condiciones edáficas (Gedoz et al., 2021).
Algunas clases y órdenes de artrópodos intervienen en los procesos de transformación del suelo (Annelida: Oligochaeta), la formación de poros (Insecta: Hymenoptera, e Isopoda) y la trituración de restos vegetales (Coleoptera, Diplopoda, Isopoda y Gastropoda), lo que permite inferir la importancia de la composición biológica del suelo y sus funciones en los sistemas productivos (Chávez-Suárez et al., 2023).
Investigaciones efectuadas mencionan que las alteraciones en las propiedades edáficas como consecuencia del manejo de los suelos o cambios en la cobertura vegetal por el manejo del cultivo provoca cambios en la macrofauna del suelo (Cabrera Dávila et al., 2022; Suárez Salazar et al., 2015). El manejo óptimo de la cobertura vegetal contribuye a la estabilidad de las poblaciones de artrópodos edáficos. La abundancia y riqueza de las comunidades de invertebrados se debe al aporte de materia orgánica fresca (hojarasca) al suelo, lo cual provee de energía y refugio, y regula el microclima (Suárez Salazar et al., 2015).
En Nicaragua existe poca información que asocie el manejo de los sistemas de producción de cafeto con la fauna del suelo y la hojarasca presente, así como la presencia o ausencia de los grupos tróficos, con el propósito de analizar la complejidad que ocurre dentro del agroecosistema (Quiroz Medina et al., 2021; Quiroz-Medina & Bárcenas-Lanzas, 2023; Rodríguez González & Salazar Centeno, 2021; Rodríguez González et al., 2022). El objetivo de este estudio fue estimar las poblaciones de macrofauna edáfica en agroecosistemas productivos de cafeto y su relación con la fertilidad del suelo y las diferentes prácticas de manejo implementadas por productores de la reserva natural Tepec-Xomolth La Patasta, Madriz, Nicaragua.
Materiales y métodos
El estudio se realizó de mayo a agosto del 2023, en la reserva natural Tepec-Xomolth La Patasta, declarada área protegida bajo la categoría de Reserva Natural por el Decreto Ejecutivo n°. 42-91, según los límites establecidos por el Ministerio del Ambiente y los Recursos Naturales el área total es de 116,65 km², de los cuales 65,70 km² corresponden al territorio de Madriz (56,32 % del área protegida). Esta área es compartida por los municipios de San Lucas con 26,90 km², Las Sabanas 16,07 km² y San José de Cusmapa con 22,73 km² (Presidente de la República de Nicaragua, 1991).
La reserva natural Tepec-Xomolth La Patasta se encuentra localizada entre los 13º 12º y 13º 40º de latitud norte y 86º 05º y 86º 45º de longitud oeste en un territorio de topografía irregular con altitudes de 1000 y 1735 m s. n. m. Su clima se caracteriza por cambiar en función de la altitud: de tropical seco en las partes bajas a tropical húmedo en las elevaciones y montañas. La época de lluvia abarca los meses de abril a julio; luego, entre octubre y noviembre. Las temperaturas promedio fluctúan entre los 16 y 23 ºC, mientras que la humedad relativa presenta rangos de 51,91 a 100 % en las elevaciones (Morán Centeno & Jiménez-Martínez, 2023). Se seleccionaron agroecosistemas productivos de cafeto en las comunidades de El Pegador, Nueva Esperanza y Buena Vista, las cuales son parte de la reserva antes mencionada.
Diseño metodológico
La investigación se efectuó en pequeños agroecosistemas productivos (menores a cinco hectáreas) con cafeto arábica, con un enfoque cuantitativo, no experimental de corte transversal, cuyo alcance fue descriptivo y correlacional, en donde no hubo manipulación de variables, limitándose a la recolección de datos en un único momento (Rodríguez González & Salazar Centeno, 2021; Rodríguez González et al., 2022; Valdez Arévalo, 2021).
Selección de los sistemas productivos de café
La selección de los sistemas productivos de cafeto se llevó a cabo en el período comprendido entre los meses de mayo a agosto del 2023, en tres comunidades de la reserva natural Tepec-Xomolth La Patasta. Se tomó una muestra de catorce sistemas productivos (Nueva Esperanza = 5, El Pegador = 4, Buena Vista = 5), todos dedicados a la producción de cafeto (Morán Centeno & Jiménez-Martínez, 2023). En todos los sistemas evaluados se empleaban como parte del manejo al cultivo las siguientes prácticas: control químico de plagas, curvas a nivel (obras de conservación de suelo), manejo de sombra en el cultivo de cafeto, poda de mantenimiento y poda sanitaria del cafeto.
Levantamiento de muestras de suelo para análisis químico
Por cada sistema productivo se realizaron veinte submuestreos a una profundidad de 20 cm, los cuales se mezclaban para conformar muestras compuestas de un kilogramo de suelo. Se trasladaron al Laboratorio de Suelos y Agua (LABSA), de la Universidad Nacional Agraria (UNA), para determinar parámetros químicos (macro y micronutrientes), con el empleo de los métodos establecidos en Salgado García et al. (2013).
Muestreo y procesamiento de la macrofauna edáfica
Para el muestreo de la macrofauna edáfica se colectaron monolitos de suelo (25 × 25 × 20 cm), en cada sistema productivo, se trazaron tres transectos similares de 50 m de longitud, separados por 50 m, se extrajeron tres monolitos por transecto (Rodríguez González & Salazar Centeno, 2021; Rodríguez González et al., 2022) y luego se colocaron en bolsas plásticas y revisadas en bandejas (Valdez Arévalo, 2021).
Los especímenes (adultos y larvas), colectados y contabilizados de forma manual fueron rotulados y almacenados en alcohol al 70 %, con excepción de las lombrices de tierra, que fueron conservadas en formol al 4 % para evitar su desecación (Rodríguez González & Salazar Centeno, 2021; Valdez Arévalo, 2021). Se identificó hasta el nivel taxonómico de familia. Dichos organismos se trasladaron al Museo Entomológico de la Universidad Nacional Agraria, para su identificación comparándolos con las colecciones entomológicas y bibliografía especializada (Jiménez-Martínez, 2020; Rodríguez Flores & Jiménez-Martínez, 2019), se observaron con un estereoscopio (CARL ZEISS, modelo 475002). Para todos los sistemas bajo estudio y grupos taxonómicos, se consideraron la abundancia, convertida en densidad, la cual corresponde al número de individuos (ind) por metro cúbico (ind/m3), y la riqueza total por sistema de estudio y por muestra de suelo, con base en el método de muestreo de macrofauna propuesto por el Programa de Biología y Fertilidad del Suelo Tropical (TSBF), según Anderson e Ingram (1994), a los cuales se le asignó su rol ecológico en el sistema productivo de cafeto (Cabrera Dávila & López Iborra, 2018; Cabrera Dávila et al., 2022; Quiroz Medina et al., 2021). Además, se calcularon los índices de diversidad para comparar comunidades y prácticas empleadas por los productores.
Análisis estadístico
Para evaluar los agroecosistemas de cafeto sobre las variables químicas de suelo, se utilizó un análisis de varianza, con modelos lineales generalizados, para lo cual se consideraron las comunidades y prácticas como efectos fijos y separación de medias al 95 % de confianza (Tukey ≤ 0,05).
En cuanto a la diversidad de organismos se obtuvo un único valor de abundancia para cada muestreo, en cada práctica, mediante la sumatoria de los individuos y especies registradas en cada monolito. Se aplicaron modelos lineales generalizados, las familias se tomaron como variables discretas. Del conjunto de modelos planteados, se seleccionó el que mostró el menor valor de Akaike (AIC), en donde hubo diferencias significativas entre los factores. Se procedió a realizar comparaciones mediante la prueba de Tukey (0,05), se empleó estadística descriptiva y análisis de componentes principales.
La abundancia se estimó con base en la frecuencia de captura de una especie (n-veces), en cada sistema de cultivo (Índice de Shannon - Weaver), la diversidad se determinó mediante el índice de Simpson, para la comunidad rural y el tipo de práctica de manejo. Para ello, se empleó el software R v.4.2.3 (R Core Team, 2023).
Resultados
Análisis químicos del suelo
Al comparar los parámetros químicos del suelo en las comunidades bajo estudio, se encontró que el contenido nutricional del suelo varía en las diferentes comunidades de manera significativa; en aquellos sistemas productivos de cafeto ubicados a altitudes menores (inferiores a 1200 m s. n. m.), mostraron los mayores porcentajes de carbono orgánico (3,34 a 5,57 %). Las concentraciones de calcio y fósforo presentaron un comportamiento similar, y en las comunidades de Nueva Esperanza y El Pegador se registraron altos valores (Cuadro 1).
Cuadro 1 Composición química en los sistemas productivos de cafeto evaluados en tres comunidades de la reserva natural Tepec-Xomolth La Patasta, Nicaragua. 2023.
Comunidad rural | Latitud | Longitud | Altitud de los sistemas productivos m s. n. m. | COT % | P mg/kg | Ca mmol/kg | ||||
Nueva Esperanza | 531870 | 1476806 | F1 (1140,12) | 5,57 | a | 48,39 | a | 337,22 | a | |
Nueva Esperanza | 539815 | 1476759 | F2 (1159,20) | 3,34 | bc | 24,98 | ab | 259,87 | abc | |
Nueva Esperanza | 539704 | 1476979 | F3 (1017,13) | 3,67 | bc | 12,53 | b | 260,38 | abc | |
Nueva Esperanza | 540088 | 1477020 | F4 (1130,50) | 4,27 | ab | 6,65 | b | 246,73 | abc | |
Nueva Esperanza | 540013 | 1477074 | F5 (1083,53) | 4,36 | ab | 22,61 | ab | 293,11 | ab | |
El Pegador | 540976 | 1477119 | F6 (1490,52) | 2,63 | c | 3,23 | b | 157,53 | c | |
El Pegador | 540414 | 1476500 | F7 (1501,50) | 3,74 | bc | 4,97 | b | 176,21 | c | |
El Pegador | 540569 | 1476586 | F8 (1143,13) | 5,51 | a | 49,80 | a | 335,01 | a | |
El Pegador | 540539 | 1476622 | F9 (1159,40) | 3,34 | bc | 25,37 | ab | 262,70 | abc | |
Buena Vista | 539790 | 1477226 | F10 (1019,13) | 3,67 | bc | 12,23 | b | 253,47 | abc | |
Buena Vista | 540518 | 1476501 | F11 (1103,30) | 4,32 | ab | 7,82 | b | 267,32 | abc | |
Buena Vista | 541983 | 1471991 | F12 (1133,10) | 4,51 | ab | 9,75 | b | 250,44 | abc | |
Buena Vista | 541890 | 1471578 | F13 (1268,38) | 3,37 | bc | 19,12 | ab | 217,54 | bc | |
Buena Vista | 541981 | 1471357 | F14 (1508,76) | 3,33 | bc | 4,50 | b | 181,40 | bc | |
Media | 3,94 | 17,87 | 246,85 | |||||||
C.V (%) | 14,74 | 74,51 | 18,50 | |||||||
R2 | 0,81 | 0,72 | 0,74 | |||||||
Pr | 0,0091 | 0,05 | 0,04 |
** Promedios con letra en común no difieren estadísticamente (α = 0,05), F: sistemas productivos de café, COT: carbono orgánico, P: fósforo, Ca: calcio. / ** Averages with letter in common do not differ statistically (α= 0.05), F: coffee production systems, COT: organic carbon, P: phosphorus, Ca: calcium.
Clasificación taxonómica, rol trófico y diversidad de la macrofauna edáfica
De acuerdo con el rol ecológico que desempeñan los diferentes órdenes y familias el orden Haplatoxida (Lumbricidae), fue el más abundante, seguido del orden Hymenoptera (Formicidae), Coleoptera (Elateridae) e Isopoda (Rhinotermitidae), en las comunidades rurales. Otro aspecto de importancia a mencionar es la presencia de depredadores lo que se puede asociar a un control natural y al mantenimiento de un equilibrio en el sistema productivo, sobresalieron las familias Opiliones, Theraphosidae y Forficulidae. En cuanto al grupo de los detritívoros, estuvieron representados por las familias Lumbricidadae, Termitidae, Rhinocricidae, Emildae, Tenebrionidae y Porcellionidae. La diferencia entre los grupos funcionales entre las comunidades sugiere distintos niveles de activación biológica del suelo (Cuadro 2).
Cuadro 2 Clasificación taxonómica, rol trófico y diversidad de la macrofauna edáfica en sistemas productivos de cafeto en tres comunidades de la reserva natural Tepec-Xomolth La Patasta, Nicaragua. 2023.
Comunidades rurales | ||||||||||
Orden | Familia | Nueva Esperanza | Buena Vista | El Pegador | Grupo funcional | |||||
Ind/m3 | % | Ind/m3 | % | Ind/m3 | % | |||||
Araneae | Opiliones | 784 | 3,8 | NP | 0,0 | NP | 0,0 | Depredadores | ||
Ê | Salticidae | NP | 0,0 | 160 | 4,7 | 384 | 3,3 | Depredadores | ||
Ê | Theraphosidae | NP | 0,0 | 64 | 1,9 | 608 | 5,3 | Depredadores | ||
Haplotaxida | Lumbricidae | 10 784 | 52,2 | 208 | 6,1 | 1 056 | 9,1 | Detritívoros | ||
Scolopendromorpha | Scolopendridae | 960 | 4,6 | 160 | 4,7 | 752 | 6,5 | Depredadores | ||
Spirobolida | Rhinocricidae | NP | 0,0 | NP | 0,0 | 64 | 0,6 | Detritívoros | ||
Mesogastrópodo | Planorbidae | NP | 0,0 | 48 | 1,4 | 48 | 0,4 | Herbívoros | ||
Pulmonata | Helicidae | NP | 0,0 | 64 | 1,9 | 608 | 5,3 | Herbívoros | ||
Veronicellidae | 624 | 3,0 | NP | 0,0 | 112 | 1,0 | Herbívoros | |||
Blattodea | Blatellidae | 16 | 0,1 | 48 | 1,4 | 96 | 0,8 | Omnívoros | ||
Coleoptera | Buprestidae | NP | 0,0 | 16 | 0,5 | 96 | 0,8 | Detritívoros | ||
Chrysomelidae | 240 | 1,2 | 32 | 0,9 | 144 | 1,2 | Herbívoros | |||
Cleridae | NP | 0,0 | NP | 0,0 | 32 | 0,3 | Herbívoros | |||
Coccinellidae | NP | 0,0 | 32 | 0,9 | 64 | 0,6 | Depredadores | |||
Curculionidae | 48 | 0,2 | 48 | 1,4 | 16 | 0,1 | Herbívoros | |||
Elateridae | 80 | 0,4 | 48 | 1,4 | 608 | 5,3 | Depredadores | |||
Elmidae | NP | 0,0 | 96 | 2,8 | 432 | 3,7 | Detritívoros | |||
Lampyridae | NP | 0,0 | 16 | 0,5 | 160 | 1,4 | Depredadores | |||
Scarabaeidae | 1392 | 6,7 | 208 | 6,1 | 576 | 5,0 | Herbívoros | |||
Staphylinidae | 320 | 1,5 | NP | 0,0 | 96 | 0,8 | Depredadores | |||
Tenebrionidae | 32 | 0,2 | 192 | 5,7 | 160 | 1,4 | Detritívoros | |||
Dermaptera | Forficulidae | 144 | 0,7 | 112 | 3,3 | 96 | 0,8 | Depredadores | ||
Diptera | Stratiomyidae | 32 | 0,2 | NP | 0,0 | 16 | 0,1 | Detritívoros | ||
Hemiptera | Aphididae | NP | 0,0 | 32 | 0,9 | 2 | 0,0 | Herbívoros | ||
Cercopidae | NP | 0,0 | 16 | 0,5 | 1 | 0,0 | Depredadores | |||
Gelastocoridae | 32 | 0,2 | 48 | 1,4 | 16 | 0,1 | Depredadores | |||
Lygaeidae | 80 | 0,4 | 48 | 1,4 | 15 | 0,1 | Depredadores | |||
Reduviidae | 80 | 0,4 | 16 | 0,5 | 25 | 0,2 | Depredadores | |||
Hymenoptera | Formicidae | 2000 | 9,7 | 1056 | 31,1 | 3008 | 26,1 | Omnívoros | ||
Mutillidae | NP | 0,0 | 320 | 9,4 | 112 | 1,0 | Depredadores | |||
Vespidae | NP | 0,0 | NP | 0,0 | 80 | 0,7 | Herbívoros | |||
Lepidoptera | Noctuidae | 32 | 0,2 | 16 | 0,5 | 32 | 0,3 | Herbívoros | ||
Sphingidae | 32 | 0,2 | 16 | 0,5 | 48 | 0,4 | Herbívoros | |||
Orthoptera | Acrididae | NP | 0,0 | 48 | 1,4 | 16 | 0,1 | Herbívoros | ||
Gryllidae | 176 | 0,9 | 16 | 0,5 | 144 | 1,2 | Herbívoros | |||
Isopoda | Porcellionidae | NP | 0,0 | 96 | 2,8 | 288 | 2,5 | Detritívoros | ||
Rhinotermitidae | 2784 | 13,5 | 64 | 1,9 | 16 | 0,1 | Detritívoros | |||
Termitidae | NP | 0,0 | 48 | 1,4 | 1520 | 13,2 | Detritívoros | |||
Total | 20 672 | 100 | 3 392 | 100 | 11 547 | 100 | ||||
Depredadores (n = 6457) | ||||||||||
Detritívoros (n = 18 000) | ||||||||||
Herbívoros (n = 4930) | ||||||||||
Omnívoros (n= 6224) |
%: porcentaje relativo, NP: sin presencia, Ind/m3: individuos por metro cuadrado. / %: relative percentage, NP: no presence, Ind/m3: individuals per square meter.
Macrofauna edáfica por comunidad y práctica de manejo del cultivo
Al analizar la macrofauna edáfica total en cada una de las comunidades bajo estudio en los sistemas de producción de café, la comunidad Nueva Esperanza mostró la mayor cantidad de individuos por metro cuadrado, lo que indica mayor abundancia de macroinvertebrados, seguida de la comunidad El Pegador y, en menor grado, Buena Vista (Figura 1). En cuanto al manejo que el productor realiza en sus sistemas productivos, se determinó que el uso de curvas a nivel y el manejo de sombra presentaron la mayor cantidad de individuos (Figura 2).
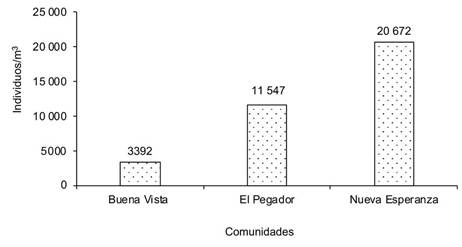
Figura 1 Número de individuos de la macrofauna edáfica en los sistemas de producción de cafeto en tres comunidades de la reserva natural Tepec-Xomolth La Patasta, Nicaragua. 2023.
Individuos por clase de la macrofauna edáfica
Se registró la mayor cantidad de individuos para la clase Insecta (n = 13 303), seguida de Clitellata (n =12 048) y, en menor cantidad, Arachnida, Diplopoda y Gastropoda. Estos resultados indican que la presencia de insectos en los sistemas productivos de cafeto es abundante, donde desempeñan diversas funciones ecológicas, debido al manejo que realizan los productores (Figura 3).
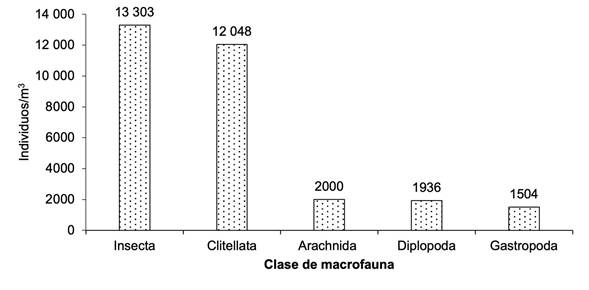
Figura 3 Número de individuos de la macrofauna edáfica por clase en el cultivo de cafeto en tres comunidades de la reserva natural Tepec-Xomolth La Patasta, Nicaragua. 2023
Al analizar las diferentes prácticas de manejo del cutivo de cafeto en relación con las familias de artrópodos, se encontró que en aquellos sistemas productivos en donde se implementan podas sanitarias, podas de mantenimiento y curvas a nivel, predominan las familias Pentatomidae, Formicidae, Elasteridae, Tenebrionidae, Blattidae, Salticidae, Termitidae y Emilcidae; al aplicar manejo de sombra son abundantes las familias Reduviidae y Scarabaeidae; por su parte, el control químico de plagas se relacionó con las familias Carabidae, Lygaeidae, Opiliones, Noctuidae, Culicidae y Navidae (Figura 4). Otras familias como Forficulidae, Chrysomelidae, Cydnidae Rhinotermitidae y Gryllidae no se asociaron a ninguna práctica en particular, lo que indica que se encuentran distribuidas de manera general en todos los sistemas productivos.
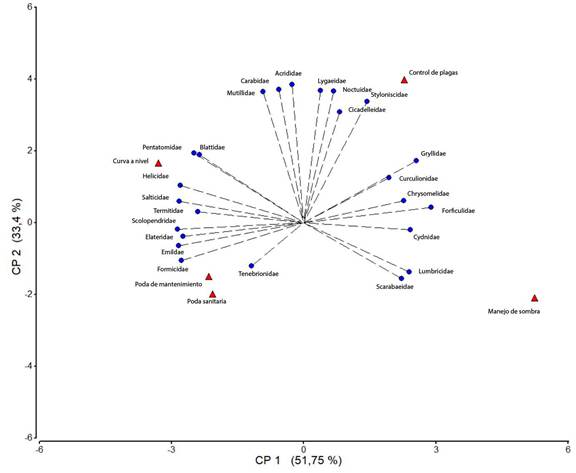
Figura 4 Asociación de las diferentes familias de artrópodos con las prácticas de manejo en el cultivo de cafeto en tres comunidades de la reserva natural Tepec-Xomolth La Patasta, Nicaragua. 2023.
Los resultados obtenidos mostraron que las comunidades de Nueva Esperanza y El Pegador fueron donde se presentaron los mayores valores del índice de Shannon, así como en el uso de curvas a nivel, poda de mantenimiento y poda sanitaria; valores similares fueron expresados mediante el índice de Simpson. Esto indica que los sistemas de cultivo de cafeto en las comunidades bajo estudio son manejados de manera tradicional por los productores, lo cual mantiene un equilibrio ecológico entre las poblaciones y propicia una alta diversidad (Cuadro 3). Al categorizar las prácticas de manejo se encontró que el manejo de sombra influye de manera directa en la presencia de macrofauna edáfica, seguido de las podas de mantenimiento y sanitaria. En el caso de las comunidades rurales, Buena Vista expresó el mayor número de familias de artrópodos, seguida de El Pegador; esto se reflejó en el número de familias identificadas.
Cuadro 3 Comportamiento de la macrofauna edáfica en el cultivo de cafeto en tres comunidades de la reserva natural Tepec-Xomolth La Patasta, Nicaragua. 2023.
Componentes | Índice de diversidad | Riqueza (familias) | Desviación estándar | HSD (0,05) | |||
Shannon-Weaver | Simpson | ||||||
Comunidades | |||||||
Nueva Esperanza | 2,5366 | 0,8817 | 24,67 | 3,31 | 272,33 b | ||
El Pegador | 2,0710 | 0,7066 | 30,33 | 2,06 | 110,95 b | ||
Buena Vista | 1,8118 | 0,7655 | 33,00 | 0,00 | 610,17 a | ||
Prácticas de manejo del cultivo | |||||||
Curvas a nivel | 2,5612 | 0,8777 | 29,80 | 4,66 | 325,09 e | ||
Manejo químico de plagas | 2,2251 | 0,8223 | 33,00 | 0,00 | 331,75 d | ||
Manejo de sombra | 1,7289 | 0,7485 | 33,00 | 0,00 | 420,06 a | ||
Poda de mantenimiento | 2,4028 | 0,8574 | 32,60 | 1,20 | 381,36 b | ||
Poda sanitaria | 2,4205 | 0,8598 | 33,00 | 0,00 | 373,41 c |
** Promedios con letra en común no difieren estadísticamente (α = 0,05), HSD: prueba de Tukey al 95 % de confianza. / **Average with letter in common do not differ statistically (α = 0.05), HSD: Tukey test at 95 % confidence.
Discusión
Las propiedades químicas del suelo evaluadas en los agroecosistemas de cafeto mostraron concentraciones promedio de 3,94 % de carbono orgánico, lo que refleja una tasa constante de reciclaje de materia orgánica proveniente de las prácticas de manejo de la plantación y la vegetación empleada como sombra en los estratos superiores, lo que se puede considerar una fortaleza del agroecosistema en términos de fertilidad y estructura del suelo, al incorporar en el sistema materia orgánica fresca, producto del manejo de sombra. En la mayoría de los suelos, las concentraciones de carbono orgánico varían de 0,3 a 11,5 %. Los valores obtenidos en este estudio se consideran aceptables al compararlos con sistemas agroforestales y bosques (Vargas-Larreta et al., 2023).
El valor promedio de fósforo fue 17,87 mg/kg. En aquellos cafetales ubicados a mayores altitudes, se registró menor concentración de este elemento; este mismo comportamiento se observó en la cantidad de calcio (promedio 246,85 mmol/kg). Estos resultados son similares a los reportados por Rodríguez Suárez et al. (2019), quienes indican que en sistemas productivos de cafeto los suelos registran contenidos altos de fósforo y calcio, atribuidos a la contribución de materia y fertilizantes orgánicos incorporados por el productor en sus actividades de manejo, lo que favorece la sanidad del cultivo y la biota edáfica.
Los resultados obtenidos indican cambios en la abundancia y riqueza de la macrofauna edáfica en los sistemas productivos, que vinculan las prácticas de manejo del cultivo y la creación de cambios espaciales y temporales que ocurren en aquellos espacios con mayor diversidad vegetal (Zhang et al., 2022). La cantidad de material vegetal incorporado al suelo, producto de las prácticas de manejo (podas de mantenimiento, sanitaria y manejo de sombra), suministra recursos alimenticios en el estrato superficial del suelo (Euteneur et al., 2020; Morel & Ortiz Acosta, 2022). La mejora de la estructura del suelo ofrece microespacios para la oviposición, alimentación y hábitats para estos individuos, en particular para las familias Scarabaeidae y Formicidae (Bedano et al., 2016; Jiang et al., 2018; Scaglione et al., 2023).
El funcionamiento eficiente del agroecosistema depende del manejo, el tipo de suelo, así como de las especies de animales y vegetales presentes y sus interacciones, lo que permite tener una biodiversidad funcional, para mantener o incrementar la fertilidad del suelo (Quiroz Medina et al., 2021; Quiroz-Medina & Bárcenas-Lanzas, 2023).
El grupo de macrofauna edáfica con mayor abundancia registrada fue la clase Insecta (13 303 individuos), seguida por Clitellata (12 048 individuos). Los detritívoros fueron el grupo funcional predominante en todos los sistemas de producción, con efectos directos sobre las propiedades químicas, físicas y biológicas del suelo, seguidos por los omnívoros y depredadores, que regulan las poblaciones de otros artrópodos que se encuentran presentes en el sistema productivo. Los resultados obtenidos demostraron que la presencia de hojarasca en la superficie del suelo confiere refugio y presas a los artrópodos, permitiéndoles realizar diferentes procesos y servicios en el agroecosistema (Cabrera Dávila, 2017; Cabrera Dávila & López Iborra, 2018; Cabrera-Dávila et al., 2017; Castillo-Trejo et al., 2023).
En los agroecosistemas la diversidad fue mayor cuando los productores cafetaleros efectuaron manejo de sombra de los estratos superiores. Esta práctica permite mayor entrada de luz solar y movilidad a los órdenes Hymenopteras, Coleopteras y Haplotaxida, bajo las plantas de cafeto, lo que incrementa sus hábitats, refugio y, por ende, la cantidad de presas. En términos generales, se considera que la variabilidad en la diversidad de artrópodos fue intermedia en todos los cafetales. Existe evidencia documentada de que el grado de perturbación del suelo y las prácticas de manejo del cultivo influyen en la salud del cultivo y la diversidad de organismos presentes (Euteneur et al., 2020; Mesa-Pérez et al., 2016). Los atributos químicos y físicos del suelo, y la humedad presente en los agroecosistemas, contribuyeron a la diversidad y abundancia de la macrofauna edáfica (Quiroz-Medina & Bárcenas-Lanzas, 2023).
Conclusiones
La riqueza taxonómica y la abundancia de la macrofauna edáfica, fueron mayores en la comunidad Nueva Esperanza, cuando se empleó manejo de sombra. La clase Insecta predominó, seguida de Clitellata en todos los agroecosistemas. El grupo funcional de detritívoros fue predominante en los sistemas productivos de cafeto, seguido por los omnívoros y depredadores. La incorporación de materia orgánica fresca contribuyó de manera significativa a la abundancia de la macrofauna edáfica. La fertilidad del suelo varió en dependencia de la altitud a la que se encontraba el sistema productivo.