Introducción
El agroecosistema es una representación de un sistema alopoiético con una red compleja y dinámica de interacciones entre recursos naturales bióticos y abióticos, la tecnología, la economía y la cultura (Quiroz Guerrero et al., 2021). Donde el conocimiento local y la toma de decisiones del productor depende, en gran medida, del contexto socioeconómico, lo cual deviene en un proceso de autoorganización y evolución constante a través del aprendizaje (Gao et al., 2016; Quiroz Guerrero et al., 2021).
El agroecosistema café (AES-café) forma parte de un sistema socio-ecológico, que puede ser analizado desde una perspectiva de adaptación cíclica integrada por cuatro fases: 1) (r) la explotación de los recursos naturales y el crecimiento que se fusiona con una fase de conservación, 2) (K) un avance lento y acumulativo en el ciclo, 3) el desarrollo de un proceso de caos y colapso (Ω) y 4) una fase de reorganización (α), la cual puede ser lenta o rápida y con posibilidad de realizar innovaciones en el agroecosistema a través del aprendizaje (Peterson et al., 2018; Walker et al., 2004).
Se considera que la resiliencia es una propiedad emergente de los agroecosistemas que se expresa a partir de la fase K. Para este trabajo, la capacidad de resiliencia es el conjunto de condiciones y aptitudes que permiten al agroecosistema absorber la perturbación y reorganizarse mientras sufre cambios, para conservar su función, estructura, identidad y procesos de realimentación (Folke, 2016; Walker et al., 2004).
En México, la mayor producción de café se basa en la especie arábica (Coffea arabica L.), con el 95 % y la especie robusta (C. canephora Pierre ex A. Froehner) con el 5 % del café ofertado en los mercados nacionales (Asociación Mexicana de la Cadena Productiva del Café (AMECAFE), 2017; Centro de Estudios Para el Desarrollo Rural Sustentable y la Soberanía Alimentaria (CEDRSSA), 2020; Fideicomisos Instituidos en Relación con la Agricultura (FIRA), 2016). La producción de café se realiza en doce entidades federativas del país, con una participación importante de productores indígenas y minifundistas (CEDRSSA, 2020; Zamarripa Colmenero & Escamilla Prado, 2002). Más del 80 % de la producción nacional se destina a la exportación, México es el principal productor de café orgánico a nivel mundial (CEDRSSA, 2020).
En la historia reciente, el AES-café ha experimentado diferentes crisis relacionadas a problemas socio económicos y a brotes de enfermedades, los cuales iniciaron en un contexto nacional, afectaron al municipio de Tezonapa y generaron daño, después se inició un proceso de recuperación (Renard, 2010). En el periodo de 1988 a 1993, el gobierno mexicano desmanteló el Instituto Mexicano del Café (INMECAFE), por lo que los pequeños productores quedaron en riesgo de colapsar ante las nuevas condiciones del mercado y precios, lo que generó una crisis socioeconómica nacional (Renard, 2010). La devaluación del peso mexicano hizo más severo el efecto de esta crisis y se reflejó en una menor inversión de los productores para el mantenimiento de sus plantaciones (Renard, 2010; Zamarripa Colmenero & Escamilla Prado, 2002).
En 2012, la roya (Hemileia vastatrix Berk et Br), que es considerada la enfermedad más destructiva para los cafetos, ocasionó estragos en todas las zonas cafetaleras de México. En Veracruz, a principios de 2013 se reportaron más de 10 mil hectáreas afectadas por roya. Para el ciclo 2015-2016, productores del estado de Oaxaca y Guerrero sufrieron pérdidas de hasta un 95 %, lo que marcó la salida de México de los diez principales países productores de café (International Coffee Organization, n.d.; Libert-Amico & Paz-Pellat, 2018).
Se espera que el cambio climático también ocasione daños al AES-café, ya que mediante el modelado de escenarios se puede observar una reducción de la extensión actual de las zonas aptas o ambientes para el cultivo de C. arabica, por lo que este efecto incluye los municipios productores del estado de Veracruz (CEDRSSA, 2020; de Sousa et al., 2019), en donde Tezonapa es una de las regiones de México más importantes en la producción de café, por las 15 900 hectáreas cultivadas (Ramírez Soto et al., 2022; Servicio de Información Agroalimentaria y Pesquera (SIAP), 2022). En Tezonapa, el cafetal cultivado con la especie C. arabica, presenta la característica de ser un sistema de policultivo tradicional, a través de una manipulación del ecosistema forestal nativo con la introducción del café por debajo del dosel de los árboles; está acompañado de numerosas especies de plantas útiles y existe un sofisticado manejo de especies nativas e introducidas (Aguirre-Cadena et al., 2012).
Los estudios previos realizados sobre resiliencia en las zonas cafetaleras cultivadas con C. arabica, se centran en el cambio climático y fenómenos hidrometeorológicos desde la percepción y adaptación del productor. En ese sentido, Viguera, Alpizar, Harvey, Martínez-Rodríguez y Saborío-Rodríguez (2019) determinaron que el 98 % de los productores de café percibieron cambios en el clima y lo asociaron con impactos negativos sobre la producción de café, por lo que el 60 % respondió que realizó prácticas de adaptación mediante el sembrado de árboles y el aumento en el uso de agroquímicos. En Guatemala, en dos paisajes: Acatenango y Chiquimula, el 95 % de los productores percibió cambios en el clima, y el 81 % consideró que tuvo efectos sobre el cafetal y que es necesario incrementar el apoyo técnico, financiero y político (Viguera, Alpízar, Harvey, Martínez-Rodríguez, Saborío-Rodríguez & Contreras, 2019).
Los estudios de resiliencia también han explicado cómo se logra la recuperación a través de los elementos bióticos de la estructura del agroecosistema café después de ser impactado por un huracán de categoría 4, donde el contexto socio-ecológico de los productores influye en la resistencia y, posteriormente, en la resiliencia de cada parcela (Perfecto et al., 2019). Otras estrategias que fomentan la resiliencia en los cafetales, es a través de la adaptación mediante el desarrollo de nuevas variedades híbridas resistentes al clima cambiante, con un aumento en la productividad entre 29 % y 61 %, comparado con variedades tradicionales, pero con la desventaja de presentar una alta demanda de insumos externos como los fertilizantes (Abraha Kashay et al., 2023). La gestión de la resiliencia a nivel local, regional y en el paisaje de las zonas cafetaleras, requiere la intervención política para diversificar las actividades de los productores y materializar el conocimiento sobre la gestión de riesgos (Eakin et al., 2012). Por ejemplo, una dimensión que aumenta el nivel de vulnerabilidad climática es la condición de los campesinos con pobreza, alto grado de marginación y carencia de acceso a servicios públicos (Intergovernmental Panel on Climate Change (IPCC), 2023). En el área de estudio, la producción de café depende de la distribución de la lluvia durante el año y se realiza en un contexto socioeconómico en donde el 75,2 % de la población está en condición de pobreza y con grado de marginación y rezago social alto (Secretaría del Bienestar, 2023), por lo que ante un desastre natural se potencia el desarrollo de un impacto quince veces mayor en comparación con un área no vulnerable al cambio climático (IPCC, 2023).
La importancia del municipio de Tezonapa en la producción de café y el abordaje simplificado de la resiliencia en estudios previos, plantea la necesidad de abordar esta propiedad emergente desde un enfoque holista e integrador, a través de las diferentes dimensiones que aportan a la resiliencia del agroecosistema café (SIAP, 2022). Es importante desarrollar un agroecosistema cafetalero con capacidad adaptativa a los diferentes choques e impactos extremos para mantener su estructura y función (resiliencia). Por ello, es importante responder las preguntas: ¿Qué dimensiones le confieren mayor o menor capacidad de resiliencia al AES-café ante la presencia de diferentes crisis? Es probable que la capacidad de resiliencia del agroecosistema café (CRAC) esté relacionada con la dimensión social, ambiental, económica y tecnológica. La condición actual de homeostasis del AES-café bajo escenarios de cambio climático puede perderse y llevarlo a rebasar el punto de quiebre y alcanzar un estado de no retorno. Por tanto, el objetivo fue determinar la capacidad de resiliencia del agroecosistema cafetalero en la región Centro del estado de Veracruz.
Materiales y métodos
Esta investigación se realizó de diciembre de 2017 a noviembre de 2018 en el municipio de Tezonapa, estado de Veracruz, México (Figura 1), en parcelas cafetaleras distribuidas en zonas de valle y de montaña ubicados en un gradiente altitudinal entre los 100 y 1400 m s. n. m. El tipo de suelo en el municipio corresponde a vertisol para la zona de valle y litosol para la de montaña (Instituto Nacional de Estadística y Geografía (INEGI), s.f.a). El tipo de clima es cálido húmedo en el valle y semicálido húmedo en la montaña (INEGI, s.f.b).
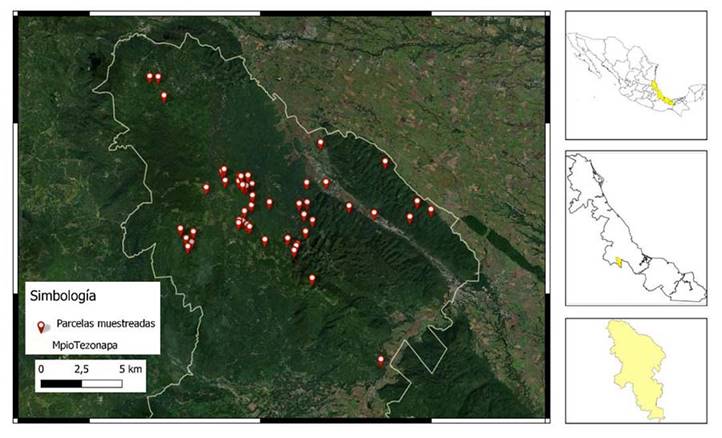
Figura 1 Distribución geoespacial de las fincas del agroecosistema café en el municipio de Tezonapa, Veracruz, México. 2018
Tamaño de muestra
Los productores de café (C. arabica) se contactaron a través de reuniones, realizadas en tres localidades, convocadas por autoridades locales (agente municipal y comisariado ejidal), así como con información proveniente del departamento de Fomento Agropecuario municipal y centros locales de acopio de café (beneficios). Se conformó un padrón de 96 caficultores, de los cuales se localizó y entrevistó a un total de 52 (54 %), cuatro productores más sí se considera una muestra representativa al 10 % de error, incluidos trece productores de la asociación local “Santa Cruz Olintzi”.
Medición de indicadores de capacidad de resiliencia
La información de los indicadores se obtuvo mediante entrevista a productores de café, en donde se utilizó un cuestionario estructurado, que incluyó seis secciones: 1) eje social, 2) eje económico, 3) eje tecnológico, 4) eje comunicaciones, 5) eje institucional y 6) eje ambiental; cada sección contenía cinco variables. El total de variables medidas en cada agroecosistema fue de treinta y se establecieron de acuerdo con la revisión de bibliografía (Acevedo et al., 2005; Akter & Mallick, 2013; Altieri et al., 2015; Altieri & Nicholls, 2013; Cabell & Oelofse, 2012; Centro Nacional de Prevención de Desastres (CENAPRED), 2015; Comisión Económica Para América Latina y el Caribe (CEPAL), 2016; Comisión Nacional de los Salarios Mínimos (CONASAMI), s.f.; Consejo Nacional de Evaluación de la Política de Desarrollo Social (CONEVAL), s.f.; Cutter et al., 2010; Espinoza-García et al., 2016; Food and Agriculture Organization of the United Nations (FAO), n.d.; FAO, 2009; Gliessman, 2002; Hicks Masterson et al., 2014; Jardel Peláez, 2015; Lin, 2011; Peralta Solorio, 2016; Qasim et al., 2016; Rose, 2007; 2009; Salgado García et al., 2013; Schmuck, 2000; Singh et al., 2015; Twigg, 2007).
La segunda etapa consistió en visitar la parcela de cada productor, para recabar datos en campo relacionados con la dimensión ambiental. Cada parcela fue georeferenciada (GPS eTREX®), y configurada en coordenadas geográficas WGS 84-Datum. Para la toma de datos se estableció un conglomerado de muestreo de una hectárea (564 200 m de radio).
Cada conglomerado estuvo constituido por cuatro sitios o subunidades circulares de 400 m2 (radio de 11,283 m) con un punto central en el sitio de muestreo. La distribución de estos sitios corresponde a una “Y” invertida con respecto al norte (N), en la que el sitio 1 representa el centro del conglomerado y los sitios 2, 3 y 4 las unidades de muestreo periféricas. Los sitios 2, 3 y 4 se encuentran a 0°, 120° y 240° de azimut, respectivamente, equidistantes a 45,14 m del centro del conglomerado (Comisión Nacional Forestal (CONAFOR), 2010) (Figura 2). Se tomaron datos de pendiente con un clinómetro digital. Se hicieron observaciones sobre la estructura vegetal de cada parcela y sobre la presencia o ausencia de: erosión laminar o de cárcavas (FAO, 2009), y asocio del cafetal con otros cultivos (García Alaniz et al., 2016).
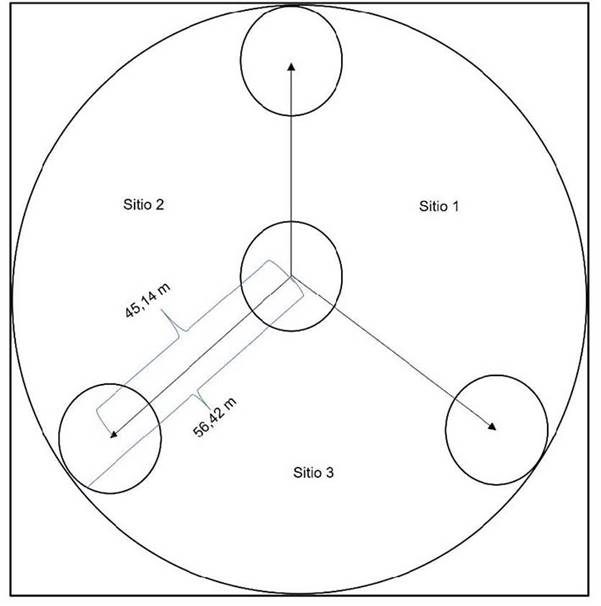
Figura 2 Trazado de cuatro transeptos orientados hacia cada punto cardinal utilizado para medir las características ambientales de cada parcela de café (C. arabica) en Tezonapa, México, 2018.
El muestreo del suelo consistió en tomar diez submuestras por cada hectárea de cada parcela, se tomaron muestras a 30 cm de profundidad. Las submuestras fueron mezcladas, hasta tener 2 kg de suelo que se almacenó en bolsas nylon de color negro. Cada muestra fue enviada al laboratorio para determinar parámetros físicos (textura y densidad aparente) y químicos (macro y micronutrientes). Para el análisis de las muestras de suelo se utilizaron los métodos establecidos en la NOM-021-RECNAT-2000 (Salgado García et al., 2013).
Índice de capacidad de resiliencia
El índice de capacidad de resiliencia se estableció en una escala de 0-1 (Cutter et al., 2010); para ello, se integraron por treinta indicadores. Estos factores fueron normalizados por el método de escalamiento mínimo-máximo (Cutter et al., 2010). Las variables donde los valores fueron más altos correspondieron a bajos niveles de resiliencia, el valor de las variables fue invertido. Los valores cercanos a 1 indican alta capacidad de resiliencia y los valores cercanos a 0 representan nula o baja capacidad de resiliencia (Cutter et al., 2010).
Las variables estudiadas fueron del tipo: nominales dicotómicas (Si= 1; No=0; presencia=1; ausencia =0) y ordinales (Nula= 0; Muy baja= 0,1-0,25 Baja = 0,26-0,49, media= 0,50-0,60, alta= 0,61-0,74, muy alta= 0,75-0,99 y excelente= 1), tal como se muestra en el Cuadro 1 (Infante & Zarate de Lara, 2013). Los valores recabados fueron acoplados con el programa Qgis versión 2.1, para generar un mapa de distribución con la técnica de interpolación espacial Inverse distance weighting.
Cuadro 1 Criterio utilizado para adjudicar valores a los indicadores de la capacidad de resiliencia del agroecosistema café (CRAC) y estandarizar su diversidad en una escala entre 0 y 1 en Tezonapa, México, 2018.
Capacidad de resiliencia | Rango |
Excelente | 1 |
Muy alta | 0,75-0,99 |
Alta | 0,61-0,74 |
Media | 0,50-0,60 |
Baja | 0,25-0,49 |
Muy baja | 0,1-0,24 |
Nula | 0 |
Análisis estadístico
Los datos fueron analizados mediante estadística descriptiva (tendencia central), análisis de frecuencias y nominales. Las diferencias entre la frecuencia de respuestas de variables con mayor y menor aporte a la capacidad de resiliencia de los indicadores ordinales fueron analizadas mediante el estadístico de X2 (Chi-cuadrada) y las respuestas de variables dicotómicas mediante la prueba estadística de Cochram (Infante & Zarate de Lara, 2013).
Los valores del índice de capacidad de resiliencia, para cada variable, fueron promediados según el factor al que cada una corresponde. Para determinar diferencias entre el aporte de factores a la capacidad de resiliencia se utilizó la prueba del signo (Infante & Zarate de Lara, 2013). Los valores de capacidad de resiliencia de cada parcela fueron clasificados según la altitud en alta: media y baja (World Coffee Research, 2023). El estadístico de Kruskal-Wallis se utilizó para determinar diferencias de capacidad de resiliencia en las parcelas (Infante & Zarate de Lara, 2013). El software estadístico utilizado fue Statistica® 7.0.
Resultados
Contribución de los indicadores a la capacidad de resiliencia
Entre las variables en escala ordinal que tuvieron mayor frecuencia positiva (Figura 3) y que aportaron a la CRAC, sobresalieron los correspondientes a la dimensión ambiental, entre ellos la estructura vegetal, por la presencia de una asociación entre cafetos con herbáceas-arbustos-árboles, esto como resultado de la necesidad de sombra de los cafetos. La calidad del suelo, respecto a otras variables, presentó diferencias significativas (X2=86,316 P<0,005), debido a que los parámetros del suelo, en promedio, presentaron valores altos, el nitrógeno total alto (0,255 ±0,033), fósforo asimilable alto (11,604 ±31,020), materia orgánica alta (9,929 ±16,773) pH moderadamente ácido (6,289 ±0,621), conductividad eléctrica muy ligeramente salina (0,128), capacidad de intercambio catiónico alta (25,144 ±9,882), hierro intercambiable adecuado (36,840 ±17,485), cobre adecuado (0,804 ±0,817), zinc adecuado (11,447±588,954) y manganeso adecuado (24,17±18,755). El 83 % de las parcelas contaba con una estructura del estrato arbóreo, arbustivo y herbáceo asociado con los cafetos. EL 11 % presentó la asociación cafetos-herbáceas, el 4 % solo cafeto y cafetos-árboles 2 %.
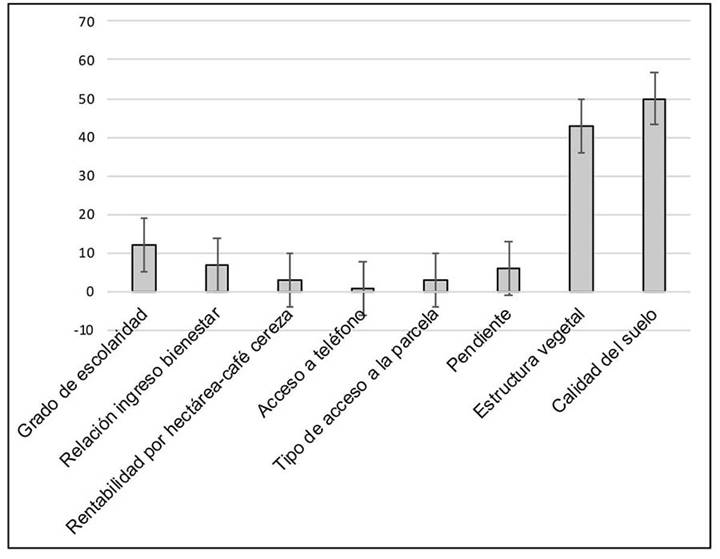
Figura 3 Frecuencia de variables ordinales positivos para la capacidad de resiliencia de 52 fincas cafetaleras de Tezonapa, México, 2018.
Con relación a variables (en escala ordinal) negativas o con menor aporte a la CRAC sobresalieron: acceso a la parcela, pendiente del terreno, relación ingreso-bienestar del productor, grado de escolaridad y rentabilidad por hectárea, los cuales presentaron diferencias significativas (X2=101,676 P<0,005) respecto a las variables acceso a telefonía celular y estructura vegetal (Figura 4).
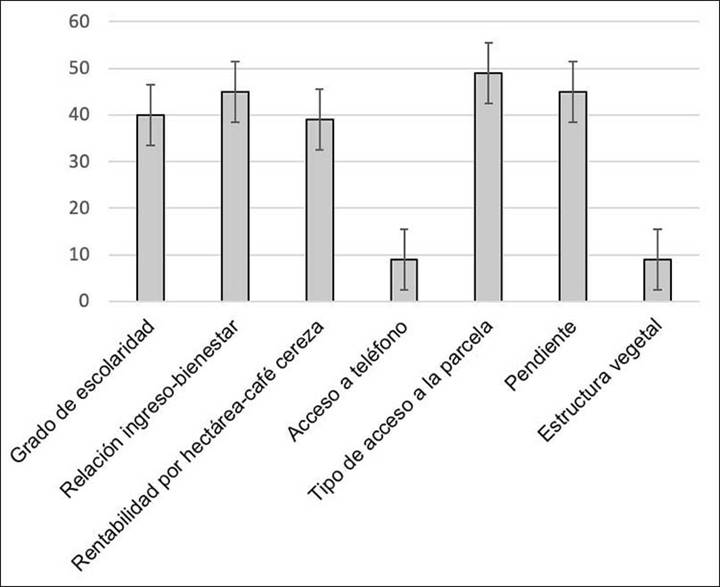
Figura 4 Frecuencia de variables ordinales negativos para la capacidad de resiliencia de 52 fincas cafetaleras de Tezonapa, México, 2018.
Los valores negativos restan aporte al valor general de capacidad de resiliencia, tal es el caso de la variable acceso a la parcela, cuya condición fue principalmente camino de terracería, seguido de sendero empedrado. Los niveles de pendiente fueron mayores a 14 %, por lo que el terreno se consideró fuertemente inclinado. En la variable ingreso-bienestar, se reflejaron ingresos económicos mensuales entre 0 y USD$ 58,08 como resultado de la cafeticultura. La rentabilidad de café cereza fue baja para el 75 % de los productores, debido a que se encontró por debajo de la línea de pobreza por ingresos del CONEVAL (s.f.). Con relación al grado de escolaridad, el 15 % de los productores presentaron un nivel nulo o 0 años de estudio, el 61 % con un nivel entre1 a 6 años, el 19 % con un rango medio entre 7 a 12 años y el 4 % con un nivel alto con más de 13 años de estudio.
Respecto a las variables con respuesta dicotómica y con mayor cantidad de frecuencia de respuestas positivas sobresalieron: acceso a servicios de salud, donde un 98 % de los cafeticultores contaban con acceso a servicios médicos, el 98 % de los entrevistados consideró que el origen de los fenómenos naturales es no causado por una divinidad existe la presencia de un atlas de riesgo, instituciones de protección civil y un plan estratégico de protección civil para toda la zona de producción de café en el municipio (Figura 5).
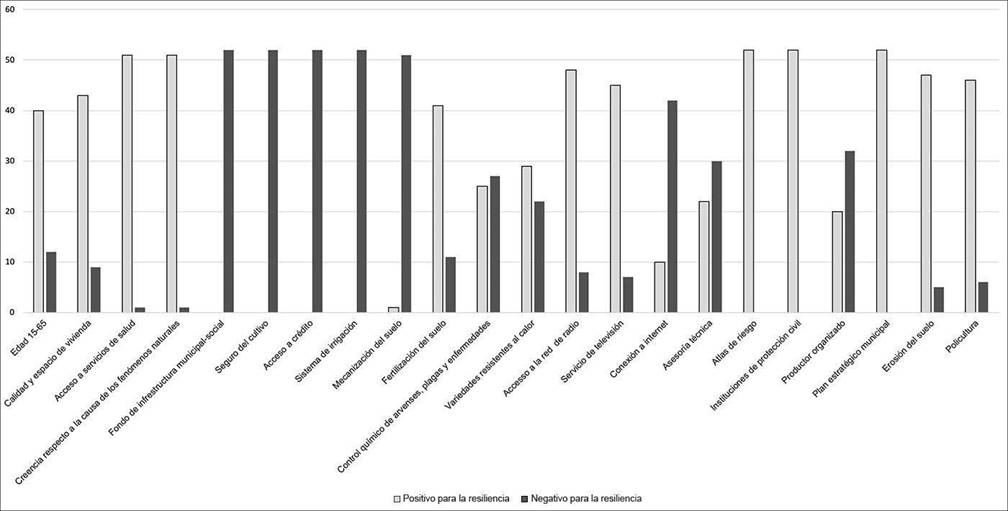
Figura 5 Frecuencia de variables con respuesta dicotómica positiva y negativa para la capacidad de resiliencia de 52 fincas cafetaleras (C. arabica) en Tezonapa, México. 2018.
Entre las variables negativas con respuesta dicotómica se encontraron: aseguramiento del cultivo y acceso a crédito, donde ambas variables estuvieron ausentes y son parte de la dimensión económica, por lo que son poco probables de controlar debido a la baja rentabilidad de la cosecha del café a nivel parcela. El 81 % de los productores contaba con conexión de internet a través de la red de telefonía celular. El 62 % de los productores no estaba organizado, el 57 % no contaba con asesoría técnica. El 25 % realizaba control químico de arvenses, el 22 % no contaba con variedades resistentes al calor.
Índice de capacidad de resiliencia
La valoración de la capacidad de resiliencia del agroecosistema café, mostró que los indicadores con mayor aporte se agruparon principalmente en indicadores de la dimensión social (80 %), ambiental (80 %), institucional (60 %) y de comunicaciones (60 %). Por el contrario, los indicadores que aportaron menos a la capacidad de resiliencia se agruparon en las dimensiones tecnológica (80 %) y económica (100 %), esta última presentó diferencias estadísticamente significativas (p<,00000) respecto al resto de las dimensiones (Cuadro 2).
Cuadro 2 Comparación de medias entre los factores integradores de la capacidad de resiliencia del agroecosistema café (C. arabica) en Tezonapa, México. 2018.
Factor | Media | Desviación estándar |
Social | 0,783 | 0,152 |
Económico | 0,065 | 0,132 |
Tecnológico | 0,226 | 0,168 |
Comunicaciones | 0,430 | 0,176 |
Institucional | 0,761 | 0,168 |
Ambiental | 0,741 | 0,195 |
Distribución geoespacial de la capacidad de resiliencia del agroecosistema cafetalero
De las 52 fincas, veintiocho (53 %) presentaron una capacidad de resiliencia baja, dieciocho (34 %) capacidad media y seis (11 %) capacidad alta. Se encontró una tendencia de las fincas con CRAC alta a encontrarse a una altitud menor y a medida que aumentaba la altitud de donde se encontraban las fincas de la CRAC fue menor. Es probable que la altitud y lo abrupto del relieve montañoso limiten el acceso de los productores a servicios públicos y productivos diversos. Se encontraron diferencias significativas entre los valores medios del índice de CRAC (prueba de F P<0,05), distribuidos según la clasificación de altitud óptima para el cultivo de café (World Coffee Research, s.f.), donde existen tres categorías: altitud baja, media y alta. Las fincas ubicadas a una altitud baja presentaron mayor CRAC con diferencias significativas respecto a la CRAC de fincas localizadas a una altitud media y alta (Figura 6).
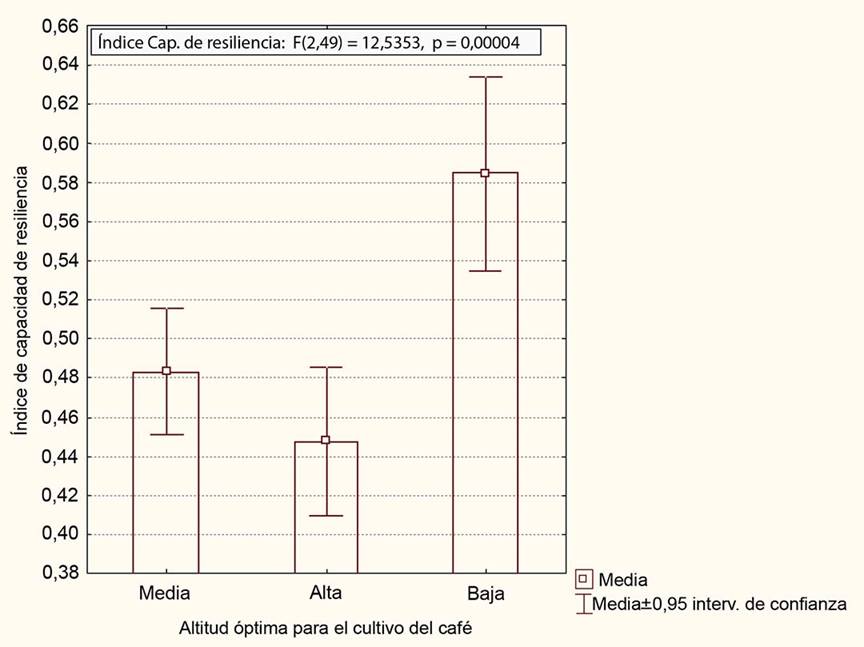
Figura 6 Comparación entre la capacidad de resiliencia del agroecosistema café (C. arabica) (CRAC) de las fincas localizadas en tres categorías altitudinales en Tezonapa, México, 2018.
El grupo de fincas de café con capacidad de resiliencia alta presentaron valores de CRAC entre 0,62 y 0,73, las fincas con CRAC media presentaron valores entre 0,50 y 0,60. Las fincas con CRAC baja entre 0,28 y 0,49 (Figura 7).
Discusión
Los indicadores de resiliencia estudiados en las parcelas de café, presentaron valores que expresan fortalezas y debilidades. La alta calidad del suelo está relacionada con el ciclo continuo de desechos orgánicos provenientes de la vegetación utilizada como sombra o sotobosque para los cafetales (Rodríguez Suárez et al., 2019). El flujo constante de material orgánico permite que en los parámetros del suelo se hayan presentado valores positivos, lo que coincide con los resultados de otros estudios como el de Jurburg et al. (2020), quienes en diecinueve plantaciones de café determinaron valores altos de nitrógeno y carbono, así como una actividad alta de las poblaciones de bacterias y hongos, los cuales están asociados a los aportes constantes de materia orgánica.
La mayor parte de las parcelas muestreadas presentaron una estructura con árboles-arbustos-herbáceas, los cuales están asociados a los cafetos, con lo que se promueve la diversidad biológica, que es un elemento importante para la resiliencia en sistemas socioecológicos y agroecosistémicos (Cabell & Oelofse, 2012; Lin, 2011). La biodiversidad que integra a los cafetales de estudio puede ser organizada para su análisis en diversas jerarquías tales como: a nivel de acervo genético, de paisaje y de cultivos agrícolas. Estas características le dan autonomía al agroecosistema frente a insumos externos y, en caso de una crisis o ante un evento climático extremo, le permite al productor tener opciones para una recuperación rápida después de los daños sufridos (Kliem & Sievers-Glotzbach, 2022).
El manejo del cafetal por parte de los productores incluye la realización de podas, cuyos restos, más los provenientes de la vegetación circundante, se integran al suelo, por lo que resulta en un efecto positivo directo generado por el manejo agroecológico, basado en la diversificación vegetal sobre los parámetros físicos, químicos y biológicos de la calidad del suelo (Mancini Texeira et al., 2021). Las labores de mantenimiento del cafetal de forma manual y la ausencia de maquinaria agrícola pesada, favorecen a que el suelo no se compacte y se estimule el desarrollo radical de las plantas y el intercambio gaseoso e hídrico. Se ha identificado que el proceso de degradación de la hojarasca se acelera y es particular para los cafetales, debido a la presencia de poblaciones microbianas propias del agroecosistema café (Schmitt & Perfecto, 2021). La presencia de árboles, arbustos y herbáceas, en las parcelas de estudio es positiva y son un factor importante, debido a que se ha determinado que especies asociadas a los cafetales benefician a parámetros como el fósforo y la materia orgánica (Getachew et al., 2023).
Las prácticas agronómicas actuales como la integración de residuos de la poda, el no uso de maquinaria agrícola, o la siembra manual de los cafetos, cuyas labores son realizadas por los productores de las parcelas muestreadas, podrían mejorarse con otras técnicas como el establecimiento de terrazas, la aplicación de bioinsumos, la siembra de arvenses fijadoras de nitrógeno y el aumento de las especies de flora local aprovechables. Además del mejoramiento de las prácticas agronómicas actuales, es importante integrar nuevos enfoques como el del sistema de intensificación de cultivos, el cual está diseñado para los productores con recursos limitados o aquellos cuyos cafetos presentan deficiencias nutricionales (Silici et al., 2011). Existen acciones sencillas aplicables en los cafetales de estudio que fomentan la adaptación del agroecosistema café ante un escenario de cambio, estas consisten en: planta de café de alta calidad, espacio o distancia óptima entre las plantas, mantenimiento de la capa superior del suelo, aireación del suelo alrededor de las plantas, drenaje para evitar que el suelo se sature de agua y se presenten condiciones hipóxicas, enmienda del suelo con materia orgánica y reducción de la dependencia de fertilizantes inorgánicos y plaguicidas, a través del uso de bioinsumos tanto como sea posible (Silici et al., 2011).
La falta de rentabilidad del café cereza en las parcelas de estudio está asociada al precio del mercado y se refleja en variables con valores negativos para la resiliencia como: relación ingreso-bienestar del productor, grado de escolaridad y rentabilidad por hectárea, los cuales son reflejo de una situación precarizada que es común en pequeños productores de café de la región y de México. En algunos casos, los costos de producción por hectárea son mayores (US$ 24 209) comparados con la ganancia por la misma superficie (US$ 11 801). Lo anterior limita el desempeño para mitigar de forma adecuada los problemas de producción y aprovechar las oportunidades que el mercado ofrece al momento de la comercialización (Campbell, 2021).
La necesidad socio-económica de los productores propietarios de las parcelas de estudio, tienen alternativas viables de mejora a través del valor agregado como la certificación en producción de café orgánico de exportación y la venta de café tostado y en bebida, a través de la integración de organizaciones de pequeños productores, lo cual les permite aumentar el precio del grano (Campbell, 2021).
Una de las causas de la falta de rentabilidad del café en Tezonapa, se debe a que es frecuente que los pequeños productores se mantienen en las primeras etapas de la cadena productiva y desempeñan las tareas más laboriosas y menos rentables con la consecuencia de una limitada (Campbell, 2021) inversión en tecnología agrícola, infraestructura de comunicaciones o capacitación, que permita la innovación requerida para enfrentar futuras condiciones de crisis (Cabell & Oelofse, 2012). La dimensión tecnológica es crucial para mejorar la productividad y aumentar las medidas de adaptación de las fincas ante escenarios de cambio climático (Bedeke et al., 2020; Nyberg et al., 2021; Roy et al., 2019).
Los resultados obtenidos mostraron que la ubicación remota de los cafetales limita el acceso a los recursos institucionales y complica la venta de la producción de café (Pérez, 2021). Para el caso de las variables acceso a la parcela y pendiente del terreno, el resultado obtenido se debe a que las parcelas muestreadas se encontraban en una zona rural y montañosa, en donde lo accidentado del relieve dificulta dar mantenimiento a la infraestructura de acceso, por lo que es frecuente encontrar pequeñas veredas en donde solo puede transitar un arriero y el animal de carga. En los cafetales cercanos a centros económicos se facilita el acceso a los recursos institucionales y su ubicación se asocia a terrenos planos, lo que representa una ventaja para innovar y adaptarse a escenarios de crisis (Hausermann, 2014; Leyva Mir, 2010).
El relieve abrupto de la zona de estudio presenta pendientes pronunciadas que limita el acceso a servicios y las labores básicas de agricultura como la mecanización del suelo, la densidad de siembra o el acceso para riego y sacar la cosecha (Morales-Hernández, 2015; Simane et al., 2013). Los productores con resiliencia del agroecosistema café (CRAC) alta, presentaron mayor conectividad con el exterior, al tener un promedio de cuatro medios de comunicación. Por el contrario, los administradores con CRAC media y baja, presentaron en promedio dos dispositivos de comunicación. Sin embargo, el 80 % de los productores no tiene conexión a internet, lo que incrementa la brecha digital en el contexto cafetalero, por lo que se debe impulsar la conectividad mediante el desarrollo económico local, ya que a mayor nivel de pobreza las posibilidades de acceso y uso tecnológico disminuyen (Gómez Navarro, 2019). El acceso internet permite acceder a información importante para la prevención de desastres o daños por plagas, pero es necesario que los productores desarrollen competencias digitales para acceder a sitios web y aplicaciones de uso agronómico, meteorológico y de prevención de desastres (Organista et al., 2016).
La orografía en donde se distribuyen las 52 parcelas influye sobre la marginación y ocasiona rezago social, económico, de infraestructura y tecnológico en los productores y en la población (Morales-Hernández, 2015). Las características orográficas de las parcelas de estudio son escarpadas, por lo que se consideran de difícil acceso, lo que dificulta y limita el proceso de producción y comercialización del cultivo de café y, en consecuencia, ocasiona menor CRAC comparado con los agroecosistemas en zona de valle.
Los valores determinados en la dimensión social de los productores cafetaleros, podrían contribuir a una recuperación lenta después de un evento de desastre tal como se ha documentado en comunidades con baja capacidad de resiliencia, en donde variables como: creencias religiosas frente a inundaciones, población sin ingresos para cubrir necesidades básicas y población con discapacidad, presentan un aporte sobresaliente en el aumento de la vulnerabilidad local ante un desastre natural (Qasim et al., 2016).
Los productores de café entrevistados presentaron un nivel de educación bajo, que limita la innovación tecnológica, la adaptación y, por consecuencia, hace más lenta la recuperación después de un desastre como los relacionados al cambio climático (Silici et al., 2011). En los pequeños productores de café, la baja escolaridad se asocia con otras razones diversas como la falta de dinero, el desconocimiento de nuevas técnicas agronómicas y el nulo acceso a mano de obra, que en conjunto limitan la utilización de medidas de adaptación respecto a los cambios ambientales (Nyberg et al., 2021).
La transferencia mejorada de conocimientos agrícolas es una vía clave para promover la sustentabilidad de la productividad de los pequeños agricultores, a través de la intensificación sostenible que promueve la conservación de los recursos naturales (Nord et al., 2022). Sin embargo, el extensionismo no ha sido suficiente para satisfacer la demanda de conocimiento por parte de los productores de café, lo que ha resultado en una limitante para desarrollar un agroecosistema de café más sostenible; lo anterior destaca la necesidad de integrar los servicios de asesoramiento agrícola gubernamentales, no gubernamentales y del sector privado, enfocados en promover estrategias de adaptación bajo escenarios de cambio (Nord et al., 2022).
Los valores negativos de algunos indicadores restan capacidad de resiliencia al AES-café (Cabell & Oelofse, 2012), esta característica se registró también con personas de comunidades poco resilientes a desastres naturales (Kusumastuti et al., 2014). Sin embargo, los valores negativos encontrados en los indicadores y ante una situación de crisis son una oportunidad para moderar los efectos negativos o para tomar provecho de ello, lo que podría motivar a los productores de café a desarrollar una diversidad de actividades de adaptación, que les permita superar retos y no rebasar el punto de quiebre del agroecosistema para mantenerse ante el escenario de cambio, tal como ha sucedido en agroecosistemas de maíz y de pastoreo (Titonell, 2013).
A escala regional, la CRAC no se distribuyó de forma homogénea en las tres categorías de altitud. Se puede considerar que el relieve (a escala regional), mediante la altitud, ejerce un efecto sobre los indicadores del agroecosistema. Lo anterior, tiene como consecuencia un efecto en cascada (Rocha et al., 2018) sobre las dimensiones o factores en las que se integran los indicadores y estas dimensiones a su vez influyen en la distribución de la capacidad de resiliencia.
Las fincas de café del presente estudio, se encuentran en una región en donde la gestión del riesgo y la prevención de desastres están presentes porque existe un atlas de riesgo, un plan de protección e instituciones coordinadas desde el enfoque de la protección civil de los tres niveles de gobierno que rigen en México. Los desastres naturales como huracanes, incendios, deslaves, inundaciones, sequías y ondas de calor, han afectado al ser humano tanto en las zonas urbanas como las rurales y es en estas últimas, en donde los estragos causados ponen en riesgo la cosecha, la derrama económica por la venta agrícola y la soberanía alimentaria de los productores, ahí la importancia de realizar gestión del riesgo ante desastres (Campbell, 2021; Chapagain & Raizada, 2017).
Los resultados negativos relacionados al aseguramiento del cultivo y acceso a crédito, son un área de oportunidad para mejorar en el agroecosistema café, debido a que se ha encontrado beneficios al corto y largo plazo por el uso de créditos, los cuales pueden aumentar la productividad del sector agrícola tal como se ha documentado en África (Aloysius Ngong, 2022). Otra ventaja del acceso a crédito es que ante un daño ocasionado por un desastre, permite recuperar lo perdido de una forma acelerada, pero también es importante que el valor de la producción del cafetal permita sostener los pagos del crédito, sobre todo ante la tendencia de descenso del precio del café como ha ocurrido desde 2011 (Aloysius Ngong, 2022; Rodríguez Toledo et al., 2019), lo que ha generado que gran parte de la contratación de seguros dependa significativamente del respaldo de los recursos públicos (Solano-Alonso et al., 2021).
Conclusiones
Los indicadores que menos aportaron a la capacidad de resiliencia del agroecosistema café (CRAC) y que presentaron valores negativos, fueron los relacionados con los factores económico y tecnológico. Es necesario, vía productor, estimular la homeostasis del AES-café y fortalecer las debilidades detectadas es estos dos ejes, ya que el agroecosistema podría alcanzar y rebasar su límite de resistencia y migrar a una condición de no retorno. La dimensión comunicaciones presenta potencial para aumentar su aporte a la CRAC y sobresale el uso de teléfonos con acceso a internet, lo que permite al productor tener acceso a información para mejorar la toma de decisiones. Por el contrario, las dimensiones más fortalecidas fueron: la ambiental, la cual está relacionada al manejo agroecológico del cafetal bajo sombra, y la social e institucional, las cuales se asocian con la presencia de programas de gobierno municipal, estatal y federal.
Los tres niveles de CRAC de las fincas muestreadas se distribuyeron en forma diferente sobre el gradiente altitudinal del área de estudio. Debido a lo anterior, existe la tendencia de que las fincas con índice de resiliencia alta se distribuyan en zonas con baja altitud, conforme se asciende se encontraron fincas con un índice de resiliencia media y en las zonas con mayor altitud las fincas tuvieron un índice bajo. Lograr la resiliencia del sector agrícola y de los agroecosistemas en particular de los cafetaleros, es y debe ser un objetivo importante para el sector agrícola en México ante la incertidumbre de fenómenos ambientales, sociales y económicos causales de crisis.