Introducción
El panorama actual de calentamiento global, ha generado una extrema variabilidad en el comportamiento del clima, lo cual ha causado cambios en la cantidad y distribución históricas de lluvias en las diferentes regiones del mundo (Hitz & Smith, 2004). Este fenómeno ha traído como consecuencia mayor ocurrencia y severidad de situaciones de estrés por déficit hídrico en el desarrollo de cultivos, aspecto que ha adquirido especial importancia a nivel mundial (Zoebl, 2006).
Se pronostica que en países desarrollados habría una reducción en la producción y rendimientos de Solanum sp. de hasta un 30 %, si no se desarrollan e implementan estrategias de manejo agronómicas enfocadas en la mitigación y adaptación a condiciones climáticas extremas. En cuanto a países en vías de desarrollo, las altas temperaturas y sequías prolongadas han causado pérdidas en 130 000 ha y se diagnostica que la productividad disminuiría en un 50 % (Hijmans, 2003).
A nivel fisiológico, uno de los principales efectos reportados bajo condiciones de estrés hídrico por deficiencia de agua es la afección en la fotosíntesis (Ierna & Mauromicale, 2006; Ramírez et al., 2016; Schapendonk et al., 1989). Un bajo contenido relativo de agua de las hojas, activan cadenas de señalización que aumentan los niveles de ácido abscísico, lo que induce el cierre de estomas como defensa contra la perdida de agua en la planta (Lim et al., 2015), por medio de la reducción de la transpiración, lo cual trae como consecuencia bajas tasas de absorción de CO2 y de formación de fotoasimilados, además de una menor acumulación de materia seca y rendimiento (Lahlou et al., 2003; Tourneux et al., 2003).
En cuanto a las limitaciones no estomáticas, las principales consecuencias son el detrimento de la membrana celular y los cloroplastos, diminución de la concentración de clorofila y afección en el funcionamiento del fotosistema, a causa de la reducción de la fotólisis del agua y menor tasa de transferencia de electrones (Casierra-Posada, 2011; Coleman, 2008; Deeba et al., 2012; Ghobadi et al., 2013; Noctor et al., 2014).
Para la experimentación frente al déficit hídrico (DH) en el cultivo de papa, se ha realizado el uso de diferentes metodologías, entre las más comunes están las evaluaciones bajo condiciones de campo, con tratamientos bajo riego y sin riego y, en ciertas ocasiones, protegidos por techos fijos o móviles que permiten la eliminación parcial o total de las lluvias. Otra estrategia, es la realización de estudios bajo invernaderos y uso de soluciones hidropónicas con diferentes potenciales osmóticos (Fernández, 2010). Para la definición del momento de mayor interés en la aplicación de tratamientos para estudios de respuesta al estrés por sequía, se consideran los periodos de emisión y llenado de tubérculos, ya que son las fases del cultivo de papa de mayor sensibilidad que afectan el rendimiento (Mane et al., 2008).
El estrés por déficit hídrico se conjuga por la interacción entre la intensidad, que hace referencia a la fuerza con que se presente, duración, la cual es el tiempo que está el cultivo bajo condiciones de déficit hídrico, genotipo, que se relaciona con la capacidad que ha desarrollado cada cultivar para adaptarse, y la fase fenológica, en la cual se da la incidencia del estrés. La interacción de estos factores define el nivel de estrés (leve moderado e intenso), y según este nivel son las respuestas fisiológicas y las implicaciones en el rendimiento del cultivo (Liu et al., 2005; Parent et al., 2009).
El estrés por déficit hídrico se suele acompañar de estrés por altas temperaturas, las cuales generan cambios anatómicos y funcionales similares a los producidos por el estrés por sequía: como el cierre estomático, disminución del tamaño celular y vasos xilemáticos, reducción en la permeabilidad y estabilidad de las membranas, aumento de la densidad estomática (Chaves-Barrantes & Gutiérrez-Soto, 2017), disminución en la concentración de RuBisCO y su afinidad por el CO2 (Bae & Sicher, 2004); por consiguiente, se promueve su actividad oxigenasa, con producción de H2O2, el cual puede ser tóxico para las células vegetales (Song et al., 2014).
La respuesta de adaptación fisiológica puede variar entre genotipos y niveles de estrés, el objetivo del presente trabajo fue identificar los niveles de respuesta fisiológica que presenta Solanum phureja bajo el estrés progresivo por déficit hídrico.
Materiales y métodos
Ubicación del estudio
El experimento se desarrolló entre noviembre de 2019 y marzo de 2020, en el centro de investigación Obonuco de la Corporación Colombiana de Investigación Agropecuaria (AGROSAVIA), en la zona rural del municipio de Pasto, departamento de Nariño, Colombia, a una altura de 2756 m s. n. m., 01° 11’ 28,3” latitud norte y 77°19’ 08,8” longitud oeste; bajo condiciones semi-controladas de invernadero, donde se controló la humedad del suelo por efecto de la cubierta y el riego por goteo, los demás factores atmosféricos no presentaron control, por lo tanto, la temperatura promedio que presentó el invernadero fue de 16,15 °C y la humedad relativa de 78 % (Figura 1).
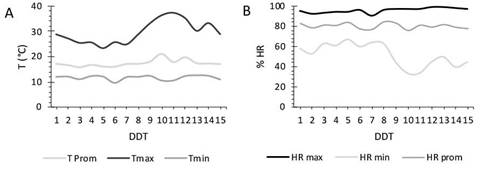
Figura 1 Condiciones de temperatura (A) y humedad relativa (B), bajo condiciones semi-controladas, durante el periodo de evaluación de la respuesta fisiológica de Solanum phureja, en el Centro de Investigación Obonuco, de la Corporación Colombiana de Investigación Agropecuaria, Pasto, Nariño, Colombia, 2019-2020.
Material vegetal
Se evaluaron cuatro genotipos de la especie Solanum phureja, 1) Criolla Colombia es el más cultivado de la especie y mayor avance genético, 2) Ratona Morada, un cultivar nativo utilizado para la seguridad alimentaria de la agricultura familiar en Nariño, 3) Mambera y 4) Morasurco (Figura 2). Cultivares intermedios entre su mejora genética y siembra con fines comerciales y alimentación familiar (Tinjacá Ruiz & Rodríguez Molano, 2015). Las muestras fueron recolectadas bajo el permiso Marco de recolección conferido a AGROSAVIA, bajo la resolución No 1466 del 03 de diciembre de 2014 de ANLAS (Colombia).
Diseño experimental
Los tratamientos se distribuyeron bajo un diseño de bloques al azar en parcelas subdivididas, para la siembra se seleccionaron tubérculos homogéneos en su tamaño, se utilizó 9 kg de suelo seco por macetero al momento de la siembra y luego se realizó un aporque, el cual consistió en agregar 3 kg de suelo a los 20 días después de emergencia, hasta completar 12 kg por balde de suelo seco. La fertilización se realizó con la mezcla: 60 (nitrógeno)- 150 (fósforo)- 60 (potasio) kg ha-1, la cual se aplicó a una dosis de 23 g de fertilizante por planta. Para el manejo de plagas y enfermedades, se realizaron monitoreos periódicos y se controló en todos los tratamientos.
Los tres factores de evaluación se distribuyeron de la siguiente manera: la parcela hace referencia a seis momentos de evaluación en días después de tuberización (DDT), los cuales corresponden al avance en la intensidad del estrés dado por el agotamiento progresivo de la humedad del suelo y las condiciones climáticas presentes en cada evaluación; la subparcela obedece al nivel de riego, el cual se dividió en plantas control y plantas con déficit hídrico; y la sub-subparcela fueron los cultivares. Se establecieron cuatro repeticiones por tratamiento en evaluación, con 32 sub-sub parcelas, las cuales se conformaron por 18 plantas (Cuadro 1).
Cuadro 1 Factores de evaluación de la respuesta fisiológica de Solanum phureja, establecidos bajo invernadero en el Centro de Investigación Obonuco, Corporación Colombiana de Investigación Agropecuaria. Pasto, Nariño, Colombia, 2019-2020.
Factores de evaluación | ||
Momento de evaluación (DDT*) | Nivel de riego | Cultivar |
Seis niveles (0,3,6,9,12,15) | Plantas con riego o control y plantas sin riego o déficit hídrico | Cuatro niveles (Criolla Colombia, Mambera, Morasurco y Ratona mora) |
*DDT: días después de tuberización. / *DDT: Days after tubering.
El control de la humedad se realizó mediante reflectometría de dominio temporal (TDR, por sus siglas en inglés), con el uso del equipo HH2 (Delta-T). Para la definición de los tratamientos de humedad del suelo se siguió como guía las metodologías implementadas por varios autores en estudios similares (Ariza Acevedo, 2017; Díaz-Valencia, 2016; Hernandez et al. 2016; Pino, 2016; Rodríguez Pérez, 2015).
Se definió agotar la humedad volumétrica (θ), a partir del inicio de la tuberización hasta que las plantas presentaran síntomas visuales de estrés intenso (pérdida alta de turgencia en las hojas, decaimiento de las plantas, cierre estomático), esto ocurrió al alcanzar un 10 % de humedad volumétrica del suelo, después de doce días de agotamiento de humedad. Las evaluaciones se realizaron cada tres días, transcurrido este tiempo se efectuó un riego de recuperación para evaluar a los quince días si se presentaban daños en el fotosistema (Cuadro 2).
Cuadro 2 Condiciones de humedad de suelo en plantas con riego y bajo agotamiento de humedad, en cuatro cultivares de papa de la especie Solanum phureja, bajo condiciones semi-controladas, en el Centro de Investigación Obonuco, Corporación Colombiana de Investigación Agropecuaria, Pasto, Nariño, Colombia, 2019-2020.
Días después de tuberización (DDT) | Nivel de riego | |
Plantas con riego Control (θ %) | Plantas sin riego* Déficit hídrico (θ %) | |
0 | 29 | 31 |
3 | 33 | 25 |
6 | 30 | 20 |
9 | 28 | 15 |
12 | 34 | 10 |
15 | 32 | 32 |
* Se agotó la humedad a partir del inicio de la tuberización hasta que las plantas presentaran síntomas visuales de estrés. / *Moisture was depleted from the onset of tuberization until the plants exhibited visual signs of stress.
Para los tratamientos control se aplicó el riego según las lecturas del TDR, el volumen de suelo por macetero y las condiciones climáticas, con el objetivo de mantener la humedad volumétrica del suelo entre 25 a 35 % (capacidad de campo) (Ariza Acevedo, 2017; Díaz Valencia, 2016; Hernandez et al., 2016; Pino, 2016; Rodríguez Pérez, 2015).
Variables evaluadas
Para las variables correspondientes a intercambio gaseoso, se evaluó la tasa de fotosíntesis neta (A) en µmol CO2 m-2 s-1, conductancia estomática (gs) en mol H2O m-2 s-1, transpiración (E) en mmol H2O m-2 s-1, carbono intercelular (Ci) en vpm y temperatura de la hoja (Tleaf) en °C, para esto se utilizó el intercambiador de gases (IRGA) modelo LC-SD (ADC ADC BioScientific Limited), según metodologías reportadas en la literatura (Ariza-Acevedo, 2017; Díaz Valencia, 2016; Pino, 2016; Rodríguez Pérez, 2015).
Para las evaluaciones se tomaron cinco plantas al azar por parcela, sin tener en cuenta los bordes, y se evaluó en el tercer foliolo funcional entre las 9 y 11:30 horas cada tres días después de inicio de tuberización, fue necesario la simulación con luz artificial generada por el IRGA y una densidad de flujo fotónico fotosintético de 1200 PAR, µmol m-2 s-1, la cantidad de luz simulada se definió por medio de una curva de luz realizada con el mismo equipo (Rodríguez-Pérez et al., 2017).
Para el cálculo de la eficiencia intrínseca en el uso del agua (WUEI) en µmolCO2/mol H2O, se dividió la tasa de fotosíntesis neta A entre la conductancia estomática (gs) (Medrano et al., 2007).
La evaluación de la clorofila (Chl) en mg m-2, se realizó basada en metodologías reportadas para la utilización del medidor de contenido de clorofila CCM-300 (ADC BioScientific), se evaluaron cinco plantas por parcela sobre un foliolo funcional, con la misma frecuencia de las evaluaciones de intercambio gaseoso (Ariza Acevedo, 2017; Díaz Valencia, 2016; Pino, 2016; Rodríguez Pérez, 2015).
La eficiencia fotosintética (Fv/Fm) se evaluó en la misma ocasión en que se midió el intercambio gaseoso, donde se tomaron tres plantas por parcela, se evaluó en el tercio superior de cada planta en un foliolo funcional, el cual primero se sometió a oscuridad por 45 min, para luego realizar la medición con un fluorómetro modulado (OS-30p+ Chlorophyll Fluorometer- ADC BioScientific), que excita las moléculas de Chl durante 0,80 s con 1,500 μmol m-2 s-1 de luz actínica (Ariza Acevedo, 2017; Diaz Valencia, 2016; Pino, 2016; Rodríguez Pérez, 2015).
Análisis de los datos
La información obtenida se analizó a través del software R V.4.1.3, con los paquetes Agricolae, ggplot2 y ggpubur. Se realizó la verificación de supuestos donde se identificó la presencia de valores atípicos extremos, distribución normal de los datos, a través del estadístico de Shapiro-Wilk (p>0,05), gráficos QQ normal y homogeneidad de varianzas a través del estadístico de Levene (R Core Team, 2020).
Las variables cuantitativas se analizaron a través de un ANDEVA para un diseño en bloques al azar en parcelas subdivididas, a través de modelos lineales generalizados, acompañado de la prueba de comparación de medias de Tukey (p<0,05). Para aquellas variables que no se presentaron diferencias estadísticas entre las interacciones de tres y dos factores, se analizó el efecto de los factores principales por separado.
Resultados
En la interacción entre los tres factores de evaluación (nivel de riego* momento* cultivar), se presentaron diferencias significativas en la temperatura de la hoja (Tleaf) con Pr(>F) 2,87e-05, la conductancia estomática (gs) con Pr(>F) 2,33e-08, la transpiración (E) con Pr(>F) 0,0010 y la eficiencia fotosintética Fv/Fm con Pr(>F) 0,001398.
Fue posible evidenciar que entre los días 9 y 12 DDT, se presentaron las temperaturas ambiente más altas durante la evaluación. Para Tleaf los mayores valores se dieron en el día 9 (DDT) en plantas con riego, donde Ratona Morada alcanzó 33,93 °C y Mambera 32,98 °C; mientras que para plantas sin riego el mayor valor fue para Morasurco con 32,97 °C. Los menores valores de Tleaf se dieron el día 15 (DDT), para Morasurco con riego y Ratona Morada sin riego con 22,51 °C y 22,62 °C, respectivamente. Además, se identificó que las plantas bajo estrés homogenizaron el comportamiento de la Tleaf, caso contrario a lo sucedido en plantas con riego, donde el comportamiento varió entre cultivares (Figura 3).
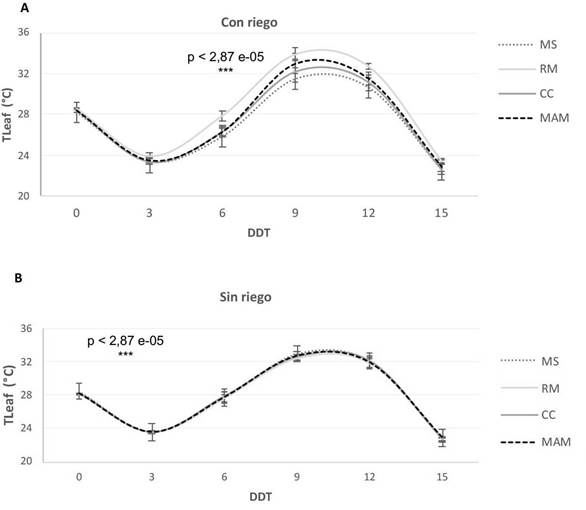
Figura 3 Comportamiento de la temperatura de la hoja (Tleaf) en tratamientos con riego (A) y sin riego (B), en plantas de cuatro cultivares de papa Solanum phureja, en invernadero, en el Centro de Investigación Obonuco, Corporación Colombiana de Investigación Agropecuaria, Pasto, Nariño, Colombia. 2019-2020.
La conductancia estomática (gs) presentó comportamiento diferencial entre las plantas con y sin riego y entre los cultivares en plantas, se evidenció, a modo general, como en plantas con riego el comportamiento varió según el momento de evaluación y, relacionado con las condiciones ambientales, como la temperatura, en plantas sin riego hizo que se presentara un descenso gradual de la gs a medida que aumentaba la severidad del déficit hídrico.
Las gs más altas se presentaron para Criolla Colombia en los días 15 y 6 (DDT) en plantas con riego con 0,416 y 0,414 mol H2O m-2 s-1, respectivamente. Los menores valores se presentaron en plantas sin riego para Morasurco en el día 12 y 9 (DDT) con 0,013 y 0,017 mol H2O m-2 s-1, respectivamente, seguidas de Criolla Colombia con 0,020 mol H2O m-2 s-1 y Mambera con 0,021 mol H2O m-2 s-1, el día 12 (DDT). Fue posible observar que Ratona Morada en plantas sin riego fue el que menos redujo la gs en los días 6 y 9 (DDT) y como en el día 15 (DDT), en el periodo de rehidratación se presentó una recuperación de la gs (Figura 4).
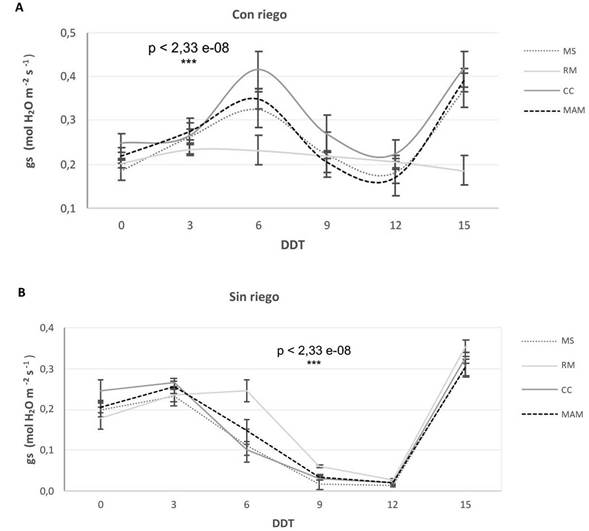
Figura 4 Conductancia estomática (gs) en plantas de cuatro cultivares de papa Solanum phureja, expuestas a tratamientos con riego (A) y sin riego o déficit hídrico (B), bajo invernadero, en el Centro de Investigación Obonuco, Corporación Colombiana de Investigación Agropecuaria, Pasto, Nariño, Colombia. 2019-2020.
En cuanto a la tasa de transpiración (E), el comportamiento fue similar a la gs, donde en plantas con riego la respuesta varió según el cultivar y las condiciones ambientales presentes en el momento de la evaluación. En cuanto a las plantas sin riego, se presentó un descenso gradual de la E a medida que descendió la humedad del suelo.
Los mayores registros de E se presentaron el día 9 y 12 (ddt), para Ratona Morada en plantas con riego, con 5,50 y 5,48 mmol H2O m-2 s-1, respectivamente, seguidos de Criolla Colombia el día 9 (ddt) con 5,31 mmol H2O m-2 s-1. Las menores (E) se presentaron en plantas sin riego el día 12 y 9 (ddt) para Morasurco con 0,29 y 0,57 mmol H2O m-2 s-1, respectivamente, y el día 12 (ddt) para Criolla Colombia con 0,71 mmol H2O m-2 s-1 (Figura 5). Además, se evidencia como Ratona Morada fue el cultivar que sostuvo E más altas bajo condiciones de déficit hídrico.
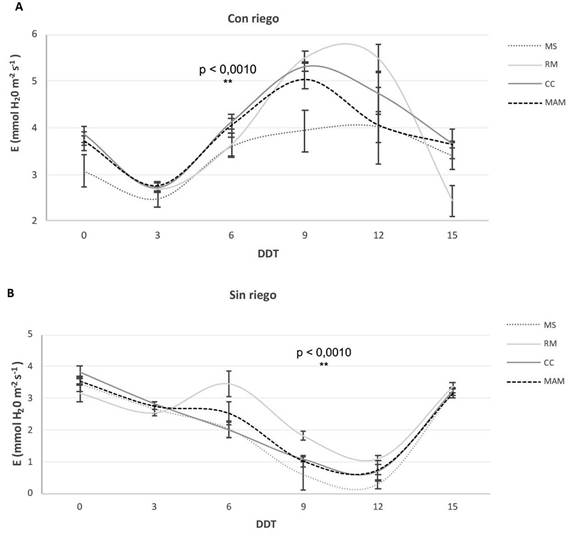
Figura 5 Tasas de transpiración (E) en plantas de cuatro cultivares de papa Solanum phureja, expuestas a tratamientos con riego (A) y sin riego o déficit hídrico (B), bajo invernadero, en el Centro de Investigación Obonuco, Corporación Colombiana de Investigación Agropecuaria, Pasto, Nariño, Colombia. 2019-2020.
La eficiencia fotosintética (Fv/Fm) más alta se presentó al inicio del estudio para Morasurco, tanto en plantas con y sin riego con 0,825 Fv/Fm, seguidos de Ratona Morada en plantas sin riego con 0,823 Fv/Fm. Los menores valores se presentaron el día 9 (DDT) para Morasurco sin riego con 0,746 Fv/Fm, seguidos de Criolla Colombia en plantas con y sin riego con 0,752 y 0,753 Fv/Fm, respectivamente (Figura 6). Además, se evidencia como en el día 12 (DDT) de evaluación en plantas sin riego se evidencian menores valores respecto al control.
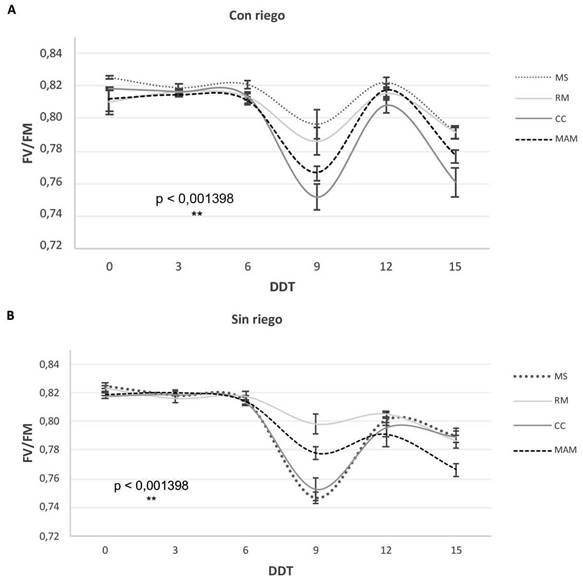
Figura 6 Eficiencia fotosintética Fv/Fm en plantas de cuatro cultivares de papa Solanum phureja expuestas a tratamientos con riego (A) y déficit hídrico (B), bajo invernadero, en el Centro de Investigación Obonuco, Corporación Colombiana de Investigación Agropecuaria, Pasto, Nariño, Colombia. 2019-2020.
En cuanto a las interacciones de dos factores, en la interacción entre el momento de evaluación y el nivel de riego se obtuvieron diferencias estadísticas altamente significativas para variables como A, gs, E, con Pr(>F) < 2e-16, WUEI con Pr(>F) 0,000183, Ci con Pr(>F) 0,000143 y Tleaf con Pr(>F) 8,66e-15. Para la variable A, el mayor valor fue 15,2 µmol CO2 m-2 s-1 a los 0 y 15 DDT para plantas con riego y el menor valor fue para plantas sin riego con 3,7 y 2,3 µmol CO2 m-2 s-1 a los 9 y 12 DDT, respectivamente.
Se evidenció una disminución progresiva para A, gs y E a medida que aumentó el déficit hídrico. Se conformaron tres grupos en el comportamiento respecto a los momentos de evaluación, entre los 0 y 3 DDT, al día 6 DDT y entre los días 9 y 12 DDT, con diferencias estadísticas entre ellos. Además, se evidenció que en las plantas con riego en los días de alta temperatura (32,6 y 31,5 °C), los valores de intercambio gaseoso fluctuaron respecto a los momentos con temperaturas más bajas (Cuadro 3).
Cuadro 3 Comportamiento de las variables de intercambio gaseoso en plantas de papa (Solanum phureja), para la interacción entre el momento de evaluación y el nivel de riego, bajo invernadero, en el Centro de Investigación Obonuco, Corporación Colombiana de Investigación Agropecuaria, Pasto, Nariño, Colombia. 2019-2020.
Ddt | A (µmol CO2/m2/s) | gs (mol H2O/m2/s) | E (mmol H2O/m2/s) | WUEI (µmolCO2/ mol H2O) | Ci (vpm) | Tleaf (°C) | ||
Con riego | 0 | 15,2 ab | 0,21 bc | 3,4 bc | 78,5 b | 205,7 d | 28,4 d | |
3 | 12,5 d | 0,20 bc | 2,6 d | 52,2 bc | 261,1 a | 23,5 g | ||
6 | 14,9 abc | 0,33 a | 3,8 b | 49,8 bc | 240,7 abcd | 26,6 f | ||
9 | 13,6 abcd | 0,23 bc | 4,9 a | 67,2 bc | 217,1 cd | 32,6 a | ||
12 | 12,9 bcd | 0,19 cd | 4,6 a | 72,6 bc | 209,5 cde | 31,5 c | ||
15 | 15,2 a | 0,34 a | 3,3 c | 54,1 bc | 246,4 abc | 22,8 h | ||
Sin riego | 0 | 14,2 abcd | 0,21 bc | 3,5 bc | 73,6 bc | 230,3 abcd | 28,2 d | |
3 | 12,7 cd | 0,25 bc | 2,7 d | 54,7 bc | 255,3 ab | 23,5 g | ||
6 | 9,7 e | 0,15 d | 2,5 d | 75,1 bc | 217,7 bcd | 27,7 e | ||
9 | 3,7 f | 0,035 e | 1,1 e | 106,1 a | 175,1 e | 32,6 a | ||
12 | 2,3 f | 0,02 e | 0,7 e | 107,3 a | 176,4 e | 31,9 b | ||
15 | 14,7 abc | 0,32 a | 3,3 c | 46,7 c | 257,6 a | 22,7 h | ||
Nivel de significancia | *** | *** | *** | *** | *** | *** |
Medias con la misma letra no son significativamente diferentes (p<0,01). Códigos de significancia: 0 ‘***’ 0,001 ‘**’ 0,01 ‘*’ 0,05. / Means with the same letter were not significantly different (p<0.01). Significance codes: 0 ‘***’ 0.001 ‘**’ 0.01 ‘*’ 0.05.
Para el uso eficiente intrínseco del agua (WUEI), los valores más altos fueron en plantas sin riego con 106,1 y 107,3 µmol CO2/mol H2O a los 9 y 12 DDT y los más bajos al día 15 DDT en plantas sin riego en periodo de recuperación con 46,7 µmol CO2/mol H2O. En cuanto al Ci, los valores más altos fueron en el día 15 DDT en recuperación de plantas que estuvieron sin riego con 257,6 vpm y los menores el día 9 y 12 DDT en plantas sin riego con 175 y 176 vpm, respectivamente (Cuadro 3).
Para la interacción entre el cultivar y el nivel de riego las variables que presentaron significancia fueron gs con Pr (>F) 2,42e-08, Tleaf con Pr(>F) < 2e-16, Chl con Pr(>F) 0,01621 y Fv/Fm con Pr(>F) 0,005566. Los valores promedio más altos en la gs se dieron en plantas con riego para Criolla Colombia con 0,31 mol H2O m-2 s-1 y la menor para Morasurco en plantas sin riego (0,14 mol H2O m-2 s-1). Además, se observó que Ratona Morada no presentó diferencias estadísticas entre sus valores promedio en plantas con y sin riego. La Tleaf fue mayor para Ratona Morada en plantas con riego (28,38 °C) y menor para Criolla Colombia en plantas con riego (27,35 °C). Mambera fue el único cultivar que no presentó diferencias significativas entre plantas con y sin riego.
En cuanto a la clorofila (Chl), la mayor concentración fue para Criolla Colombia sin riego con 479,35 mg m-2 y la menor concentración para Mambera con riego con 424,53 mg m-2. Se observó como para Morasurco las concentraciones de Chl no variaron estadísticamente entre plantas con y sin riego y fue superior respecto a los demás cultivares en plantas con riego. En cuanto a la Fv/Fm, el mayor valor fue para Morasurco en plantas con riego con 0,812 Fv/Fm y la menor eficiencia para Criolla Colombia con riego con 0,794 Fv/Fm. Se observó que Morasurco fue el único cultivar que presentó diferencias significativas entre las plantas con y sin riego (Cuadro 4).
Cuadro 4 Comportamiento de variables en plantas de papa (Solanum phureja) por efecto la interacción entre el cultivar y el nivel de riego, bajo invernadero, en el Centro de Investigación Obonuco, Corporación Colombiana de Investigación Agropecuaria, Pasto, Nariño, Colombia. 2019-2020.
Nivel de riego | Cultivar | gs (mol H2O/m2/s) | Tleaf (°C) | Chl (mg m-2) | Fv/Fm | ||
Con riego | CC | 0,31 a | 27,35 d | 450,35 b | 0,794 c | ||
MAM | 0,27 ab | 27,61 cd | 424,53 bc | 0,799 bc | |||
RM | 0,21 c | 28,38 a | 451,94 b | 0,805 abc | |||
MS | 0,26 b | 26,99 e | 477,35 a | 0,812 a | |||
Sin riego | CC | 0,16 d | 27,80 bc | 479,35 a | 0,797 bc | ||
MAM | 0,16 d | 27,75 bc | 459,37 ab | 0,798 bc | |||
RM | 0,18 cd | 27,69 bc | 474,14 a | 0,807ab | |||
MS | 0,14 d | 27,94 b | 470,18 a | 0,799 bc | |||
Nivel de significancia | *** | *** | * | ** |
Medias con la misma letra no son significativamente diferentes (p<0,01). Códigos de significancia: 0 ‘***’ 0,001 ‘**’ 0,01 ‘*’ 0,05. / Means with the same letter were not significantly different (p<0.01). Significance codes: 0 ‘***’ 0.001 ‘**’ 0.01 ‘*’ 0.05.
Para el nivel de riego en el comportamiento de la Chl se obtuvo Pr (>F) 0,0000969, los mayores valores se presentaron para las plantas expuestas a déficit hídrico con 470,76 mg m-2. En cuanto a la Tleaf con Pr (>F) 0,0000166, fue mayor para las plantas sin riego con 27,80 °C. Ci con Pr (>F) 0,015551 mostró los valores más altos en plantas control 230,09 vpm, y para el WUEI con Pr (>F) 0,000104 fue para plantas sin riego con 77,17 µmol CO2/mol H2O. Variables como la A, gs y E con Pr (>F) < 2e-16, los valores más altos siempre fueron para plantas con riego (Cuadro 5).
Cuadro 5 Comportamiento de las variables fisiológicas para el efecto simple del nivel de riego en plantas de papa (Solanum phureja), bajo invernadero, en el Centro de Investigación Obonuco, Corporación Colombiana de Investigación Agropecuaria, Pasto, Nariño, Colombia. 2019-2020.
Variables | Nivel de riego | Nivel de significancia | |
Con riego | Sin riego | ||
A | 14,05 a | 9,42 b | *** |
(µmol CO2/m2/s) | |||
gs | 0,26 a | 0,16 b | *** |
(mol H2O/m2/s) | |||
E | 3,81 a | 2,29 b | *** |
(mmol H2O/m2/s1) | |||
WUEI | 62,41 b | 77,17 a | *** |
µmol CO2/mol H2O | |||
Ci | 230,09 a | 218,72 b | * |
(vpm) | |||
Tleaf | 27,58 b | 27,80 a | *** |
(°C) | |||
Chl | 451,04 b | 470,76 a | *** |
(mg m-2) |
Medias con la misma letra no son significativamente diferentes (p<0,01). Códigos de significancia: 0 ‘***’ 0,001 ‘**’ 0,01 ‘*’ 0,05. / Means with the same letter were not significantly different (p<0.01). Significance codes: 0 ‘***’ 0.001 ‘**’ 0.01 ‘*’ 0.05.
Para el efecto simple del cultivar, se evidenció como la A (Pr(>F) 0,08704) no presentó diferencias entre los cultivares con un promedio de 11,74 µmol CO2 m-2 s-1. En cuanto a la gs (Pr(>F) 0,000192), los mayores valores fueron para Criolla Colombia con 0,23 mol H2O m-2 s-1. Las variables E (Pr(>F) 1,27e-07), Tleaf (Pr(>F) 3,28e-15) y Fv/Fm (Pr(>F) 2,88e-05), mostraron los valores más altos en Ratona Morada, con 3,24 mmol H2O m-2 s-1, 28,03 °C y 0,806 Fv/Fm. Por último, la concentración de Chl (Pr(>F) 9,68e-05) más alta fue para Morasurco con 473,76 mg m-2 (Cuadro 6).
Cuadro 6 Comportamiento de las variables fisiológicas para el efecto simple del cultivar en plantas de papa (Solanum phureja), bajo invernadero, en el Centro de Investigación Obonuco, Corporación Colombiana de Investigación Agropecuaria, Pasto, Nariño, Colombia. 2019-2020.
Cultivar | A (µmol CO2/m2/s) | gs (mol H2O/m2/s) | E (mmol H2O/m2/s) | Tleaf (°C) | Chl (mg m-2) | Fvfm |
CC | 12,14 a | 0,23 a | 3,17 a | 27,57 bc | 464,85 a | 0,796 b |
MAM | 11,92 a | 0,21 ab | 3,08 a | 27,68 b | 441,95 b | 0,798 b |
MS | 11,18 a | 0,20 b | 2,72 b | 27,46 c | 473,76 a | 0,805 a |
RM | 11,70 a | 0,19 b | 3,24 a | 28,03a | 463,04 a | 0,806 a |
Promedio | 11,74 | 0,21 | 3,05 | 27,69 | 460,9 | 0,8 |
Nivel de significancia | *** | *** | *** | *** | *** |
Medias con la misma letra no son significativamente diferentes (p<0,01). Códigos de significancia: 0 ‘***’ 0,001 ‘**’ 0,01 ‘*’ 0,05. / Means with the same letter were not significantly different (p<0.01). Significance codes: 0 ‘***’ 0.001 ‘**’ 0.01 ‘*’ 0.05.
Discusión
El estrés por déficit hídrico afectó el comportamiento de los diferentes grupos de variables evaluados, y activaron una cadena de efectos y respuestas graduales por parte de las plantas, que dependieron, de la intensidad y duración del estrés, el genotipo y covariables como la temperatura. El cierre estomático (gs) jugo un papel principal como estrategia para evitar la pérdida de agua en el presente estudio, como lo reportado para cultivos de trigo, algodón y caña azucarera (Da Graça et al., 2010; Pallas et al., 1967; Siddique et al., 2000). Además, los valores obtenidos de gs concuerdan con estudios similares, donde los valores más bajos fueron por debajo de 0,074 mol m-2 s-1 (Ariza Acevedo, 2017; Rodríguez Pérez, 2015).
El cierre estomático causó la disminución gradual de la A y E, además del incremento en la temperatura foliar y el WUEI. Varios autores han reportado como la gs y la conductancia del mesófilo son unas de las principales limitaciones de la fotosíntesis bajo estrés hídrico (Ierna & Mauromicale, 2006; Liu et al., 2006; Tourneux et al. 2003). El aumento en la WUEI se debió a la efectividad de los estomas en maximizar la fotosíntesis y reducir la pérdida de agua, por lo cual se configura como una estrategia de adaptación. También se evidencia la acción refrescante de la E para la hoja, la cual al disminuir, aumentó la temperatura foliar, lo que puede traer efectos secundarios al déficit hídrico (Ahmadi et al., 2010).
La diminución del Ci fue más lenta a la gs, solo se evidenció cuando el estrés fue severo. La concentración de Ci es reguladora de la apertura de los estomas, a bajas concentraciones los estomas se abren independiente de los demás factores ambientales, a excepción de situaciones de estrés hídrico severo, comportamiento evidenciado en este estudio. Al inicio del estrés el cierre estomático se correlacionó con la reducción de la tasa de fotosíntesis, pero no fue la única causa de esta disminución, porque la concentración del Ci en las hojas no se vio afectada, lo que evidencia la presencia de otros factores inhibidores de la fotosíntesis diferentes a los estomáticos (Schapendonk et al., 1989).
Se pudo observar que según el nivel de estrés la respuesta de la planta fue gradual, y se activaron mecanismos e interacciones entre los diferentes grupos de variables, lo que permitió identificar diferentes momentos de respuesta durante el periodo de estudio: cuando no se presentó estrés (capacidad de campo-20 % θ), estrés moderado (20 -15 % θ) y estrés severo (15 - 10 % θ). Valores similares fueron reportados para A (Díaz Valencia 2016). Los parámetros de intercambio gaseoso son sensibles a cambios presentados durante el día y se consideran variables de respuesta rápida, y son de utilidad en la identificación rápida del estrés y para la planificación de momentos de riego (Ramírez et al., 2014; 2015; Rolando et al., 2015).
La definición de programas de riego a partir de mediciones de contenido de agua del suelo son los más utilizados. Sin embargo, un grupo de autores han sugerido el uso de variables fisiológicas sobre el estado hídrico de la planta para una definición más exacta del momento óptimo de aplicación de riego (Medici et al., 2014). En estudios similares postularon que este rasgo es el indicador más apropiado de la intensidad del estrés hídrico. Así, se han propuesto valores de gs entre 0,1 y 0,15 mol H2O m-2 s-1, como umbral óptimo para regar el cultivo de papa (Flexas et al., 2004).
Fue posible evidenciar la eficiente recuperación de las plantas al rehidratarse, diferentes autores han reportado esta capacidad que presenta este cultivo (Ramírez et al., 2016). Otros autores plantean que gs por debajo de 0,05 mol H2O m-2 s-1 en papa, representa un umbral fisiológico que de superarse durante etapas sensibles, no se producirá una recuperación total, lo que conllevará a una reducción del rendimiento (Flexas et al., 2004; 2008). En el presente estudio, en estrés severo, las gs fueron menores a las asociadas como umbral, sin presentar daños profundos en el funcionamiento fotosintético al rehidratarse, pero sin evaluar las repercusiones en rendimiento de tubérculo, lo cual es una asignatura pendiente para definir umbrales fisiológicos en este tipo de cultivares.
En cuanto al efecto de los cultivares en la evaluación, de manera general, se encontró diferencias en sus respuestas fisiológicas; Criolla Colombia tendió a ser superior en sus parámetros fisiológicos respecto a los demás cultivares sin estrés, pero bajo condiciones de estrés el comportamiento entre los cultivares fue similar, incluso Ratona Morada tardó más en el cierre de estomas. El cierre estomático rápido, puede estar ligado a los cultivares tolerantes; sin embargo, se deben evaluar de forma integral estas variables para definir la tolerancia, puesto que un cultivar que tiene la capacidad de mantener las gs o tasa de fotosíntesis más altas bajo déficit hídrico, también puede ser tolerante (Obidiegwu et al., 2015).
La concentración de clorofila también evidenció diferentes respuestas ligadas al genotipo, por ejemplo, la reducción en la concentración en Morasurco puede estar relacionada con la producción del oxígeno sínglete y el incremento de enzimas clorofilasa bajo estrés (Eckardt, 2009). En la variedad Criolla Colombia el aumento de la concentración puede ser interpretado como un mecanismo de protección del sistema fotosintético; la disminución de la turgencia celular y, por consiguiente, la reducción del área foliar pueden explicar el aumento en la concentración de clorofila bajo estrés por déficit hídrico (Fanizza et al., 1991). De igual manera, se ha reportado un aumento del verdor por déficit hídrico en papa y en Bouteloua gracilis (García-Valenzuela et al., 2005; Ramírez et al., 2014; Yactayo et al., 2013).
En las primeras fases de estrés la concentración de clorofila y Fv/Fm no fueron afectadas, pero al aumentar la intensidad del estrés se presentó una variación en los valores de estas variables, al igual como se mencionó con la concentración de Ci. En otro estudio se concluyó que un 62 % de agua aprovechable en el perfil del suelo, causó disminución de la gs, por el contrario, no se afectó la actividad del PSII, ni el equilibrio entre el quenching no-fotoquímico (NPQ) y el fotoquímico (qP) (Jefferies, 1994). Estas variables son de respuesta intermedia a lenta y pueden ser útiles para la selección de genotipos e implementación de programas de mejora genética para la adaptación al cambio climático.
La mayoría de los experimentos no distinguen entre estrés hídrico y estrés térmico. Estos factores están correlacionados de forma positiva. En el presente estudio se observó una reducción en las gs, A y E, a excepción de Ratona Morada, la cual presentó un aumento en la tasa de transpiración bajo condiciones de alta temperatura en las plantas que no se sometieron a estrés; resultados similares observaron distintos autores (Morales et al., 2006; Paul et al., 2017). De igual manera, la Fv/Fm presentó una caída en sus valores por alta temperatura en plantas control; otros estudios obtuvieron comportamientos similares en (Fv/Fm), con valores de 0,53 al exponerlas solo a déficit hídrico y con 0,49 a déficit hídrico y altas temperaturas (Havaux,1993).
Conclusiones
La disminución de los valores de intercambio gaseoso se dio de manera progresiva con la reducción gradual de la humedad del suelo, dándose tres niveles de respuesta, estrés leve, moderado y severo. El cierre estomático como una respuesta de aclimatación resultó útil para detectar estrés temprano en las plantas. Esto podría, luego en otros estudios, definir calendarios de riego y/o épocas de siembra según el ambiente.
El comportamiento de variables como Ci, clorofila y fv/fm, variaron después de la disminución del intercambio gaseoso, lo que da indicios de una cadena de respuestas a nivel fisiológico que aumentan su complejidad según crece la intensidad y duración del estrés, lo cual puede ser objeto de estudio para propuestas de futuras investigaciones.
La respuesta de la papa varió entre sus cultivares, por lo cual resulta de gran importancia profundizar en estudios donde se evalué de manera conjunta las variables mencionadas en este estudio, con variables referentes al crecimiento y rendimiento para determinar la tolerancia y susceptibilidad.