Introduction
Fusarium fungi are cosmopolitan plant pathogens which lead to important yield and economical loss in many crops. Among this genus, many species have saprophytic life cycles and do not represent any risk, but some are plant pathogens and even can act as human and animal opportunistic pathogens (Harmann et al. 2004). Fusarium wilt on tomatoes (Solanum lycopersicum L.) is caused by Fusarium oxysporum f.s. lycopersicy Schltdl. which leads to a severe wilt of the vascular system and a general plant chlorosis, necrosis, premature leaf drop, etc., as consequences. Exudates from plant roots trigger germination of the fungal resting structures which are in the soil, penetrating the host plant’s root tips, root wounds, or lateral roots. The fungi then colonize cortical cells and migrate intercellularly toward the vascular parenchyma and invade the xylem vessels (Yadeta & Thomma 2013).
In the last decades, concerns about the overuse of chemical pesticides has increased and countless attempts to incorporate biological control agents in the fields have been done. Trichoderma spp. Persoon are well known biological control agents to plant pathogenic fungi (Harman 2006), specially soil-borne diseases. The beneficial effects of Trichoderma have been attributed to many factors such as antagonism, mycoparasitism (Kubicek et al. 2001), production of secondary metabolites (Reino et al. 2008), promotion of plant growth by improvement on water and nutrient uptake (Chet 2001, Harman 2006, Li et al. 2018), promotion of plant resistance (Jogaiah et al. 2018) and effects mediated by environmental factors (Zhang et al. 1998), Trichoderma species and the host plant genotypes (Tucci et al. 2011). These mechanisms have been favoured by the ability of Trichoderma for colonizing plant roots (Howell 2006) which can even reduce foliar diseases (Harman et al. 2004). Some Trichoderma species are capable to improve soil conditions for root development, reducing plant stress (Shoresh et al. 2005) and by activation of physiological and biochemical mechanisms to induce resistance to pathogens by plants (Hanson & Howell 2004, Harman et al. 2004, Hoitink et al. 2006).
In Venezuela, more than 11.900 ha are dedicated to tomato production and up to date, nearly 1000 ha of greenhouses have been installed to grow tomato, sweet pepper, lettuces and flowers mainly. As many places in the world, Venezuelan crops are highly affected by Fusarium species, but in many cases the management of the disease is inefficient and losses are important. Some authors have calculated the severity of F. oxysporum in Venezuelan tomato plantations and have determined more than 60% of yield losses (Anzola & Román 1982) in many places across the country. Currently the vast majority of control measures worldwide to tackle Fusarium are related to plant resistant varieties and chemical fungicides applications; however, the pathogen remains an enormous problem. The excessive and indiscriminate use of chemical fungicides also have increased detrimental effects on the environment, water sources, food, and people (especially farm workers, and final consumers).
The use of Trichoderma strains in Venezuelan fields has increased since 1990 but many aspects regarding their mode of action, plant protection effect or yield increase remain unknown (Perdomo et al. 2007, Guédez et al. 2009, López et al. 2010). At the beginning, some alien species were introduced to the country but with less than good results (probably, due to ecological differences between the place of origin of the strain and the tropical environment of Venezuela). Isolation and production of native species has increased during the years and now around 20 commercial Trichoderma-based products are being sold in the country while research goes on. The objectives of this work were i) to test the effect of 4 Trichoderma species isolated in the country on F. oxysporum in in vitro conditions ii) to evaluate the potential of these Trichoderma spp. as biological control agents in controlled conditions and iii) to evaluate their performance in commercial greenhouses for the production of tomatoes.
Materials and methods
Biological material
F. oxysporum was isolated directly from tomato diseased plants found in different horticultural farms at El Jarillo, Estado Miranda, Venezuela. Pieces of stem of the plants were surface sterilized using sodium hypochlorite at 3% for 10 minutes. After sterilization, the stem pieces were cut longitudinally and damaged tissues were separated and plated in Potato Dextrose Agar (PDA). Plates were incubated for 5 days at 28°C and growing mycelia were re-plated and purified following the same protocols. Identification was done by both light microcopy observation of conidia and molecular tools (Rep et al. 2004) (done in the Molecular Phytopathology laboratory at Instituto de Estudios Avanzados.
Trichoderma spp. were obtained from the collection of the Molecular Phytopathology laboratory that houses isolates from different agricultural soils across the country. Four species were used in the experiments (T. koningiopsis Samuels, Suárez & Evans (VT16), T. virens Mill., Giddens & Foster (VT43), T. spirale Bissett (VT56) and T. harzianum Rifai (CT6)).
Antagonism tests
Dual culture assay
Twenty five PDA plates were prepared and inoculated with F. oxysporum or individually with each Thrichoderma species and incubated as explained earlier. After 5 days the plates were used as inoculum for antagonism experiments.
A plug of growing mycelium (5 mm) of F. oxysporum was placed in a side of a PDA plate and incubated for 48 h at 28°C. Then a plug of a single Trichoderma species was placed in the opposite side of the petri dish and returned to the incubator (control plates were inoculated only with F. oxysporum). The daily growing rate of F. oxysporum was measured every 24 h, measuring the diameter of the fungus in 2 perpendicular directions and the experiment was finished when the control PDA plate (only F. oxysporum) was totally covered by the fungus.
The perpendicular measurements of the F. oxysporum mycelium were averaged. The antifungal index was determined in comparison with the control plates, and calculated by the Wang et al. (2009) equation seen below. Where Da is the diameter of the growing mycelium of the F. oxysporum in the antagonism plates, and Db is the diameter of the growing mycelium of the F. oxysporum in the control plate (Wang et al. 2009).
Antifungal index (%) = [1-(Da-0.5)/(Db-0.5)] x 100 The experiment had 5 replicas and was repeated three times.
Micro-culture of fungi for microscopic observations
The microscopic effects of the 4 Trichoderma species on F. oxysporum mycelium, and conidia were evaluated using the protocol developed by Riddell (1950) with few modifications. A PDA square (10 mm diameter x 20 mm height) was placed upon a sterile glass slide. Using a microbiological loop, tiny pieces of F. oxysporum mycelium were placed in the opposite lateral sides of the agar block (i.e. north and south sides) and placed in a moist chamber for 48 h at 28°C in an incubator. The chamber consisted on a Petri dish half filled with 10 ml of 10% glycerol solution and some glass rods used as support to avoid the slide glass to sink. After F. oxysporum inoculation, tiny pieces of Trichoderma were attached to the other free sides of the agar block (i.e. east and west sides). A sterile cover glass was then set on upon the top of the block and the slide was returned to the moist chamber and incubated for 96 h at 28°C. After incubation, the glycerol solution in the Petri dish was substituted by a solution of 10% formaldehyde for 2 h to fix fungal structures. The cover glass was withdrawn out from the PDA block and set in a new microscope glass slide with a drop of cotton blue/lactophenol. The new slides were sealed an evaluated using a Leica® DM2500 compound microscope fitted with a differential interference contrast system. The experiment was replicated 5 times and repeated 3 times.
Trichoderma antibiosis
To study the effect of secondary metabolites, experiments were done following Vinale et al. (2009). Twenty five 50 ml Erlenmeyer flasks were filled with Potato Dextrose Broth (PDB) and sterilized by autoclaving. Five plugs of 5 mm of growing mycelium of every Trichoderma species were transferred aseptically into the flasks (1 plug per flask) and 5 flasks remained as control broths. The flasks were place in a shaker (100 rpm) for 9 days at room temperature (25±2°C). After incubation, the flask content was pre-filtered to separate the mycelium from the broth using filter paper Whatman No.6 (3 μm pore). The resulting broth was vacuum filtered using Millipore® membranes (0.22 μm) to extract only metabolites. An aliquot of the sterilized metabolite suspension was plated in PDA to confirm the fungus absence.
A 5 mm plug of plated F. oxysporum was soaked in the Trichoderma metabolite suspensions for 15 min (a control plug was soaked in the control broth) and plated in PDA. The mycelium growth was recorded by measuring the diameter of the fungus in 2 perpendicular directions and the experiment was finished when the control PDA plate (only F. oxysporum) was totally covered by the fungus. The experiment was repeated 3 times and replicated 5 times. The antifungal index was calculated as before.
Effect of different Trichoderma species on tomato plants infected or non-infected with F. oxysporum in laboratory conditions
Inoculum preparation
F. oxysporum PDA plates were prepared as before and incubated for 7 days. Sterile water was applied after incubation into the plates and the mycelium was scraped with a sterile glass rod. The suspension of spores was filtered and poured into tubes and the concentration of spores were adjusted using a hemocytometer to 1,0 x 106 spores.ml-1. Trichoderma species inoculum was set in the same way than F. oxysporum but 3 different concentrations of spores were prepared (1,0 x 106, 1,0 x 107 and 2,0 x 106 spores.ml-1).
Tomato seed treatments
Thirty grams of tomato seeds (undetermined hybrid Camelia, Hazera®, resistant to F. oxysporum f. sp. lagenariae (Race1)) were surface sterilized by soaking them in a 20% solution of sodium hypochlorite for 10 min. Seeds were washed 3 times with sterilized distilled water for 5 min. Sterile seeds were separated into 12 groups (2 g each) and inoculated accordingly with the 4 Trichoderma species using 3 different spore concentrations (1,0 x 106, 1,0 x 107 and 2,0 x 106 spores.ml-1). Seeds were soaked in the Trichoderma spore inoculum (5 ml water and 1 ml of spore suspensions) for 15 min and placed in a filter paper to remove water excess. Two groups of sterile seeds were kept sterile to serve as control (one group as negative control using sterile water as inoculum and the remaining as positive control inoculated with F. oxysporum as before).
After 30 days post-emergence, plantlets were transplanted in 1 l pots (10 plants per treatment) containing a sterile mixture of peat moss and sand (3:1 v/v) and placed randomly in a table under controlled temperature (25±2°C) and 12 h photoperiod. Irrigation was done manually with sterile water. After 7 days post-transplant, the plantlets were inoculated with F. oxysporum by applying the spores (1,0 x 106 spores in 5 ml of water) directly on the substrate (except negative controls), the rest of Trichoderma inoculated plants were used as positive control (without F. oxysporum) and to measure the development of plants inoculated only with Trichoderma. After 60 days post emergence, 5 plants per treatment were chosen for further measurements: stem height, stem and roots fresh weight, stem and roots dry weight, severity of F. oxysporum infection. The experiment was replicated 3 times.
Greenhouse study
The study was carried in “Finca Frutiagro PTM” at El Jarillo, Estado Miranda, Venezuela (N 10°20’42”; W 67°9’35”) in commercial greenhouses in which cherry tomatoes were grown (undetermined hybrid Camelia, Hazera®) in which a plant death outbreak of 34.7% was attributed to F. oxysporum (such plants were the F. oxysporum source for this work).
Three weeks before transplanting, seed were set in trays with sterilized peat moss to ensure disease -free plantlets. The greenhouse soil was prepared, beds were rose, and a fungicide based on 77% copper hydroxide was applied, following manufacture instructions by drench (1 kg per ha). Beds then were covered with plastic and drip irrigation was installed. Soil physic chemistry is as follows: (pH = 6.2; electric conductivity = 0.2; %clay = 17; %silt = 27; %sand = 56; %RH inside the greenhouse = 75%; photoperiod = 12:12, mean temperature inside the greenhouse = 33°C).
Free disease tomato plantlets, using sterile substrate and distilled water were produced in a local nursery. Before transplanting, the germination trays with the plantlets were soaked in a suspension containing the given Trichoderma species (5 liters of distilled water with 5 ml of a suspension of 5,0 x 108 spores.ml-1) for 15 min. Control trays were soaked in distilled water. From the trays, 40 plantlets per Trichoderma species and 40 control plantlets were chosen randomly and transplanted in 4 blocks distributed randomly inside the greenhouse in lines of 10 plants (1 line per treatment). The plantlets were transplanted directly in the greenhouse soil (with no treatment) to allow the natural infections of F. oxysporum. The Trichoderma inoculations were repeated once a week (100 ml of water with 1 ml of the spore suspension of 5,0 x 108 spores.ml-1) after transplanting for 4 week using a manual spray pump. At day 90 after transplanting, the first harvest of tomatoes was done; randomly 3 plants per line per treatment were chosen and their height, number of fruits of the harvested first cluster and fruit weight were measured. If any plant showed symptoms of F. oxysporum wilt, samples of stem tissues were taken to microscopically confirm F. oxysporum presence in PDA plates.
Statistical analysis
Data collected were evaluated using Minitab® statistical program. Prior analysis, normality tests were performed. Experimental differences between treatments when normality was achieved were determined by ANOVA. If significant differences were found, Tukey family comparisons were done. If collected data were in percentage, the results were angular transformed and presented untransformed (%±s.d.).
Results
Antagonism tests
Dual culture assay
The antifungal index of Trichoderma spp. to F. oxysporum was different according to the species (p≤0.001; α=0.05) (Table 1). Along the experiment until 168 h, T. koningiopsis showed the highest antifungal indexes, but there was a delay in its effect in the last measurement. Trichoderma spirale was the most effective inhibitory fungus in plates at the end of the experiment (192 h) by 78.3±4.9%, followed by T. koningiopsis (76.6±5.5%). Trichoderma virens and T. harzianum showed less inhibitory capacity than the previous ones (73.3%).
Table 1 Atifungal effect caused by different Trichoderma species against Fusarium growth at different time in Petri dishes.
Percentage of radial growth inhibition (%) | ||||||||
Treatment | 24 h | 48 h | 72 h | 96h | 120 h | 144 h | 168 h | 192 h |
D94 | 0±0 d | 0±0 d | 0±0 d | 0± 0 d | 0±0 d | 0± 0 d | 0±0 d | 0±0 d |
D94+VT56 | 0±0 d | 0±0 d | 23.2±3.9 c | 34.4±5.2 c | 51.9±4.9 c | 62.1±4.9 c | 68.5±4.9 c | 78.3±4.9 c |
D94+VT43 | 0±0 d | 0±0 d | 16.9±3.8 b | 24.2±6.2 b | 42.2±5.0 b | 54.4±5.0 b | 62.0±5.0 c | 73.3±5.0 b |
D94+VT16 | 0±0 d | 0±0 d | 21.1±3.8 a | 27.5±6.4 a | 48.8±5.6 a | 54.6±5.7 a | 65.2±5.7 a | 76.6±5.5 c |
D94+CT6 | 0±0 d | 0±0 d | 17.7±4.3 b | 24.2±7.0 b | 42.4±5.5 b | 54.6±5.5 b | 62.2±5.5 b | 73.3±5.5 b |
Different letters indicate significant differences within the same time of evaluation according to Tukey test (p<0.05).
Micro-culture of fungi for microscopic observations
Interactions between Trichoderma and F. oxysporum were different according with the studied species. All Trichoderma species showed mycoparasitism against F. oxysporum. First, the Trichoderma hyphae entered in contact with the pathogen hyphae and, after recognition, the antagonist hyphae adhered and penetrated the F. oxysporum hyphae destroying them. On the other hand, interactions between spores were observed. Trichoderma conidia surrounded the F. oxysporum ones causing deformation and posterior lysis.
Trichoderma antibiosis
When secondary metabolites were tested, the antifungal index to F. oxysporum also differed according to the Trichoderma species (p≤0.001; α=0.05) (Table 2). Metabolites coming from T. spirale were more effective in fungal inhibitory capacity (64.28±0.8%) followed by T. virens (61.42±0.6%). Metabolites from T. harzianum and T. koningiopsis were less effective against F. oxysporum in in vitro conditions, with percentages of growth inhibition of 60±1.3% and 57.2±0.5%, respectively.
Table 2 Atifungal effect caused by metabolites produced by different Trichoderma species against Fusarium growth at different time in Petri dishes.
Percentage of radial growth inhibition (%) | ||||||||
Treatment | 24 h | 48 h | 72 h | 96h | 120 h | 144 h | 168 h | 192 h |
D94 | 0±0 e | 0±0 e | 0±0 e | 0±0 e | 0±0 e | 0±0 e | 0±0 e | 0±0 e |
D94+VT56 | 5±0.7e | 16.6±0.6d | 15.5±1.2 d | 18.6±1.0 d | 33.8±0.7 d | 48.4±0.7 d | 56.2±0.7 d | 64.2±1.2 d |
D94+VT43 | 5±0.7 e | 16.6±0.6 d | 18±0.8 c | 18.6±1.0 d | 33.8±0.7 d | 44.8±1.0 c | 51.1±1.1 c | 61.4±0.3 c |
D94+VT16 | 8.8±0.8 c | 16.6±0 d | 12.5±1.5 b | 16.6±0.6 c | 32.3±0.5 c | 43.2±1.0 b | 49.1±0 b | 57.2±0.5 b |
D94+CT6 | 0±0 e | 13±1.3 c | 7±0.8 a | 18.6±1.0 d | 33.8±0.7 d | 44.8±1.0 c | 51.3±1.2 c | 60±1.3 c |
D94 = Fusarium oxysporum (control); VT56 = Trichoderma spirale; VT16 = Trichoderma koningiopsis; VT43 = Trichoderma virens and CT6 = Trichoderma harzianum.
D94 = Fusarium oxysporum (control); VT56 = Trichoderma spirale; VT16 = Trichoderma koningiopsis; VT43 = Trichoderma virens and CT6 = Trichoderma harzianum.
Different letters indicate significant differences within the same time of evaluation according to Tukey test (p<0.05)
Effect of different Trichoderma species on tomato plants infected or non-infected with F. oxysporum in laboratory conditions
Plants infected with F. oxysporum
The disease presented 30% of incidence when tomato plants were treated with 1,0 x 106 spores of T. koningiopsis whereas the rest of the Trichoderma species treatments showed an incidence of 20%. When the dose was increased to 1,0 x 107and 2,0 x 106 spores of Trichoderma per ml, the incidence lowered to 10% in all treatments. Plants treated only with Trichoderma or only with F. oxysporum produced 0% and 70% of incidence of the disease, respectively, although symptoms of the disease were not severe.
Measured variables such as plant height were significantly different when compared with positive control (plants infected with F. oxysporum) (p≤0.001; α=0.05). Plants inoculated with Trichoderma species and F. oxysporum kept the same
height than the control plants, around 14.7±1.4 cm (Figure 1A). However, significant differences were noticed depending on the Trichoderma species under test, when fresh and dry weight of the roots and stems of the infected plants were measured.
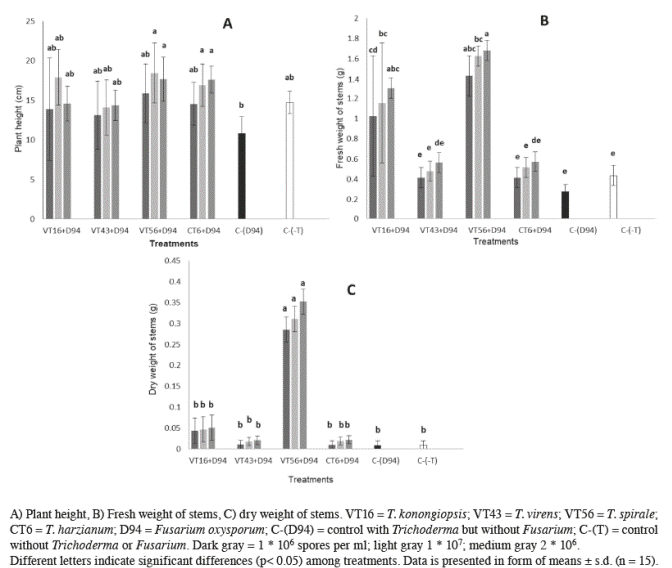
Figure 1 Effect of different doses of 4 Trichoderma species on tomato plants inoculated with Fusarium oxysporum in aerial measurements after 60 days post-emergence.
Fresh weight of stems (Figure 1B) revealed that plants inoculated with T. virens and T. koningiopsis weighed 66% and 57% more than all other treatments, including T. spirale, T. harzianum and both controls (p≤0.001; α=0.05). Spore numbers were the same when fresh weight of the stems was measured. Dry weight of stems also showed significant differences among treatments (p≤0.001; α=0.05). Dry stems coming from plants treated with T. spirale were heavier (0.5±0.03 g) than those produced by all other treatments, including controls (in which none of them was heavier than 0.05±0.01 g) (Figure 1C).
Fresh weight of plant roots showed significant differences between treatments (p≤0.001; α=0.05). Trichoderma spirale, and control plants (without F. oxysporum or Trichoderma sp.) were heavier (0.5±0.06 and 0.43±0.03 respectively) (Figure 2A) compared to all other treatments. When the roots’ dry weight was calculated, plants infected with F. oxysporum and inoculated with T. spirale showed differences comparing with other treatments (p≤0.001; α=0.05) (Figure 2B).
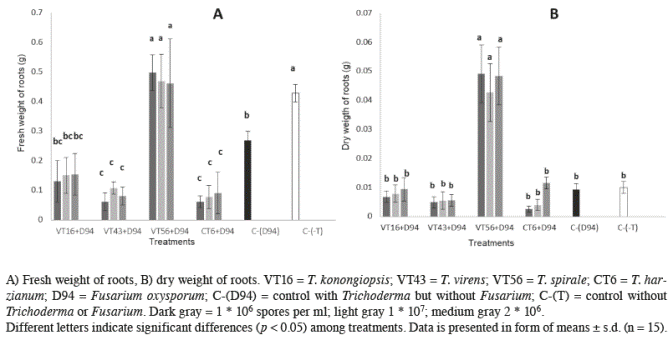
Figure 2 Effect of different doses of 4 Trichoderma species on tomato plants inoculated with Fusarium oxysporum in root measurements after 60 days post-emergence.
Effect of Trichoderma species on the development of tomato plants
Plants inoculated only with Trichoderma spp. showed differences in plant height (p>0.001; α=0.05) (Figure 3A). Plants inoculated with 1,0 x 107 and 2,0 x 106 spores of T. spirale and 2,0 x 106 spores of T. koningiopsis showed the highest growing rate among treatments as 22.8±2.4, 21.7±0.9 and 21.9±2.0 cm, respectively. The shortest height went to control plants infected with F. oxysporum (10.83±1.4 cm). Control plants (with neither F. oxysporum nor Trichoderma) were also reduced when compared to every Trichoderma treatment (14.7±2.1 cm).
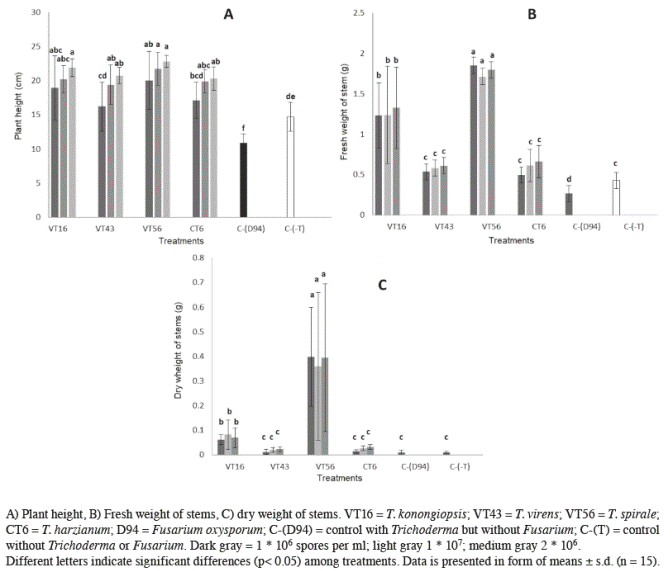
Figure 3 Effect of different doses of 4 Trichoderma species tomato plants (Fusarium free) aerial measurements after 60 days post-emergence.
Fresh stem weight also varied significantly among treatments (p>0.001; α=0.05) (Figure 3B); 4 different groups were clearly identified. The maximum weight was recorded when plants were inoculated with 1,0 x 107 and 2,0 x 106 spores of T. spirale (1.85±0.1 g and 1.8±0.1 g respectively). Plants treated with T. koningiopsis formed a second group with a maximum fresh weight of 1.32±0.5 g. The third group was formed by T. harzianum, T. virens and control plants (without F. oxysporum infections) where maximum weight were 0.66±0.2, 0.61±0.1 and 0.43±0.1 g respectively. Control plants inoculated with F. oxysporum had lighter fresh stems (0.42±0.1 g).
As before, dry weight of stems were significantly different among treatments (p<0.001; α=0.05) (Figure 3C). However in this case, only plants treated with T. spirale and T. koningiopsis showed such differences. Plants treated with T. spirale registered the heaviest stems with a maximum of 0.40±0.2 g whereas T. koningiopsis formed a second group with a maximum weight of 0.08±0.2 g, all other treatments including both controls weighted equally, forming a third group.
Fresh and dry weight of roots also showed significant differences among treatments (p>0.001; α=0.05 and p>0.001; α=0.05 respectively), plants treated with T. spirale performed the best (Figure 4A and 4B). Patterns were similar compared to the fresh and dry weight of stems, plants treated with T. spirale had heavier stems than all other treatments (p<0.001; α=0.05 and p<0.001; α=0.05 respectively).
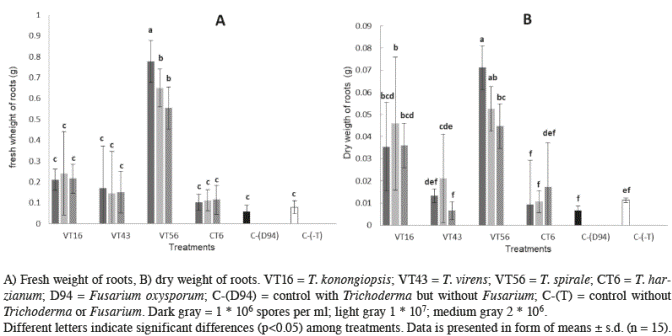
Figure 4 Effect of different doses of 4 Trichoderma species on tomato plants (Fusarium free) in root measurements after 60 days post-emergence.
Field study higher (120.4±25.3 cm) followed by plants treated with T. virens (91.4±31 cm), all other
Plant height at 90 days varied accor- plants were similar but smaller (74.4±12.82 ding to treatment (p<0.001; α=0.05). Plants cm) (Figure 5A).
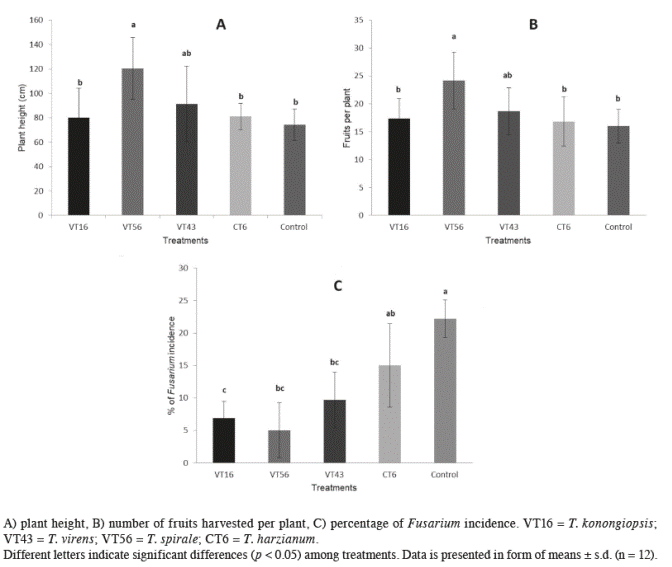
Figure 5 Effect of 4 Trichoderma species on tomato plants in a commercial farm greenhouse after 90 days post-transplant.
The number of harvesting fruits followed the same pattern that the plant height according to the applied treatment (Figure 5B). The number of fruits harvested in the period of the experimental trial (90 days after transplanting) was significantly higher (p=0.04; α=0.05) on those plants treated with T. spirale (24±7 fruits), followed by plants treated with T. virens (19±4 cm), remaining treatments produced similar number of fruits as controls (16±3 cm). Individual weight per fruit was not statistically different between treatments or the control (p=0.58; α=0.05), mean weight value being 18.8±3.9 g per fruit.
Plants affected by F. oxysporum wilt differed according to the Trichoderma species (p>0.001; α=0.05). Control plants and those inoculated with T. harzianum were affected by F. oxysporum in 22.3±2.9% and 15±6.4%, respectively. Plants inoculated with T. virens and T. koningiopsis showed medium rates of infection (9.7±4.3% and 6.9±2.6%, respectively), whereas plants treated with T. spirale were less affected by the pathogen (5±4.22%) (Figure 5C).
Discussion
According to the results, in vitro antagonism tests showed an evident effect of Trichoderma against F. oxysporum fungus. This antagonistic activity has been reported in a number of studies in which authors have proposed several mechanisms to explain the biocontrol effect of Trichoderma (Lorito et al. 1993, Latunde-Dada 1993; De Boer et al. 2003, Howell 2003, Asran-Amal et al. 2005, Hoyos-Carvajal et al. 2008, Sempere & Santamarina 2008). Kullnig et al. (2000) mentioned mycoparasitism, antibiosis and competence for both space and nutrients as the most important mechanisms of Trichoderma species for reducing or inhibiting the growth of phytopathogenic fungi. The inhibition percentages in the present work between Trichoderma species tested in in vitro experiments and the pathogen indicates biocontrol potential in all Trichoderma species, however, T. spirale performed better inhibitory capacity against F. oxysporum.
All 4 species tested in this work, presented different levels of antagonism against F. oxysporum, and mycoparasitism was observed in all species. The mycoparasitic process includes localization through chemotrophic growth, recognition, adhesion to target hyphae, coiling and lysis (Chet & Inbar 1994, Bruce et al. 1995). Trichoderma species were able to deform and produce septation in F. oxysporum conidia, as well as to lysate and destroy conidiophores and spores. These effects may be related to enzyme productions such as chitinase, α-glucanase, celullase, proteases, among others (Harman 2006). Similar processes were reported against Pythium oligandrum Dreschler, Rhizoctonia solani Kühn, F. oxysporum, Phytophthora megasperma Dreschler and Pythium ultimum Trow (Benhamou et al. 1999).
Antibiosis of Trichoderma is augmented because of the competition with other pathogens. The application of culture filtrates containing secondary metabolites produced by the 4 Trichoderma species in agar plates, reduced the growth of Fusarium mycelia. These results coincide with many other works and depend on the species tested (Bolar et al. 2000, Reino et al. 2008, Li et al. 2015, Moosa et al. 2016).
Competition for nutrients and space, also depend on the Trichoderma species and the pathogen (Infante et al. 2009). Martínez et al. (2008), evaluated 59 Trichoderma isolates and reported a strong competition for the substrate, especially competing for nitrogen, carbohydrates and macronutrients. The beneficial effect of Trichoderma on the protection of tomato plants in vivo under laboratory conditions, demonstrate that T. spirale strain used in the experiments, protected the plants again the pathogen and also improve their agronomic variables, possibly because improving water and nutrient intake by the plants (Santander et al. 2003, Patel & Saraf 2017). The increased dry weight tomato plant stems and roots by different Trichoderma species has been reported previously (i.e. T. koningiopsis (Moreno et al. 2009), T. harziarum (Li et al. 2015) and T. asperellum (Li et al. 2018)). Altomare et al. (1999) established in in vitro conditions that T. harzianum was able to solubilize phosphates and micronutrients such as Zn and Mn in liquid cultures. In other experiments, the use of nitrogen fertilizers was reduced to 33% by applying different Trichoderma species (GuigónLópez & González 2004, Harman 2006).
In field experiments, T. spirale produced best results after 90 days post-transplanting both by production and by protecting plants against Fusarium wilt (Figure 5). Plant height and number of harvested fruits indicated the benefit of using T. spirale over the other 3 used species. Kleifeld & Chet (1992) and González-Cárdenas et al. (2005) indicated an increase of production in tomato plants and a good control of Fusarium using different isolates of T. harzianum; however the results did not show any improvement on the measured variables when T. harzianum was used. Bal & Altintas (2008) also demonstrated that application of T. harzianum did not increase yield in tomato. On the other hand, integrated management of Fusarium wilt in tomatoes using cow manure and a combination of T. viride and T. harzianum produced the best inhibition and showed lower levels of disease severity in in vivo experiments (Moosa et al. 2016). The use of T. spirale as biological control agent is uncommon and few reports on its biological characterization are available in the literature. Hakim et al. (2015) proved a high antifungal index against Fusarium, endophytic behavior and phosphate solubilizing effect of a T. spirale strain from Indonesia but in in vitro conditions. Conversely to the results, T. spirale had low effect controlling F. oxisporum in eggplants under fields conditions comparted to T. hamatum and T. virens (Abdel-Monaim et al. 2014). As it has been mentioned, the responses varied according to the species/strain of Trichoderma and even the beneficial effect of Trichoderma, is also modulated by the tomato plant genotype (Tucci et al. 2011).
In Venezuela commercial products available are formulated almost exclusively with T. harzianum but in experimental trials have failed to control Fusarium compared to noncommercial species (Salazar et al. 2011). The authors attributed that lack of effectiveness of the commercial products to problems related to shelf life or in store handling, however in many cases, some Trichoderma isolates commercialized in the country have been imported from other latitudes and probably their performance is compromised due to different environmental conditions. According to the results, the native T. virens strain tested in this work showed a superior performance in both protecting and improving plants production, compared to the other species tested. More research is mandatory in order to evaluate this strain against other pathogens and crops in order to improve development of a formulated product.