Introduction
Gamete handling standardization procedures are a necessary tool for the development of hatchery techniques and for the use of gametes in laboratory research (Beirão et al., 2019; Fabbrocini et al., 2023; Ramos-Júdez et al., 2019). In this way, optimal gametes preservation for a short period before fertilization is a valuable tool to the use of samples after their collection (Contreras et al., 2020).
Short-term gametes storage is an inexpensive and simple technique that allows the use of the same batch of eggs or sperm at different times, maximizing the application of research protocols and the use of gametes in production (Bokor et al., 2021; Cejko et al., 2022; Kristan et al., 2020; Yasui et al., 2015). Besides, using short-term storage techniques, gametes transportation from broodstock-rearing sites to other teachings, laboratory research, or aquaculture facilities is possible (Kiyomoto, 2019). In the last decade, the welfare of natural populations of invertebrates has become a greater concern, highlighting the importance of minimizing animal collection for research, education, or production (Beirão et al., 2019; Fabbrocini et al., 2023; Rubilar & Crespi-Abril, 2017). Short-term gamete storage enables the reduction of animal collection by sharing gamete samples for laboratory research, which is also in accordance with recommended guidelines such as ARRIVE (Animal Research: Reporting of In Vivo Experiments, https://nc3rs.org.uk/arrive-guidelines).
Most procedures for gametes handling in aquatic species have been developed for aquaculture purposes and mostly for fish (Bokor et al., 2021; Kristan et al., 2020; Ramos-Júdez et al., 2019). However, in recent years, the development of breeding methods to reproduce model species on a laboratory scale has increased (Cirino et al., 2017; Fabbrocini & D’Adamo, 2011; Fabbrocini et al., 2021; Tsang et al., 2017). Echinoderms are excellent animal models among marine organisms, it is a Phylum of invertebrates exclusively marine that plays important ecological roles in each environment they inhabit (Brusca & Brusca, 2003; Chiarelli et al., 2019; Matranga & Corsi, 2012; McClay, 2011). Sea urchins and starfish embryos have been used for embryological studies (Boveri, 1901; Briggs & Wessel, 2006; Dufossé, 1847; Garner et al., 2016; McClay, 2011; Williams & Anderson, 1975), ecotoxicology analysis (Rahman et al., 2009), and more recently for neurogenesis and molecular studies (Garner et al., 2016; Hinman & Burke, 2018; McClay, 2011). In addition, throughout history, humans have been consuming both sea urchins and sea cucumbers, being highly valued fishing and aquaculture resources, considered a gastronomic delicacy in many regions of the world (Lawrence, 2007; Stefansonn et al., 2017; Sun & Chiang, 2015).
The green sea urchin Arbacia dufresnii (Blainville, 1825) is an abundant coastal species from the coast of Buenos Aires in Argentina (35⁰ S) to Puerto Montt in Chile (42⁰ S) including some islands of the South Atlantic Ocean (Bernasconi, 1947; Brogger et al., 2013). In recent years, great advances have been made in the aquaculture management of A. dufresnii, optimizing its nutrition, water quality parameters, culture density in recirculating aquaculture systems, and early developmental culture (Chaar et al., 2021; Fernández et al., 2021; Sepúlveda et al., 2021; Vera-Piombo et al., 2022). Additionally, significant advancements have been made in the purification of pigment extracts from gametes, which can be utilized in the development of nutraceutical products and potentially beneficial for human health (Avaro et al., 2022; Barbieri et al., 2021; Rubilar et al., 2021). All this knowledge made it possible that A. dufresnii is now a species with great aquaculture potential and as a source for the nutraceutical industry (Rubilar & Cardozo, 2021; Rubilar et al., 2016). However, there is still a lack of information on the appropriate handling and storage of gametes in A. dufresnii, which is of great value to scale up its productivity. Therefore, the aim of this study was to determine the effect of male and female gamete aging on the fertilization success of the sea urchin A. dufresnii in order to optimize the use of gametes after collection decoupling spawning from fertilization.
Materials and methods
Collection and maintenance of experimental animals: 30 A. dufresnii adults (30-35 mm test diameter) (Epherra et al., 2015) were collected by scuba diving in Nuevo gulf, Argentina (42º 46’ 44’’ S & 64º 59’ 52’’ W). Individuals were transferred, in thermal containers with seawater and controlled temperature, to the Experimental Aquarium of the CCT-CONICET-CENPAT in Puerto Madryn, Chubut, Argentina. For acclimatization, the individuals were kept for seven days in recirculating 100 L tanks under similar to local natural conditions of temperature and salinity (12 ⁰C ± 1, 35 ± 1 ppm) and starvation.
Spawning induction and collection of gametes: Males and females were induced to spawn by injection through the peristomial membrane of 0.3 mL of 0.5 M KCl solution (Strathmann, 1987). To account for the fertility variability observed among individuals of A. dufresnii (Fernández et al., 2021), standardized fertilization was performed in each bioassay by utilizing an egg pool from five females and a sperm pool from five males. Eggs were collected on filtered and UV sterilized seawater and placed in a single container, generating a pool of eggs. Then, the pool of eggs was quantified in triplicate in a Sedgewick Rafter chamber using a Leica DM 2 500 microscope. Sperm was collected dry in a single container and kept on ice for further processing.
Experimental design: Two bioassays were performed to test the effect when both types of gametes are aged and when one type of gametes is aged. In both bioassays, each treatment and the control had 3 replicas. Each replica consisted of a 250 ml glass beaker, with filtered seawater conditioned through a series of mechanical filters (10, 5, and 1 µm) and sterilized (UV filter), constant aeration, the temperature of 12 ⁰C ± 1, the salinity of 35 ± 1 ppm and photoperiod (12:12 h). Each container was independent and contained about 100 000 eggs.
Aging Gametes: To age the eggs, they were placed in the glass beakers filled with seawater and kept at a temperature of 12 ± 1 °C during each bioassay. As for the sperm, two different ways were used: activated sperm, which consisted of a pool of sperm in seawater (1: 1 000 v/v) that was kept at a temperature of 12 ± 1 °C during each bioassay, and dry sperm, which consisted of a pool of sperm that was kept dry at the same temperature during each bioassay. Before each fertilization, an aliquot of the dry sperm was resuspended in seawater to activate it (1: 1 000 v/v), and then it was immediately used to fertilize the eggs.
Bioassay 1. Fertilization success with both gametes aged over time: The impact of gametes aging in fertilization success was investigated by performing fertilization tests with gametes aged for 0 h, 24 h, 48 h, 72 h, and 96 h (Table 1). At each time point, eggs were fertilized with 1: 100 000 v/v sperm in seawater. The sperm used for fertilization were either activated sperm or dry sperm. Fertilization success was daily checked by observing the appearance of the fertilization envelope after 30 minutes of launching the assay. The fertilization percentage was calculated using the formula F = Fertilized eggs/total eggs x 100.
Table 1 Assay design to study fertilization success of simultaneous aging of male and female gametes. E: Eggs, AS: Activated sperm, DS: Dry sperm.
Fresh | 24 h Aged | 48 h Aged | 72 h Aged | 96 h Aged | |
E x DS | 0 h (E X DS) | 24 h (E X DS) | 48 h (E X DS) | 72 h (E X DS) | 96 h (E X DS) |
E x AS | 0 h (E X AS) | 24 h (E X AS) | 48 h (E X AS) | 72 h (E X AS) | 96 h (E X AS) |
Bioassay 2. Effect of aged gametes on fertility: To determine the contribution of aged sperm and eggs to the decline in fertilization success, two separate spawning and fertilization tests were conducted. The fertilization success of aged sperm was evaluated individually by combining fresh eggs (0 h) with aged activated sperm (96 h) and aged dry sperm (96 h), as well as using fresh sperm (0 h) as the control (Table 2). Similarly, the fertilization success of aged eggs was assessed by conducting a fertilization test with fresh sperm (0 h) and aged eggs (96 h), as well as using fresh eggs (0 h) as the control (Table 2).
Table 2 Bioassay design to evaluate the incidence of aged sperm or aged eggs on the fertilization success. E: Eggs, S: Sperm, AS: Activated sperm, DS: Dry sperm.
Fresh sperm (S) | Aged dry sperm (DS) | Aged activated sperm (AS) | |
Fresh eggs (E) | E (0 h) X S (0 h) | E (0 h) X DS (96 h) | E (0 h) X AS (96 h) |
Aged eggs (E) | E (96 h) X S (0 h) | - | - |
Data analysis: For bioassay 1, a Generalized Linear Model (GLM) was applied to analyze fertilizing success over time after spawning. As a first step, a graphical exploration based on scatter and residual plots of the data was performed to understand the relationship between time and fertilizing success. Different replicates were included in the model analysis to evaluate method error and to determine if the replicates presented similar values. Starting with a null model, different models were created, incrementing the complexity of the models by combining time (h) and type of gametes combined (Eggs x Activated sperm or Eggs x Dry sperm) in a double interaction model. The best model was selected based on the Akaike criteria, taking into account residual analysis, explained variance (deviation), and the principle of parsimony or normality (Hurvich et al., 1998). For bioassay 2, one-way ANOVA was applied to analyze the contribution of each type of aged gamete on the decline in fertilization success. Normality was tested using the Shapiro-Wilks test and homoscedasticity of variance was tested using the F-test (Zar, 1984). All GLM analyses were performed with the free software RStudio (version 3.5.1) (R Core Team, 2016). The software InfoStat (InfoStat, 2016) was used for ANOVA, Shapiro-Wilks, and F-tests.
Results
Bioassay 1. Fertilizing success over time after spawning: The fertilization success of eggs and sperm decreased over time, being less than 30 % by the end of the experiment at 96 h (Fig. 1). Eggs combined with dry sperm presented high values of fertilization success (95.1 ± 0.7 %) for up to 48 h. Afterwards, the fertilization success fell rapidly, with only 26.5 ± 1.4 % of eggs being fertilized at 96 h. On the other hand, fertilization success of eggs combined with activated sperm was high (96.1 ± 1.3 %) only for 24 h, values fell slightly at 48 h and finally fell rapidly with only 15.5 ± 2.5 % of eggs being fertilized at 96 h. GLM analysis revealed that the type of gametes combined (Eggs x Activated sperm or Eggs x Dry sperm) in interaction with time (h) influences fertilization success (Table 3).
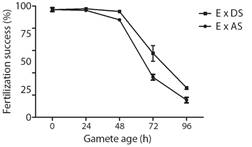
Fig. 1 Fertilization success of both eggs and sperm over time calculated as percent fertilization (Mean ± SEM, n = 3) at different times after spawning in A. dufresnii. E: eggs, DS: Dry sperm, AS: Activate sperm.
Table 3 GLM analysis for fertilization success in A. dufresnii of both eggs and sperm over time. In bold, the model with the best fit according to the Akaike information criteria (AIC).
Model | AIC | DIF | |
1 | Null | 1 296.79 | 107.85 |
2 | Type of gametes combined | 298.28 | 109.33 |
3 | Tiempo (h) | 212.88 | 23.94 |
4 | Type of gametes combined + time | 201.34 | 12.40 |
5 | Type of gametes combined * time | 188.94 | 0.00 |
Bioassay 2. Effect of aged gametes on fertility: Fertilization success presented significant differences when fresh eggs (0 h) were combined with the three types of sperm (F2,6 : 191.28, p < 0.0001) (Fig. 2). The fertilization success of 96 h aged dry sperm decreased to 51.1 ± 2.2 %, and the fertilization success of 96 h aged activated sperm decreased to 13.1 ± 3 %, whereas fresh sperm (0 h) achieved a success rate of 86.8 ± 2.8 % (Fig. 2). Similarly, significant differences were observed in the fertilization success when fresh sperm (0 h) was combined with the two types of eggs (F1,42 : 3.35, P < 0.05). The fertilization success with 96 h aged eggs decreased to 72.3 ± 4.8 % when compared to fresh eggs (0 h) that achieved a success rate of 96.8 ± 1.7 % (Fig. 3).
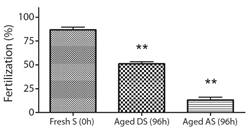
Fig. 2 Fertilization success of fresh eggs combined with fresh sperm (Fresh S), 96 h aged dry sperm (Aged DS), and 96 h aged activated sperm (Aged AS), calculated as the percentage of fertilization success (Mean ± SEM, n = 3) in A. dufresnii. S: sperm, DS: Dry sperm, AS: Activate sperm. The asterisk (**) indicates treatments that showed significant differences (P < 0.0001).
Discussion
Under laboratory conditions, the influence of gamete age on fertilization success is one of the main limitations to optimize the use of samples after their collection (Contreras et al., 2020). In general, a higher fertilization success rate (over 80 %) is desirable as it increases the efficiency of gamete utilization and reduces the cost of producing embryos or larvae (Fabbrocini et al., 2021; Lera & Pellegrini, 2006). However, depending on the context and goals of the study or application, a fertilization success rate of 50 % may be considered acceptable. For example, if the goal is to obtain a large number of sea urchin embryos for education, a fertilization success rate of 50 % may be acceptable as long as a sufficient number of embryos are obtained (Epel et al., 2004; Kiyomoto et al., 2014). On the other hand, if the goal is to produce sea urchin larvae for aquaculture purposes, a higher fertilization success rate may be necessary to meet the industrial requirements (Contreras et al., 2020; Fabbrocini et al., 2021).
The findings of the present study show that the fertilization success in the sea urchin A. dufresnii gametes remains relatively unchanged for up to 48 h after spawning. Although there is a slight decrease in fertilization success between 24 and 48 h when using activated sperm, using both types of gametes combinations (Eggs x Activated sperm or Eggs x Dry sperm) results in a fertilization success rate over 87 %, which represents a high fertilization success. In practice, this means that both female and male gametes of A. dufresnii can be preserved for 48 h post-spawning with a very high probability of fertilization between them. Additionally, the sperm can be kept dry or activated for up to 48 h. This aspect is highly variable between different marine invertebrates and even within the same taxon, being necessary to determine in each species in order to standardize its handling. For example, in the asteroid Asterias rubens, fertilization reaches 100 % only during the first 4 h after spawning and then falls to 0 % at 24 h (Williams & Bentley, 2002), while in eggs of the echinoid Strongylocentrotus droebachiensis fertilization success is high for 48 to 72 h (Meidel & Yund, 2001).
On the other hand, we found important differences in fertilization success when combining eggs with dry sperm or activated sperm of A. dufresnii at 72 and 96 h of gamete aging. Further analysis using generalized linear models (GLM) confirmed that the effect of time on fertilization success varied depending on the type of gamete combination used, with Eggs x Activated sperm exhibiting lower fertilization success rates. This difference may be due to the fact that activated sperm is activated at the beginning of the bioassay (0 h) and is allowed to age, which results in the depletion of its energy reserves and a noticeable reduction in fertilization capability after 72 h. In contrast, dry sperm was aged and activated immediately before each fertilization, enabling it to retain its energy reserves and maintain a higher fertilization capability. Under laboratory conditions, Eggs x Dry sperm showed a fertilization success rate of over 50 % at 72 h, making it a viable option for certain purposes. In contrast, the use of Eggs x Activated sperm aged 72 h, which exhibited a fertilization success rate of 36 %, would not be advisable. At 96 h, Eggs x Dry Sperm still had a higher fertilization success rate compared to Eggs x Activated sperm, but the overall fertilization success rate for both types of gametes combinations was less than 30 %, indicating that using either dry or activated sperm to fertilize aged eggs would not be recommended. Therefore, this means that if both the female and male gametes of A. dufresnii are aged simultaneously, acceptable fertilization rates can be achieved up to 72 h only using dry sperm.
When analyzing the results of bioassay 2, it becomes evident that each type of aged gamete contributes differently to the decline in fertilization success. Furthermore, it is observed that the fertilization success after 96 h of gamete aging differs between male and female gametes. This observation is consistent with prior research, which has indicated that the process of sperm aging is more pronounced than that of eggs and that the rate of sperm aging accelerates with increasing sperm dilution (Benzie & Dixon, 1994). In A. dufresnii, a significant decrease in fertilization success was observed after 96 h of gamete aging when combining aged sperm (96 h) with fresh eggs (0 h). Notably, the decrease was significantly more pronounced in the case of activated sperm (96 h) compared to dry sperm (96 h). Therefore, under laboratory conditions, it would be possible to use aged dry sperm (96 h) to fertilize fresh eggs (0 h) and obtain a fertilization success of approximately 50 %. On the contrary, it is not recommended to use aged activated sperm (96 h) combined with fresh eggs, since fertilization success was under 30 %. When evaluating the fertilization success of the aged eggs (96 h) combined with fresh sperm (0 h), a decrease of less than 25 % is observed. Therefore, under laboratory conditions, it could be feasible to use eggs for up to 96 h and obtain acceptable fertilization percentages when combining them with fresh sperm. In practice, this implies that the extension of fertilization capability of aged eggs and dry sperm up to 96 h can be achieved by combining fresh gametes. These findings could have significant implications for the management of A. dufresnii gametes, including the development of hatchery techniques and their use in education and laboratory research (Beirão et al., 2019; Fabbrocini et al., 2023; Ramos-Júdez et al., 2019). However, in order to ensure viable offspring, a more extended study would be necessary, analyzing the normal development of A. dufresnii progenies from aged gametes. Nonetheless, these results provide valuable insights into the aging process of A. dufresnii gametes and offer a foundation for future studies on the management and conservation of this species.
Taken together, the results of this work suggest that the fertilization success of aged gametes in A. dufresnii is influenced by several factors, such as the type of aged gamete, the duration of aging, the dilution of sperm, and the gamete combination. In addition, in some studies, it has been possible to obtain a longer duration of the gametes by avoiding bacterial proliferation, since it is the main cause of egg deterioration. Epel et al. (2004), described for the first time that it is possible to satisfactorily increase the post-spawning time by keeping gametes in antibiotic-modified seawater. In another study, Kiyomoto et al. (2014) evaluated the egg storage conditions of five sea urchin species in antibiotic-modified seawater and found that depending on the species there are differences in the time and temperature range used in storage. This evidence indicates that there are multiple factors to take into account for the conservation of sea urchin gametes, such as temperature, the application of antibiotics, and the species of sea urchin analyzed. Knowledge of the proper management of each of these variables could even improve the survival time described for A. dufresnii gametes and their effectiveness in fertilization. In this context, in the present work the first steps have been taken to understand the conservation of A. dufresnii gametes with the minimum possible intervention, that is, in filtered and sterilizer seawater, temperature similar to the environment and without the addition of antibiotics or other substances. Based on these results, further research can be conducted to understand the survival of A. dufresnii gametes and to explore modifications to seawater conditions, and the use of antibiotics, that can potentially increase their viability and fertilization success.
Ethical statement: the authors declare that they all agree with this publication and made significant contributions; that there is no conflict of interest of any kind; and that we followed all pertinent ethical and legal procedures and requirements. All financial sources are fully and clearly stated in the acknowledgments section. A signed document has been filed in the journal archives.