Introduction
Sea urchins of the genus Diadema can conquer different habitats like coral reefs and rocky grounds, due to their generalist diet (Benítez-Villalobos et al., 2015). Even though, sea urchin Diadema mexicanum (A. Agassiz, 1863) are mainly herbivores and feed mostly on algae, it is also common to see them feeding on living and dead corals (Cabanillas-Terán et al., 2016; Glynn & Morales, 1997; Tribollet & Golubic, 2011). Added to this, this species plays an important role in the Eastern Tropical Pacific (ETP) due to its bioeroding action on coral reefs (Alvarado et al., 2016a), which has intensified in El Niño events (Eakin, 2001).
When feeding, D. mexicanum scrapes the surface where the food is located, sometimes causing bioerosion to the substrate (Benítez-Villalobos et al., 2008; Hutchings, 2011). Grazing made by sea urchins can be advantageous in some specific cases, as it happens for algae, whose development and abundance gets restricted, helping corals to easily obtain light and space (Herrera-Escalante et al., 2005; McClanahan et al., 1996). Similarly, they also have an impact on rocky reefs, because they control organisms that grow over them, however high densities of this sea urchins could lead to a negative impact, due to the high abrasion they cause, changing the reef structure (Alves et al., 2003; Eakin, 2001).
Feeding of this sea urchin is related to many dynamic processes of the ecosystem and its composition. Gut content found inside the sea urchin can be divided in three categories: organic matter (OM), non-carbonated fraction (NCF) and carbonated fraction (CaCO3). This depends on what the sea urchins feed on and the substrate in which they obtained their food. Food availability in the zone and food preference will consequently influence the gut content (Alvarado et al., 2015).
Due to this, knowing the amount of matter that the sea urchins are consuming according to their stage of development is important, since it makes it possible to quantify their selection of bio-eroded substrate. Taking this into account, determining whether the location of D. mexicanum influences stomach content can be a relevant factor to slow down coral reef degradation. Here, we thereby determine if there is a difference in the gut content of D. mexicanum according to the study site and development stage and establish if there is a correlation between their gut content and their age. We predict that there is going to be a difference between stomach contents of D. mexicanum according to their age and locality. We expect there to be a significant correlation between the sea urchin size and the stomach content type.
Materials and methods
This study was carried out in 12 localities with coral reefs in the Eastern Tropical Pacific (ETP) (Fig. 1), which included continental, peninsular and insular environments, both protected and non-protected areas (Alvarado et al., 2016a, Alvarado et al., 2016b).
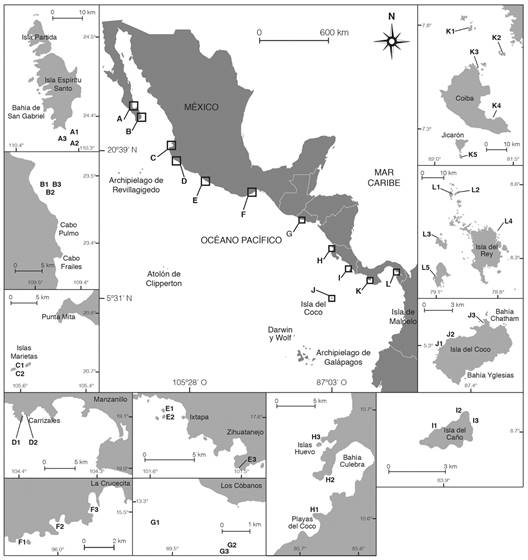
Fig. 1 Diadema mexicanum study localities along in the ETP. A. Isla Espíritu Santo; B. Cabo Pulmo; C. Islas Marietas; D. Carrizales; E. Ixtapa-Zihuatanejo; F. Bahías de Huatulco; G. Los Cóbanos; H. Bahía Culebra; I. Isla del Caño; J. Isla del Coco; L. Isla Coiba; M. Archipiélago de Las Perlas.
Diet composition was determined by collecting the evacuated gut content (EGC), and organic matter (OM), calcium carbonate fraction (CaCO3) and non-carbonated fraction (NCF) were analyzed. To obtain the ECG, 30 individuals per locality were collected (50 in Coiba and Las Perlas), from January 2009 to September 2010, and placed in 10 L plastic containers with continuous ventilation, letting them evacuate for 24 hours (sensuGlynn, 1988; Reyes-Bonilla & Calderón-Aguilera, 1999). The evacuated material by each individual sea urchin was dried in an oven at 60 ºC for 24 hours. The samples were weighed in a top loading balance (0.001 g), and then combusted for 6 hours at 550 ºC to burn the OM. The OM starts burning at 200 ºC and gets completely burned away at 550 ºC, the carbonates are not affected (Griffin et al., 2003). After cooling, the samples were weighed again, and the difference in mass was the organic matter present in the material evacuated (Carreiro-Silva & McClanahan, 2001). The remaining fraction represents the inorganic fraction that consisted of calcium carbonate (CaCO3) and insoluble residues (rock fragments, quartz grains, sponge spicules, diatoms, radiolarians and silt). The inorganic fraction was digested in 10 % hydrochloric acid and after the dissolution of CaCO3 filtered using pre-weighed fiberglass filters. The weight of the residual material retained on the filter corresponds to the non-carbonated fraction (NCF). The difference between the inorganic fraction weight and the NCF was CaCO3 (Carreiro-Silva & McClanahan, 2001). All sea urchins were measured with a caliper, and it was established that a size greater than 2.5 cm corresponds to an adult organism, while those less than or equal to said size were considered juveniles according to Benítez-Villalobos et al. (2015).
A Wilcoxon test (W) was conducted to determine the difference in stomach content between size groups. The difference in stomach content between localities was analyzed using a non-parametric Kruskal-Wallis. Finally, a linear regression model with a Kendall correlation was made to obtain the correlations between their different stomach fractions (g) and their size (cm). All these analyses were performed using R studio (v4.2.2.) (R Core Team, 2023).
Results
The EGC of 160 juvenile and 210 adults of D. mexicanum were analyzed. The organic matter (OM) (W = 11 694, p < 0.001), carbonated fraction (CaCO3) (W = 3 980.5, p < 0.001) and non-carbonated fraction (NCF) (W = 4224.5, p < 0.001) varied between both sizes. Juveniles presented an average of 0.3708 ± 0.1745 g of CaCO3, 0.1141 ± 0.0795 g of OM and 0.0606 ± 0.075 g of NCF, while adults presented 1.3527 ± 1.2244 g of CaCO3, 0.1956 ± 0.2563 g of OM and 0.2553 ± 0.2579 g of NCF on average (Fig. 2). Also, the carbonated fraction increased 0.47 g on average for each cm of growth of D. mexicanum (R𝜏 = 0.5690, p < 0.05, Fig. 3), while the organic matter only increased 0.05 g per cm (R𝜏 = 0.2630, p < 0.05, Fig. 4).
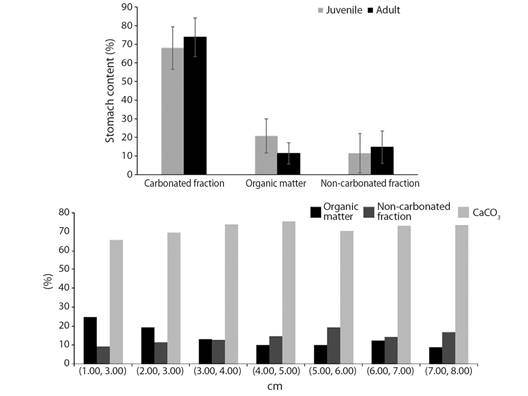
Fig. 2 A. Percentage of the stomach content of Diadema mexicanum according to its development stage. The error bars represent the standard deviation; B. % Gut content per size frequencies of test’s (cm).
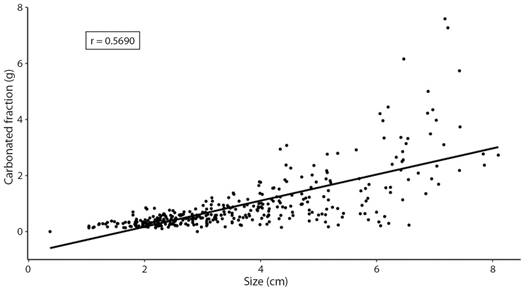
Fig. 3 Correlation of Diadema mexicanum between its size and the carbonated fraction, per individual.
Overall, Coco’s Island presented the highest average of the three fractions of the gut content (CaCO3 = 2.2519 ± 1.1802 g, OM = 0.5380 ± 0.5293g, NCF = 0.7525 ± 0.2909 g). Coiba had the lowest averages of CaCO3 (0.4179 ± 0.2710), while the lowest value of OM was presented in Pulmo (0.0892 ± 0.0281 g) and the lowest average of NCF was obtained in Huatulco (0.0418 ± 0.6633 g). Also, significant differences were obtained between CaCO3 (χ2 = 144.9, df = 11, p < 0.001), OM (χ2 = 135.66, df = 11, p < 0.001) and NCF (χ2 = 213.67, df = 11, p < 0.001) by locality (Fig. 5).
Discussion
The amount of carbonates per individual per size class (Fig. 2) was similar to the ones observed for Diadema savigny (Audouin, 1809), Echinothrix diadema (Linnaeus, 1758) and Echinostrephus molaris (Blainville, 1825) in French Polynesia (Bak, 1990) or for D. antillarum in Barbados (Scoffin et al., 1980) (Table 1). Also, similar to the values found by Herrera-Escalante et al. (2005) for D. mexicanum in Huatulco, 0.47 g CaCO3 ind-1 for sea urchins smaller than 3 cm, 1.41 g CaCO3 ind-1 for urchins between 3-5 cm and 2.96 g CaCO3 ind-1 for urchins between 5 and 7 cm of test diameter.
Table 1 Average carbonate grams per individual (g CaCO3 ind-1) in Barbados (D. antillarum; Scoffin et al., 1980), French Polynesia (D. savigny, E. diadema, Echinostrephus molaris; Bak, 1990) and in ETP (D. mexicanum; this research).
Class interval | Barbados | French Polynesia | ETP |
(1.00, 2.00) | 0.273 | 0.01 | 0.277 |
(2.00, 3.00) | 0.507 | 0.04 | 0.404 |
(3.00, 4,00) | 1.292 | 0.16-0.36 | 0.705 |
(4.00, 5.00) | 1.490 | 0.71-1.06 | 1.150 |
(5.00, 6.00) | 2.264 | 1.87-3.03 | 1.290 |
(6.00, 7.00) | 4.32-6.81 | 2.478 | |
(7.00, 8.00) | 3.886 | ||
(8.00, 9.00) | 2.728 | ||
Average | 1.165 | 1.185-1.885 | 1.435 |
Hawkins (1981) and Hawkins and Lewis (1982) found a change in the percentage composition of organic matter among sea urchins bigger than 3.5 cm, as we found. The percentage of encrusting coralline algae in the gut content of D. antillarum changes between sizes (Hawkins & Lewis, 1982). In sea urchins smaller than 3.5 cm, these algae represent between 70-80 %, while epilithic or endolithic algae represent between 5 % and 20 %. In sizes over 3.5 cm, encrusting coralline algae become a minor element of the gut content (5-20 %), while epilithic algae represent between 50 and 75 %. Sea urchins smaller than 3.5 cm present a higher growth rate (3.4 mm month-1) than sea urchins above this size (1.5 mm month-1) (Hawkins & Lewis, 1982). When sea urchins grow, their diet varies from encrusting coralline algae (with high organic matter content) to epilithic algae (with low organic matter content). The highest growth rates in small sea urchins require a higher quality diet with an organic content richer than the diet of sea urchins over 3.5 cm. Additionally, sea urchins that eat encrusting coralline algae possess an absorption efficiency ranging between 26-72 %, while urchins that eat other algae possess an efficiency between 1 % and 24 % (Hawkins, 1981). In the ETP, 51 % of sea urchins are under 4 cm (Fig. 4). Also, in seven of the studied localities, between 53 % and 100% of the collected individuals were under 4 cm, highlighting the abundance of young urchins. It seems that small individuals prefer calcareous algae, which give them the necessary energy requirements to grow fast and reach adulthood.
Regarding the development of the sea urchins, it has been seen that their growth and gonad development do not occur at the same time (Lawrence, 2000). On the contrary, usually these individuals focus on one process or another, both requiring nutrients, both limited by food availability (Lawrence, 2000). The transition from a larval state to a juvenile has not been studied deeply, and this process involves a drastic change in the urchin’s diet (Fadl et al., 2018). There is a period of time, specifically at the beginning of this process, where the urchin is deprived of nutrients, which is related to the development of Aristotle’s lantern (Fadl et al., 2018).
What the sea urchins eat during the first juvenile stages is unknown and the change of stage can lead to a different diet (Fadl et al., 2018). Also, it has been proven by experiments that sea urchins with a more calcareous diet do not get to change into an adult stage, meaning they never develop their gonads to a point of sexual maturity (Meidel & Scheiblingl, 1999). This implies that the idea and presence of the organic matter and proper nutrients are necessary during juvenile development stages, which means that a diet based on more organic matter in the early stages is to be expected (Meidel & Scheiblingl, 1999).
In the case of the general increase of the stomach content related to size, D. mexicanum gonads grow in proportion to their size (López-Pérez & López-López, 2016). The gonads size not only depends on the increase in number and size of gametes, but also depends on the phagocytes and the nutrient storage needed for the start of gametogenesis. These nutrients need to be recovered with food since individuals who do not eat or do not have a good diet tend to either have smaller gonads or not have them at all (Muthiga & McClanahan, 2007).
Then, the increase in size could be related to a strategy for the urchin to prepare for a reproductive stage. An example of this has been seen in the green sea urchin, Strongylocentrotus droebachiensis (O.F. Müller, 1776), which presents a mobilization of nutrients from the nutritive phagocytes, indicating a new gametogenesis, surrounding germ cells with the released nutrients (Benítez-Villalobos et al., 2015). Also, it has been seen that the size is directly proportional to the amount of carbonates consumed (López-Pérez & López-López, 2016), which is supported by the non-parametric test done (Kruskal, p < 0.001).
The size of the sea urchins can increase due to external factors not directly related to nutritive requirements, which is the case for the genus Diadema, where it has been seen that, in some occasions, when the population density is low, the individual size increases (Benítez-Villalobos et al., 2008). This happens because sea urchins are considered density-dependant, where whenever there are fewer individuals, the resource availability increases, leading to a better general diet (Benítez-Villalobos et al., 2008). Another case where this happens is depending on the substrate of the localities (López-Pérez & López-López, 2016). Generally, the size and density of the organisms changes according to the size of the site, for example, places with bigger rocks tend to have bigger sea urchins (López-Pérez & López-López, 2016).
Lastly, coral reefs are very susceptible to environmental changes, which can create differences between them throughout the Eastern Tropical Pacific (Alvarado et al., 2016a). D. mexicanum lives in these ecosystems, which could have been modified by El Niño of 1982-1983, which generated a high mortality rate in the corals, and subsequently an increase in macroalgae growth, that populated the site, considerably increasing their coverage (Alvarado et al., 2016a). This phenomenon is important because the bioerosion of D. mexicanum is approximately twice as high in dead coral substrate (Glynn, 1988), which influences considerably, because the sea urchins arrive at these affected places in search of food and this leads to a localized abrasion of the calcareous deposits (Herrera-Escalante et al., 2005).
Another factor that goes hand in hand with the disturbances in the reefs of the ETP zone is that these are very susceptible to erosive changes due to their little development, meaning any damage would be more drastic. The higher bioeresion rate could also be attributed to D. mexicanum generally found in groups (Herrera-Escalante et al., 2005). The feeding of urchins can also have an impact on carbonate levels and algal cover of coral reefs (Alvarado et al., 2016a). Variations in the ecosystems and conditions, like the ones described above, can cause differences in feeding, so, a change can be observed in the type of stomach content in relation to the location.
As a recommendation, for other studies like this one, it is important to note that adult sea urchins have high nutrient stages, where they seem to prepare for their reproductive stage and spawning (Benítez-Villalobos et al., 2015). This is an important consideration since the season for the data collection can influence the results. Another aspect to consider is that some of the areas of study were protected areas and that not all of them have the same level of conservation (Alvarado et al., 2016a). This is important since it has been reported that areas with overfishing, unprotected areas or less protected areas, usually present higher densities of sea urchins and a more noticeable bioerosion effect (Graham et al., 2011).
In conclusion, it seems that the life stage of the sea urchin does influence the stomach content, and the juveniles tend to consume a higher percentage of organic matter related to the necessity of the early-stage sea urchins for nutrients to maintain their growth. The amount of OM is crucial for the development during this stage, meaning that there is a difference in substrate selection associated with growth.
Aside from this, the locality, which relates to the kind of substrate and the availability of nutrients is key to the diet of D. mexicanum. At last, there is an important correlation between the size of the urchin and their stomach content, which states that whenever the size increases, all of the stomach content increases too, being most noticeable in the case of the carbonated fraction. Also, the percentage distribution of the stomach content significantly differs, where it is seen that the carbonated fraction has double the percentage of consumption in the case of juveniles compared to adults. All of this could suggest that in case of a situation of D. mexicanum overpopulations, the adults are going to be causing more damage to the ecosystem and mostly to corals, leading to a possible disbalance of carbonate levels and algal covers, so controlling the adult population is advised.
Ethical statement: the authors declare that they all agree with this publication and made significant contributions; that there is no conflict of interest of any kind; and that we followed all pertinent ethical and legal procedures and requirements. All financial sources are fully and clearly stated in the acknowledgments section. A signed document has been filed in the journal archives.