Introduction
The genus Echinaster comprises a total of 30 species, although this figure is still under debate since some new morphotypes are being described (Lópes et al., 2016; Seixas et al., 2019). These are medium-sized starfish whose color varies between yellow and red, with five cylindrical-conical arms and a small central area. Their skin is thick and covered with glands that offer them a good chemical defense, which probably explains the absence of pedicellariae in the Echinaster species (Turner, 2013). In the present work we focus on the study of one of the most emblematic species of the genus Echinaster: the one commonly known as red Mediterranean starfish, Echinaster sepositus (Retzius, 1783). This species belongs to the class Asteroidea, superorder Spinulosacea, order Spinulosida, family Echinasteridae, genus Echinaster and subgenus Echinaster. It was described by Aristotle in ancient Greece more than 2 300 years ago in the Historia Animalium, being the first starfish to be mentioned in science (Turner, 2013). In recent years it has been used as a model species for studies of systematics and morphology of asteroids (Lafay et al., 1995; Mah & Blake, 2012). Its distribution covers the entire Mediterranean basin and the temperate waters of the eastern Atlantic, from the southeastern limit of the English Channel to Cape Verde. It is a species that inhabits from shallow waters (less than 2 m) to depths of 250 m (Wirtz & Debelius, 2003) and can live in a wide variety of environments, such as soft sediment bottoms, rocky substrates, phanerogams seagrass or communities of coralline algae (Entrambasaguas et al., 2008). The spatial distribution of E. sepositus varies depending on the algal cover, the sand cover and the depth (Entrambasaguas et al., 2008). However, the exact causes of these variations remain unknown. Furthermore, the density of individuals varies among apparently similar locations, which suggests that it could be affected by factors, biotic or abiotic, that act on scales of hundreds of meters or even less (Chapman & Underwood, 2008; Underwood & Chapman 1996). E. sepositus feeds on detritus and small organisms that are found on the substratum by the evagination of their stomach towards the outside of the buccal opening (Vasserot, 1961). There are indications that they also feed on sponges, although the defensive systems of many species can repel the starfish (Waddell & Pawlik, 2000). Villamor & Becerro (2010) also observed a certain affinity of E. sepositus by areas where encrusting algae predominate, characterized by having associated small invertebrates and being a place of recruitment for many species of benthic invertebrates.
E. sepositus is a dioecious species, although some authors have described an “accidental hermaphroditism” in up to 4 % in the individuals studied (Cognetti & Delavault, 1962; Scheibling & Lawrence, 1982). The Mediterranean red starfish reproduces only sexually, through external fertilization, giving rise to a lecithotrophic larva whose period prior to settlement does not usually exceed a week (Turner, 2013). The gonads are found in pairs on each limb and communicate with the exterior by means of the gonopores, located on the side of the arms, near the central disc (Turner, 2013).
Lecithotrophic larvae have an energy reserve in the form of a yolk that is consumed at the time of settlement. This means, with respect to the planktotrophic larvae, a higher probability of successful recruitment and a higher survival of settlers (Byrne et al., 1999; Villinski et al., 2002), but a lower number of larvae emitted. The inability of the lecithotrophic larvae to feed also implies limited dispersal capacity in relation to planktotrophic larvae and can have large consequences on the genetic structure of their populations, although the high probability of settling near the areas inhabited by adults of the same species favors the location of an area good enough for their vital development (Cisneros, 2016). Species that have a lecithotrophic larvae have a buffer system through which they limit the large population fluctuations suffered by other echinoderms, both in decrease as well as population growth (Uthicke et al., 2009). It has been seen that in the northwestern Mediterranean Sea the spawning of gametes occurs during the months of summer, especially during June and July, when the starfishes have a greater gonadal weight (Villamor & Becerro, 2010). However, in September 2015, the spawning of E. sepositus was observed a few days apart at different points along the coast of Catalonia (Cisneros, 2016). On October 18, 2018, the emission of gametes of E. sepositus was recorded during a night dive in Cala del Racó (Calpe, Alicante) and the same group of researchers observed this phenomenon again the following week at the same time (Diana López, personal communication, 2018).
Gametogenesis in asteroids may be regulated by endogenous factors such as age, nutritional status or size, or by exogenous factors such as photoperiod, temperature or food availability (Mercier & Hamel, 2009). Among all these factors, the photoperiod is probably the most important factor in controlling the reproduction in asteroids, and not only the annual photoperiod, but also the daily one (Basch & Pearse, 2022; Byrne et al., 1999; Georgiades et al., 2006; Gibson et al., 2011; Pastor-de-Ward et al., 2007; Stewart & Mladenov, 1997). This influence has been corroborated by multiple manipulative experiments in different species of starfish (Pearse & Beauchamp, 1986; Pearse & Bosch, 2002; Pearse & Eernisse, 1982; Pearse & Walker, 1986). On the other hand, previous studies have described that the influence of nutritional status or food availability sometimes follows an inverse relationship with the gonadal index, so that the direct influence of feeding on the reproduction of asteroids remains unclear (Barker & Xu, 1991; Chen & Chen, 1992; Farmanfarmaian et al., 1958; Grange et al., 2007; Rubilar et al., 2005).
While predation on offspring can be avoided with parental care, species that do not protect the clutch develop alternative strategies to avoid high mortality thereof (Balshine, 2012). To avoid predation by diurnal animals, some groups synchronize the spawning of gametes during twilight or nocturnal hours, when mortality is considerably lower (Čech et al., 2005; Metcalfe et al., 1999). Despite the lack of previous scientific observations on the relationship between light and the laying of gametes in E. sepositus, the last observations of spawning in Cala del Racó were made during the night, which has led us to hypothesize that daily photoperiod would be influencing the spawning of this species, possibly to ensure greater offspring survival.
Despite being one of the most common starfish in the Mediterranean Sea, information about the biology of E. sepositus or its role in benthic communities is scarce and sometimes contradictory (Villamor & Becerro, 2010). Besides, some populations of E. sepositus in the northwestern Mediterranean have been reduced in the last decade. Among the main causes is the direct extraction of individuals for ornamental aquariums or souvenirs (Villamor & Becerro, 2010), practice that is widespread and that lacks an appropriate system of regulation (Olivotto et al., 2011). For this reason, the main objective of this work is to improve the knowledge of the ecology of this species through annual night monitoring via fixed sampling stations. The specific objectives are, in the first place, to perform a characterization with temporal monitoring of the population of E. sepositus in Cala del Racó (Calpe), where the spawning was recorded in 2018 and, in the second place, to try to record in situ, once again, the spawning of this species.
Materials and methods
Study area: The study took place in Cala del Racó (38º38’09’’ N & 0º04’16’’ E), in the town of Calpe (Alicante, Spain). The waters of Cala del Racó bathe the west of the “Peñón de Ifach”. This calcareous outcrop was declared a Nature Reserve by the Generalitat Valenciana (Decree 1/1987), on 1987, January 19. However, it was not until 1993 when a plan of park administration was approved. The marine area that surrounds the rock has been declared a Zone of Special Conservation Area for Birds (ZEPA) under (Directive 79/409/CEE, 1992) and includes a Site of Community Importance (SCI Espacio Marino de Ifach: ESZZ16006) for hosting funds of Posidonia oceanica in a good state of conservation.
Cala del Racó is protected from the wind and waves due to the geographical protection of the adjacent mountains (Oltá, Bernia and Mascarat). However, on the south face it is more exposed to waves. The substrate of the study area is composed of rocks or boulders with a high presence of photophilic algae that, with the gradient of depth, gives way to different biocenoses of fine sand and Posidonia oceanica meadows. On this side, the P. oceanica meadows extend from 6 to 22 m deep (Abbiati et al., 2017) (Fig. 1).
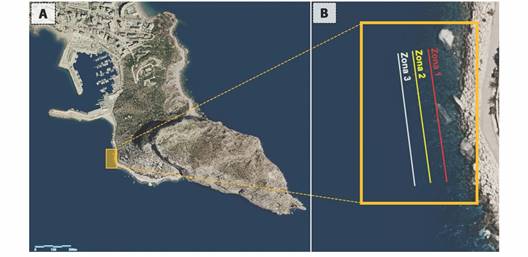
Fig. 1 A) Map of the study area located in Cala del Racó (Calpe), west of the Natural Park of Peñón de Ifach. Coordinates: 38º 38’ 03’’ N & 0º 04’ 16’’ E. B) Exact location of the transects of monitoring carried out it the different sampling areas. Zone 1: 3.5 m deep, rocky surface. Zone 2: 5 m deep, interface between rocky and Posidonia oceanica meadow surfaces. Zone 3: 7 m deep, P. oceanica meadow surface (GVA Viewer, 2022).
Experimental design: All the dives were carried out with the logistical support of the marine station of the Instituto de Investigación en Medio Ambiente y Ciencia Marina (IMEDMAR-UCV). Sampling began in May 2021 and finished in May 2022. They were distributed based on the results of the gonadal maturation of Villamor & Becerro (2010) and the information obtained during the registration of the spawning of E. sepositus gametes that occurred in 2018 in Cala del Racó. Since the aforementioned gamete spawning lasted for at least two weeks and was recorded overnight, the dives in this study were conducted during the night with variable frequencies depending on the period of the year: weekly during the period with the highest probability of spawning (October 2021), biweekly during the periods of moderate probability of spawning (between May and October 2021 and between November and December 2021), and monthly in periods of lesser spawning probability (from January to May 2022). The samplings were made to coincide with the full moon and the new moon whenever the weather conditions allowed it, to know if gametogenesis in E. sepositus followed a lunar pattern, as has been detected in captivity in other starfish such as Protoreaster nodosus (Scheibling & Metaxas, 2008). However, spawning records have not been analyzed because this phenomenon has not been detected during this study.
For data collection and characterization of the E. sepositus population, all samplings were carried out with the help of autonomous diving equipment. Three fixed areas were established for the entire studio. Zone 1 was found at an average depth of 3.5 m and is made up of a mainly rocky environment, with a large cover of arborescent photophilic algae and crusty coralline algae, although small patches of P. oceanica and sand were also found. Zone 2 had an average depth of 5 m and included the interface between the rocky surface and the beginning of the meadow of P. oceanica, for which reason substrates both common to Zone 1 and Zone 3 occurred. Zoe 3 was located at an average depth of 7 m and was made up mostly of P. oceanica meadow and sandy bottoms, although some scattered rocky surfaces were also found. The zones were separated by a limited depth gradient of 3 to 7 m. However, the environment observed at each depth changed rapidly. In each of the 3 zones mentioned above, a line transect of 50 x 2 m was established and the presence of the starfish E. sepositus was noted. Therefore, a 100 m2 surface was sampled in each transect. For each starfish observed, the depth, the corresponding area (1, 2 or 3), the distance of the transect at which it was located and the substrate (naked rock, arborescent photophilic algae (AF), crusted coralline algae (CCA), sand, sponges, P. oceanica leaves or P. oceanica rhizomes) were recorded. In case of observing a starfish on more than one of these substrates at the same time, we would consider the substrate as “mixed substrate. The length of the arm opposite to the madreporite (LB) was also recorded, that is, the distance from the end of the arm to the beginning of the central area. The reason for measuring LB is that Bodí Broseta (2019) showed with R2 = 0.78 that this is the best variable to estimate the total length (i.e. the distance between the end of the arm and the end of the opposite arm) (TL) of E. sepositus individuals.
A database was built with Microsoft Excel (2016) with all the data collected from each observed individual. A data matrix was developed and the E. sepositus density (individuals/m2) was determined for each of the surveys and zones. In this way, descriptive graphs showing the changes in the density of starfishes for the entire year, in each of the zones, were generated. Besides, a one-way analysis of variance (ANOVA) was used to determine if there were significant differences between the densities of E. sepositus recorded in each of the zones. Water temperature was also recorded twice a month in vertical profiles of up to 15 m depth, using the oceanographic probe AAQ-RINKO 177 (LFE Advantech CO., Ltd.) to relate the seasonal temperature changes with the population changes of E. sepositus. Subsequently, the Ocean Data View (ODV) program was used to graphically represent the temperature profiles taken throughout the study year.
The size distribution of the starfishes was also analyzed by zone (measured in LB). Since LB measures were continuous quantitative data, LB measurements were classified into the following 6 categories: “< 3 cm”, “3-4 cm”, “4-5 cm”, “5-6 cm”, “6-7 cm” and “>7 cm”. The program IBM SPSS Statistics was used to perform a Pearson Chi-square analysis to see if there was a statistically significant dependency between the zone and the size of the starfishes. For this work, the sizes were grouped into 3 categories: SMALL if its LB is less than 4 cm, MEDIUM if its LB is between 4 and 6 cm, and BIG if its LB is greater than 6 cm. On the other hand, another Pearson Chi-square analysis was performed to see if there was a statistically significant dependency between the LB and the substrate in which the individuals were found. For this analysis, the same 3 categories as in the previous Chi-square were used: SMALL, MEDIUM, BIG. Subsequently, the program R-Project 4.1.3 (R Core Team, 2022) and the “readxl” package were used to graphically represent the size distribution (SMALL, MEDIUM, BIG) in each of the substrates using a barplot.
Results
After 19 days of sampling, a total of 488 individuals of E. sepositus were recorded, with an average number of 25.7 starfish per sample with a standard deviation of 11.2.
Echinaster sepositus density in each of the sampled zones: ANOVA showed significant differences in density in each of the zones (F = 4.01, P = 0.002). In Zone 1, 120 individuals were found (24.6 % of the total) with an average density of 0.06 individuals/m2. Such density increased irregularly from spring (time with a lower density in Zone 1) to winter, when the density reached twice the maximum value recorded in this area (0.14 ind/m2). There was also another peak density in summer (0.1-0.11 ind/m2), although not as marked as the one that appeared from December to March. The lowest densities were recorded between spring and summer, with zero individuals recorded on one occasion.
In Zone 2, 218 individuals were found (44.7 % of the total encounters) and an average density of 0.11 ind/m2. In this case, the maximum density was observed in spring (0.24 ind/m2). This density was reduced in summer and increased again between October and November, where the second highest density peak occurred (0.17-0.19 ind/m2). The lowest recorded starfish density (0.02 ind/m2) was observed in May.
In Zone 3, 150 individuals were found (30.7 % of the total encounters), with an average density of 0.08 ind/m2. The individual density remained between 0.01 and 0.06 from May to August. From September, the density increased progressively until October, when it reached its maximum (0.24 ind/m2). After this peak, the density remained lower between 0.11 and 0.15 ind/m2 and then it reached a second peak in January (0.21 ind/m2). In the following months, the density declined until it equaled the low densities found in spring.
The sampling day with the highest number of starfish found was the 21st of October of 2021, in autumn. Up to 51 individuals were recorded in one transect (0.51 ind/m2). On the contrary, on May 10th, 2022, in spring, the lowest E. sepositus density in a sample was recorded (0.09 ind/m2) (Fig. 2).
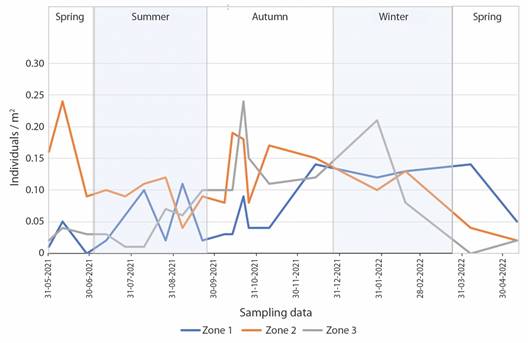
Fig. 2 Echinaster sepositus density (ind/m2) in the different sampling zones during the study. Zone 1: 3.5 m deep, rocky surface. Zone 2: 5 m deep, interface between rocky and Posidonia oceanica meadow surfaces. Zone 3: 7 m deep, P. oceanica meadow surface.
The water temperature showed the typical seasonal variations of the Western Mediterranean Sea, with a minimum temperature of 14.13 ºC in March 2022 and a maximum of 27.17 ºC in August 2021. As usual in these waters, the summer period presented a seasonal thermocline, while the winter months presented homogeneity of temperature in the water column. Between mid-November 2021 and January 2022 and in July 2022, there was missing data due to malfunction of the oceanographic probe (Fig. 3).
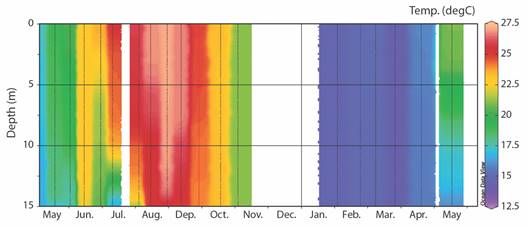
Fig. 3 Oceanographic profile of the station at a depth of 15 m of temperature values during the sampling period in Calpe. Between mid-November 2021 and January 2022 and in July 2022, there was missing data due to malfunction of the oceanographic probe.
Percentage of appearance of Echinaster sepositus in the different types of substrates: Of the 120 individuals studied in Zone 1, 68 individuals (59.6 %) were found on AF. Subsequently, 29 specimens (24.17 %) were found on CCA, 11 starfish (9.17 %) on mixed substrate, 6 (5 %) on rhizomes of Posidonia plants and 4 individuals (3.3 %) on bare rock. Finally, the substrates in which a smaller number of starfish were found were Posidonia leaves and sponges, with only one specimen on each (< 1 %). No individual was found on the bare sand. Of the 218 sightings of E. sepositus in Zone 2, 120 (55 %) occurred on AF, 39 encounters (17.9 %) were observed on the rhizome of P. oceanica, 21 (9.6 %) on CCA, 16 (7.3 %) on mixed substrate, 10 (4.6 %) on bare rock and 8 (3.67 %) on the leaves of P. oceanica. Finally, there were 3 records of starfish (1.38 %) on sandy bottom and a specimen was sighted on a sponge (0.46 %). In Zone 3, 150 individuals of E. sepositus were recorded. Of the total of sightings, 110 (73.3 %) occurred on the rhizomes of P. oceanica, 23 records (15.3 %) occurred on Posidonia leaves, 7 starfish (4.67 %) were observed on the sandy bottom and 5 individuals (3.3 %) appeared on a substratum occupied by AF. Finally, in the CCA and mixed substrates, 3 and 2 sightings were recorded respectively, which represents 2 and 1 % of the sightings. No specimen of E. sepositus has been recorded on sponges or on bare rocks. The percentage of appearance of E. sepositus in the different types of substrates is shown in Table 1.
Table 1 Percentage of appearance of E. sepositus in the different types of substrates in each of the zones.
AF | CCA | Rock | P. leaves | P. rhizomes | Sand | Sponge | Mix | |
Zone 1 | 56.67 | 24.17 | 3.33 | 0.83 | 5.00 | 0.00 | 0.83 | 9.17 |
Zone 2 | 55.05 | 9.63 | 4.59 | 3.67 | 17.89 | 1.38 | 0.46 | 7.34 |
Zone 3 | 3.33 | 2.00 | 0.00 | 15.33 | 73.33 | 4.67 | 0.00 | 1.33 |
AF: arborescent photophilic algae; CCA: crusted coralline algae; P. leaves: leaves of Posidonia oceanica; P. rhizomes: rhizomes of P. oceanica. Zone 1: 3.5 m deep, rocky surface. Zone 2: 5 m deep, interface between rocky and P.oceanica meadow surfaces. Zone 3: 7 m deep, P.oceanica meadow surface.
Size distribution of Echinaster sepositus in the sampling areas: The starfish studied in Zone 1 had an average LB of 5.8 cm, a maximum LB of 8.8 cm and a minimum LB of 2.9 cm, with a peak of individuals between 5 and 6 cm. In Zone 2, an average size of 5.3 cm, a maximum size of 9.4 cm and a minimum of 3.4 cm were recorded, with a predominant size distribution between 4 and 6 cm in this zone. Individuals which were in Zone 3 have an average LB of 4.34 cm, a maximum LB of 6.2 cm and a minimum LB of 2.6 cm and a very noticeable peak of specimens between 4 and 5 cm (Fig. 4).
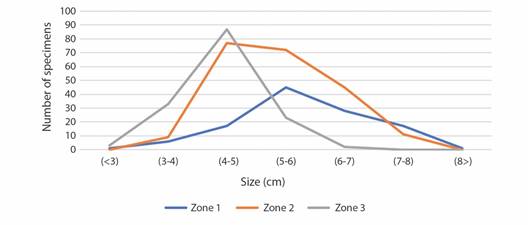
Fig. 4 Distribution of the sizes (cm) of the number of individuals of Echinaster sepositus registered in each of the sampling zones. Zone 1: 3.5 m deep, rocky surface. Zone 2: 5 m deep, interface between rocky and Posidonia oceanica meadow surfaces. Zone 3: 7 m deep, P.oceanica meadow surface.
The Pearson Chi-square analysis showed that there was a statistically significant relationship between the different zones and the different sizes grouped into categories: SMALL, MEDIUM, BIG, with 95 % confidence. So the distribution of the different sizes of starfish in each zone was not uniform and they had size segregation per zone (P < 0.001) (Table 2). While the small starfish were located mostly in Zone 3, most of the largest animals were found in Zones 1 and 2. Similarly, another Chi-square analysis established, with 95% confidence, that there was a statistically significant relationship between the different substrates and the starfish sizes (again grouped into SMALL, MEDIUM, BIG) (P < 0.001) (Table 3). The individuals of medium to large sizes were located preferentially on substrates covered by AF and CCA. On the other hand, medium to small starfish were found preferentially in the rhizomes or the leaves of P. oceanica (Fig. 5).
Table 2 Result of Pearson’s Chi-Square analysis between the different substrates and the sizes of Echinaster sepositus grouped into categories.
Crosstab between Zone*LB (category) | |||||||
---|---|---|---|---|---|---|---|
BIG | MEDIUM | SMALL | Total | ||||
Study areas | Zone 1 | 52 | 64 | 4 | 120 | ||
Zone 2 | 58 | 150 | 10 | 218 | |||
Zone 3 | 3 | 111 | 36 | 150 | |||
Total | 113 | 325 | 50 | 488 | |||
Pearson’s Chi-Square Test | |||||||
Value | gl | Asymptotic significance (bilateral) | |||||
Pearson’s Chi-Square Test | 95.881 | 4 | <.001 | ||||
Likelihood ratio | 105.572 | 4 | <.001 | ||||
N of valid cases | 488 |
Zone 1: 3.5 m deep, rocky surface. Zone 2: 5 m deep, interface between rocky and P.oceanica meadow surfaces. Zone 3: 7 m deep, P.oceanica meadow surface. gl: degrees of freedom. LB: length of the arm.
Table 3 Result of Pearson’s Chi-Square analysis between the different study areas and the sizes of Echinaster sepositus grouped into categories.
Croostab between Substrate*LB (category) | |||||
---|---|---|---|---|---|
BIG | MEDIUM | SMALL | Total | ||
Substrate | AF | 64 | 121 | 4 | 189 |
Sand | 0 | 8 | 2 | 10 | |
CCA | 22 | 26 | 4 | 52 | |
Sponge | 1 | 1 | 0 | 2 | |
Mix | 9 | 21 | 1 | 31 | |
P. leaves | 1 | 27 | 5 | 33 | |
P. rhizome | 8 | 112 | 34 | 154 | |
Rock | 8 | 9 | 0 | 17 | |
Total | 113 | 325 | 50 | 488 | |
Pearson’s Chi-Square Test | |||||
Value | gl | Asymptotic significance (bilateral) | |||
Pearson’s Chi-Square Test | 96.312 | 14 | <.001 | ||
Likelihood ratio | 111.036 | 14 | <.001 | ||
N of valid cases | 488 |
gl: degrees of freedom. LB: length of the arm.
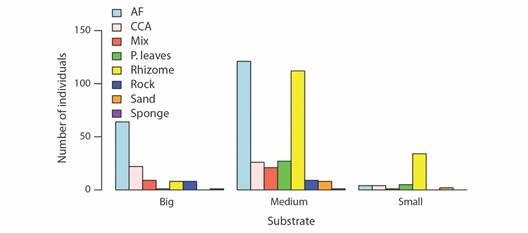
Fig. 5 Size distribution of Echinaster sepositus grouped into categories in each of the substrates considered in the study. (Small: LB lower than 4 cm. Medium: LB between 4 and 6 cm. Big: LB bigger than 6 cm).
Reproduction in Echinaster sepositus: After 19 samplings carried out during one year of research, there was no record of spawning of E. sepositus.
Discussion
Populations of the Mediterranean red starfish follow an aggregated distribution, so that they can be absent or be very abundant in locations close to each other. However, the factors determining this distribution remain unknown (Villamor & Becerro, 2010). A greater variability of habitats favors a greater settlement of species that can occupy the different niches, regulating different ecological parameters such as abundance and distribution of species (Hewitt et al., 2005; Zajac et al., 2003). The high density of individuals observed in Cala del Racó could be due to the diversity of habitats occurring in a relatively small area, including rocks, sandy bottoms and meadows of P. oceanica (Abbiati et al., 2017). Another characteristic of the study area that could help to explain the high density of individuals in Cala del Racó, is the protection to the North and East winds offered by the Peñón de Ifach and the West winds from the Morro the Toix outcrop. An earlier study by Villamor & Becerro (2010) indicated a change in the communities at a depth of 5 m produced by the hydrodynamics, which makes the presence of E. sepositus accidental. However, in our study area, there have been 120 records of starfish in Zone 1, whose average depth was 3.5 m.
In Zone 1, located at a shallower depth, there was an increase in the density of starfishes from December to March, when the temperature of the water drops to 14 ºC, and there was a decrease in density in spring and summer, when the water temperature reaches 27 ºC. In Zone 3, on the contrary, the density of individuals begins to increase from the month of August to October-November and decreases again during winter and early spring, except for records taken in the sampling carried out in January. These data suggest that the population of E. sepositus would be moving, from deeper and colder areas in summer, to shallower and warmer areas in winter. Changes in the behavior of starfish due to temperature have already been studied. A study carried out with Astropecten irregularis (Pennant, 1777) concluded that this species exhibited a seasonal pattern of abundance, with maximum densities in summer and minimum densities during the winter (Freeman et al., 2001). Similar results already described in A. irregularis by Christensen (1970), recorded a decrease in population density in the coldest months. This author contemplated the idea that these starfish could even “hibernate” during certain months of the year. The starfish Asterias rubens Linnaeus, 1758 also shows a greater density in the warm months and less in the cold months. However, in A. rubens, its variation is due to the seasonality of its main food, mussels (Gallagher et al., 2008). In the same way, it is possible that the movement observed in E. sepositus at different depths is due, not only to the temperature itself, but also to the seasonal availability of food.
In Zone 1, most of the starfishes were located on AF (56.7 %) and on CCA (24.17 %). In Zone 2, the substrates with the highest number of E. sepositus specimens were AF (55.1 %) and the rhizomes of P. oceanica with 17.9 % of the records. In Zone 3, 73.3% of the starfishes were located between the rhizomes of P. oceanica and 15.3 % on the leaves. The appearance of starfishes in sand, bare rock or sponges was much lower. This study coincides with previous ones that mention the presence of E. sepositus in seagrass, sand, rock, pebbles and crusted coralline algae (Bacallado et al., 2020; Caballero et al., 2000; Clark & Downey, 1992; Entrambasaguas et al., 2008; Villamor & Becerro, 2010). A study conducted on the Catalan coast concluded that the abundance of E. sepositus was only correlated with the percentage cover of crusted coralline algae (Villamor & Becerro, 2010). However, in Cala del Racó only 10.6 % of the sightings occurred over coralline algae, well behind sightings over AF and rhizomes of P. oceanica, 38.7 % and 31.5 %, respectively. As for the presence of red starfish on rocky bottoms, our data showed a greater similarity with the observations of E. sepositus made on the coast of Corsica by Raisch (2018), where E. sepositus appeared in greater abundance in the tussock and shrub algae.
If we focus on the relationship between the size of the starfishes and the area in which they were found, we observed a clear predisposition of medium and large individuals in Zone 1 and Zone 2, which were at an average depth of 3.5 to 5 m respectively. Nevertheless, the smallest specimens were located preferably in Zone 3, which was at an average depth of 7 m. A clear preference of medium and large individuals was also observed by substrates covered by arborescent photophilic algae and by crusted coralline algae. Instead, the smallest starfishes tend to be in the Posidonia meadow, both in the leaves and in the rhizome. One of the reasons why this phenomenon happens could be a change in nutritional requirements of E. sepositus at different stages of growth. At the same time, it could be due to a hydrodynamic reason. Hydrodynamic plays a central role in the ecology of environment shorelines influencing survival, morphology, movement, feeding and reproduction (Denny, 2006). Adverse hydrodynamic conditions can produce changes in the behavior of some echinoderms (McEuen, 1988). The Zone 1, where AF and CCA substrates abound, was the shallower and more exposed environment to currents. Smaller starfishes might have a harder time withstanding these currents while feeding on the algae that grow on the rocks.
The Posidonia meadow, together with a greater depth, would offer a calmer environment for smaller starfish. Species of the genus Echinaster feed on microscopic organisms and detrital materials by internal digestion or may consume tissues partially digested from larger organisms by external digestion (Turner, 2013). The observation of a starfish with its stomach everted indicates that it is feeding at the time (Lawrence, 2013). Observations made in aquariums indicate that E. sepositus feeds on different groups of invertebrates, microbial films and carrion (Brooks & Gwaltney, 1993; Ferguson, 1984; Jangoux & Lawrence, 1982; Scheibling & Lawrence, 1982; Sloan, 1980). They have also been found with the stomach evaginated on multiple invertebrates, algae and sediments (Ferguson, 1969). However, no study has shown what E. sepositus eats in the wild (Turner, 2013). Some authors considered sponges as a key food for E. sepositus (Sarà & Vacelet, 1973; Vasserot, 1961). However, subsequent studies have not found a consistent trophic relationship between the Mediterranean red starfish and the sponges (Maldonado & Uriz, 1998). The presence of E. sepositus on sponges has been anecdotal during the sampling year in Cala del Racó, unlike 33 % of appearance on sponges of E. gramicola in an investigation by Ferguson (1969). This could support the results obtained by Maldonado & Uriz (1998). It is necessary to carry out specialized studies on the feeding of E. sepositus since there are many knowledge gaps to be resolved (Turner, 2013). Despite the feeding of E. sepositus was not the main objective of the present work, the results obtained in the location of the starfishes in the different substrates, and the differentiation of size in each one of the substrates, provide useful information to improve the development of future research.
Regarding the reproductive cycle, there is a great variety of strategies in asteroids regarding reproduction, which can be seasonal or continuous (Mariante et al., 2010). Seasonal reproduction has been demonstrated in some species of the genus Echinaster in the Atlantic Ocean and the Gulf of Mexico (Chen & Chen, 1992; Ferguson, 1975; Guzmán & Guevara, 2002; Scheibling & Lawrence, 1982; Turner, 2013). The main advantage of this kind of reproduction is the synchronization of gametogenesis in males and females, which makes fertilization more likely (Bos et al., 2008; Carvalho & Ventura, 2002; Raymond et al., 2007). After 19 samplings carried out during a year in Cala del Racó, no specimen of E. sepositus has been observed reproducing. For the spawning to occur in the time intervals between the samplings, it should have happened in a short time window of a few days in summer and autumn, but no indications of it were recorded. It is more probable to suppose that the laying of gametes in E. sepositus did not happen that year because it does not occur annually. The reproduction in the subgenus Echinaster (Echinaster) could be dependent on environmental conditions such as temperature, hydrodynamics or the amount of available food, which would determine the starfish of spawning; as it happens with the subgenus of Echinaster (Otilia) (Mariante et al., 2010; Scheibling & Lawrence, 1982). The importance of temperature in gametogenesis has also been mentioned for Marthasterias glacialis, a species of starfish that inhabits from the sublittoral to depths of 180 m and that lays gametes in surface waters, where temperature increase is maximum in spring-summer (Benítez-Villalobos et al., 2006; Mercier & Hamel, 2009; Minchin, 1987).
Despite reproduction not being observed, the highest density of individuals (0.51 ind/m2) occurred in October, far exceeding the densities recorded in the samplings directly before and after. This date coincides with the time of the year in which the reproduction of E. sepositus was recorded in this same area in 2018. This timing also seems to adjust to the maximum gonadal development registered for the months of June and July by Villamor & Becerro (2010). During breeding events, asteroids congregate to facilitate external fertilization after spawning (Hancock, 1958). To have occurred in the same time intervals between sampling, spawning would have had to take place within a short window of a few days in summer and autumn. However, no evidence of this was recorded. Another possibility is that gamete spawning in E. sepositus did not occur that year, possibly due to some water conditions such as temperature, hydrodynamics or the amount of available food (Mariante et al., 2010). The conditions of the water could also be the ones that explained the time difference between the different records of reproduction in E. sepositus. For future research, we recommend repeating the sampling intensity, and concentrating the effort in the months of September and October, when data indicate that the probability of observing spawning is higher.
Ethical statement: the authors declare that they all agree with this publication and made significant contributions; that there is no conflict of interest of any kind; and that we followed all pertinent ethical and legal procedures and requirements. All financial sources are fully and clearly stated in the acknowledgments section. A signed document has been filed in the journal archives.