Introduction
The banana passionfruit plants (Passiflora tripartita var. mollissima) produce fruits with high export potential because of its organoleptic and nutritional properties, and high content of bioactive agents, antioxidants, phenolic compounds, carotenoids and fiber (Fischer et al., 2020; Flechas-Bejarano et al., 2019). It is a species in the Tacsonia subgenus in the Passifloraceae family, native to the Andean region from Bolivia to Venezuela, that grows in tropical and subtropical areas (Esquerre-Ibañez et al., 2014; Primot et al., 2005). In 2022, Colombia experienced a 25 % surge in banana passionfruit exports to European countries, making it the fourth most exported passion fruit. Nationally, the supply reached 14 365 tons, with Boyacá contributing a significant 78.39 % (DANE, 2023).
In general, the cultivation of other passionfruit species has been increasing; however, due to changes in climatic conditions, such as high temperatures and low precipitation, crop productivity is not satisfactory (Fischer et al., 2009; Gomes et al., 2012). A decrease in water availability produces a water deficit in plants, altering growth, development, and production.
Drought resistance mechanisms in plants can be classified as escape, avoidance, tolerance and recovery (dos Santos et al., 2022). They may have escape responses, completing their life cycle before the dry period begins when phenological development successfully fits with the availability of moisture in the soil either by means of plasticity in development or rapid phenological development (Farooq et al., 2009). They may have avoidance mechanisms, in which plants reduce the impact of drought through morphophysiological changes that minimize water loss, including stomatal control and root characteristics such as biomass, length, density and depth, among others (dos Santos et al., 2022; Farooq et al., 2009; Turner et al., 1996). They may exhibit drought tolerance mechanisms when plants could grow, reproduce, and provide an economic crop yield with a poor water supply by means of adaptive characteristics such as accumulation of compatible solutes, increased response of the antioxidant system and maintenance of water potential (dos Santos et al., 2022; Farooq et al., 2009; Turner et al., 1996).
Water deficits alter different processes, and plant susceptibility depends on variables such as genotype, state of development, duration, and intensity of stress. The latter may vary depending on soil, climatic and agronomic factors (Anjum et al., 2011). The effects of a water deficit on cultivated species have been studied by several authors, where changes in growth and development were observed (Anjum et al., 2017), along with anatomical (Bacelar et al., 2003; Choat et al., 2003), and physiological responses that mainly affected stomatal conductance, CO2 fixation, photosynthetic rate, chlorophyll concentration and water status (Basu et al., 2016; Ben Abdallah et al., 2018; Boughalleb et al., 2014; de Freitas et al., 2017).
Although some studies have been carried out on Passiflora tripartita var. mollissima for responses of plant growth under different conditions, for fruit quality and for post-harvest treatments (Fischer & Miranda, 2021; Flechas-Bejarano et al., 2019; Mayorga et al., 2020; Rodríguez et al., 2019a; Téllez et al., 2011), the effects of a water deficit on anatomy and physiology are not known. It has been reported that, under a water deficit, other Passiflora species experience a reduction in growth, dry biomass, and root vitality (Qi et al., 2023); a decrease in physiological parameters such as leaf area, stomatal conductance (Lozano-Montaña et al., 2021), and a diminution in some chlorophyll fluorescence parameters (Gomes et al., 2012). Additionally, changes due to water deficit at the anatomical level have been demonstrated in tissues of the leaf blade (Gutiérrez et al., 2009; Souza et al., 2018) and root (Lima et al., 2019), and at the molecular level with gene families involve in drought stress (Rizwan et al., 2022; Yang et al., 2022).
Since a water deficit is one of the greater constraints limiting agriculture productivity and profitability worldwide (Farooq et al., 2009), information on the strategies used by plants in response to water availability is essential to develop crops that are more tolerant to droughts and have good agricultural production. The objective of this study was to analyze some physiological and anatomical responses of banana passionfruit plants to water deficit.
Materials and methods
Growing conditions and biological material: This experiment was conducted under greenhouse conditions (4°38’08’’ N & 74°04’58’’ W, at 2 640 m.a.s.l.) at the Departamento de Biología of the Universidad Nacional de Colombia, Bogotá. Data on environmental conditions during the experiment were collected with a weather station (EM50 Data Logger Decagon Devices Inc., Washington, USA). An average ambient relative humidity of 74.04 %, air temperature of 16.67 ºC, photosynthetically active radiation of 169.04 μmol photons m-² and photoperiod of 12 light h:12 h of darkness were recorded.
Three-month-old Passiflora tripartita var. mollissima plants, from Sabaneta village, municipality of La Vega, in the department of Cundinamarca, with 11 leaves and 6 tendrils, were transplanted into pots (20x15 cm), with a soil substrate (loamy texture: 30 % sand, 52 % silt, 18 % clay; pH 5.6) and rice husks at a 3:1 ratio. The seedlings were acclimatized for 1 month, keeping them under optimal conditions for health, water and nutrients, after which two irrigation treatments were carried out: (1) Irrigated treatment at field capacity (Control treatment) and (2) treatment for water deficit at 70 % water reduction (Water deficit treatment). The requirements to reach field capacity for the substrate were gravimetrically calculated to determine the percentage of water used, along with the amount of water to be added to the 70 % reduction treatment (water deficit). The respective irrigation to maintain the water conditions of each treatment was carried out every third day. The experiment was monitored for 50 days.
Growth: The height of the stem was measured weekly from the base to the last node, and the number of leaves and leaf area were determined. The measurements were taken on days 0, 7, 14, 21, 28, 35, 42 and 49 after the start of treatment-dat. The leaf area index (LAI) was estimated as proposed by Gardner et al. (2013). At the end of the experiment, the dry weight of the shoot (stem plus leaves) and roots was determined after drying the plants at 60 °C for 72 h (49 dat). Subsequently, the root: shoot ratio (R/S) was determined.
Water status: The relative water content (RWC) was determined with the methodology described by Smart and Bingham (1974) with foliar discs. This parameter was determined at 49 dat with leaves from the middle-third of three plants per treatment.
Stomatal conductance: This parameter was determined with a porometer (Decagon Devices, Inc., Washington, USA). Three leaves from the middle-third of each plant were measured in seven plants for each treatment. This measurement was taken from 08:30-10:00 AM, selected with daily curves, on 0, 7, 14, 21, 28, 35, 42 and 49 dat. The data are shown as the average of seven plants per treatment along with the standard error.
Photochemical efficiency of PSII (Fv/Fm): The Fv/Fm was measured with a non-modulated fluorometer (Pocket Pea-Hansatech, UK). This measurement was taken at 5:00 AM in 7 plants per treatment using one leaf per plant, which were previously adapted to darkness for 20 minutes on days 0, 7, 14, 21, 28, 35, 42 and 49 dat. The data are shown as the average of seven plants per treatment along with the standard error.
Chlorophyll content: Leaves with different green color intensities were selected, and this parameter was estimated in SPAD units with a Minolta SPAD 502 (Konica Minolta Sensig, Inc., Sakai, Osaka, Japan). Leaf discs were extracted from the exact same location as the SPAD measurement. The leaf discs were immediately ground, and the chlorophyll was extracted with acetone (Lichtenthaler 1987; Melgarejo et al., 2010; Rodríguez et al., 2019b;). A calibration curve was constructed to relate the chlorophyll content of different coloring shades leaves to cover the entire color spectrum and SPAD units (Lozano-Montaña et al., 2021; Parry et al., 2014). During the experiment, the chlorophyll content was estimated with the SPAD using leaves from the middle-third in three leaves from 7 plants per treatment each week (0, 7, 14, 21, 28, 35, 42 and 49 dat). This measurement was taken on the same leaf where stomatal conductance and Fv/Fm were measured, and it was calculated as the average of three readings. The SPAD data were interpolated in the calibration curve to calculate the chlorophyll content.
Anatomical morphometrics: At the end of the experiment (49 dat), three leaves were collected from the middle-third of plants in each treatment. The leaves were fixed in FAA (formaldehyde: acetic acid: 70 % ethanol, 10:5:85), stored in 70 % ethanol, and treated following the protocol of Toro-Tobón et al. (2022). This involved standard methods of dehydration using a clearing agent (Histoclear), paraffin infiltration, sectioning with a rotary microtome (820 Spencer, American Optical Company, New York, USA), and attachment to microscope slides. The slides were stained with astra-blue and basic fuchsin and deposited. They were analyzed and photographed using a microscope Olympus BX50 with a Moticam Pro 282B camera (Olympus Optical Co., Ltd, Tokyo, Japan), in the optical equipment laboratory of the Departamento de Biología, Universidad Nacional de Colombia. Digital images were processed and edited with ImageJ (Schneider et al., 2012).
The images were used to take measurements in leaf cross-sections for the thickness of the upper and lower epidermis, spongy and palisade parenchyma, cuticle, outer diameter of the central rib and xylem. In addition, the internal diameter of xylem vessels and the number of vessels were measured. The measurements were taken ImageJ (Schneider et al., 2012). 3 leaves per treatment were taken, and 10 cuts were taken from each leaf for the variables (N = 30).
Stomatal density: An epidermal impression was made by coating the leaf surface with nail varnish using 4 leaves for each treatment (Melgarejo et al., 2010). The surface morphology/anatomical characteristics were studied using a light microscope, Olympus BX50 with a Moticam Pro 282B camera (Olympus Optical Co., Ltd, Tokyo, Japan), and the stomatal density was calculated with 5 optic fields at a 400 magnification.
Experiment design and data analysis: Each treatment had a total of 20 plants, of which 7 were used to measure physiological variables (non-destructive methods) during the experiment, and another 7 were used for biomass measurements (destructive methods) carried out 49 dat. Water status assessed in leaf discs (3 plants per treatment), and anatomical parameters were determined using leaves (3 plants per treatment), both at the experiment’s end. The experiment was replicated once under identical conditions and with the same number of plants, yielding similar results.
For the statistical analysis, the measured variables of both treatments were compared over time using the Kruskal-Wallis test. When there were significant differences in this test, peer comparisons (‘t’ tests) were used. For the variables of the destructive methods, a single comparison was made with a student’s ‘t’ test. Finally, a Pearson correlation matrix was performed with all variables at the 49 dat. The significance level for all tests was α = 0.05. Figures were performed with Prism (GraphPad, 2023) and statistical tests were performed with R (R Core Team., 2021).
Results
Foliar physiology and biochemistry: A lower plant height was observed with the water deficit than in the control plants starting at day 35 after treatments began (dat). The plants with the water deficit presented a height of less than 38.5 % at day 49 with respect to the control plants (Fig. 1A). Lower values for the number of leaves and leaf area were also evidenced from day 35 for the water deficit treatment (Fig. 1B, Fig. 1C).
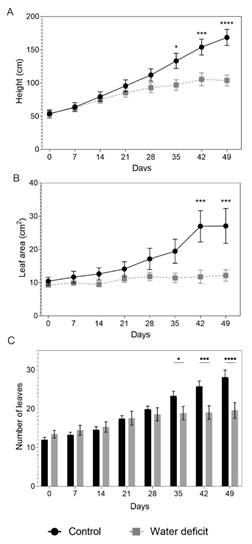
Fig. 1 Growth variables of Passiflora tripartita var. mollissima plants under water deficit for 49 days. A. Plant height, B. leaf area and C. number of leaves. Vertical bars represent the standard error of the mean, N = 7. Asterisks indicate significant differences between treatments (control and water deficit), on each of the days (P ≤ 0.05).
For the accumulation of biomass, the control plants obtained a greater dry weight of shoot and roots than those subjected to the water deficit (Table 1). Significant differences were found between the treatments for the root: shoot ratio (R/S) and leaf area index (LAI). The plants with the water deficit conditions had a higher R/S ratio and a lower LAI than the control plants, with a difference of 42.2 % and 82.9 %, respectively (Table 1). The relative water content (RWC) in the water deficit plants decreased, with a significant difference at 49 dat (Table 1), with a reduction of 32.5 % as compared to the control plants.
Table 1 Relative water content (RWC), leaf area index (LAI), shoot dry weight (SDW), root dry weight (RDW) and root: shoot ratio (R/S), of Passiflora tripartita var. mollissima plants under two treatments (control and water deficit).
Variable | Control | Water Deficit | Test | P-value |
RWC | 96.684 | 65.295 | Kruskal−Wallis | 0.0495* |
LAI | 1.325 | 0.282 | Kruskal−Wallis | 0.001* |
SDW(g) | 6.130 | 3.090 | Kruskal−Wallis | 0.017* |
RDW (g) | 3.496 | 3.226 | t−Test | 0.723 |
R/S | 0.598 | 1.035 | t−Test | 0.015* |
* Asterisks indicate significant differences between treatments (P ≤ 0.05).
The maximum photochemical efficiency of PSII (Fv/Fm) had significant differences between the treatments only until 42 dat, with values close to (0.81), suggesting that the plants that were subjected to the water deficit did not exhibit photoinhibition of photosynthesis (Fig. 2A). The stomatal conductance was also lower in the plants subject to water deficit. This variable had a progressive decrease until day 49 dat, with a significant difference from the control from day 21 dat (Fig. 2B). No significant differences between treatments were found for the chlorophyll content although a decreasing trend was observed in the plants subject to the water deficit from 21 dat to 49 dat (Fig. 2C).
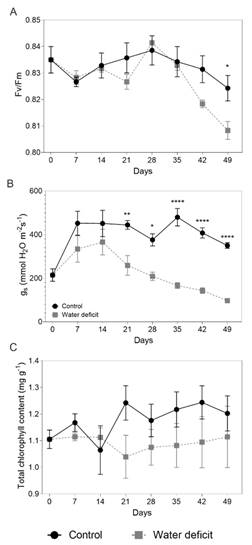
Fig. 2 Physiological and biochemical variables of Passiflora tripartita var. mollissima plants under water deficit for 49 days. A. Maximum photochemical efficiency of PSII Fv/Fm, B. stomatal conductance and C. total chlorophyll content. Vertical bars represent the standard error of the mean, N = 7. Asterisks indicate significant differences between treatments (control and water deficit), on each of the days (P ≤ 0.05).
Foliar anatomy: A dorsiventral mesophyll was evidenced in the cross section of the leaf blade in both treatments (Fig. 3A, Fig. 3B), with a uniseriate epidermis and a single layer of palisade parenchyma. The plants subjected to the water deficit had spongy parenchyma cells that were closer together (Fig. 3B) than in the plants without water deficit (Fig. 3A). The evaluation of the stomatal imprint on both sides of the leaves showed that banana passionfruit plants have hypostomatic leaves with anisocytic (three cells with a similar size enclosing the guard cells) and anomocytic stomata (five or more cells enclosing the guard cells) (Fig. 3E, Fig. 3F). The epidermal cells had sinuous anticlinal walls in both the control plants and plants subject to the water deficit (Fig. 3A, Fig. 3B).
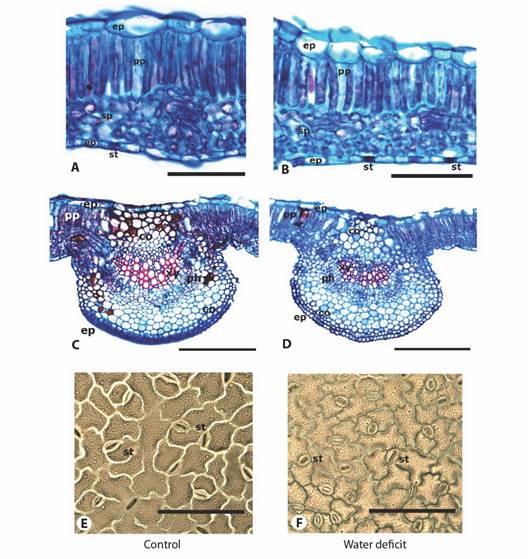
Fig. 3 A. C. and E. Anatomy of the Passiflora tripartita var. mollissima leaves under control, and B. D. and F. water deficit. A. B. C. and D. optical microscopy, sections stained with basic fuchsin and astra blue. A. and B. foliar blade, C. and D. midrib, and E. and F. abaxial side. (co: collenchyma; ep: epidermis; ph: phloem; pp: palisade parenchyma; sp: spongy parenchyma; st: stomata; xy: xylem). Scale bars (A. B. E. and F.) = 100 µm; (C. and D.) = 300 µm.
In the anatomical variables of the lamina and the leaf midrib (Fig. 3B, Fig. 3C, Table 2), significant differences were found (P ≤ 0.05) between the control plants and those subjected to the water deficit. A decrease in the thickness of the mesophyll and its tissues was observed in the plants subject to water the deficit, except for the lower epidermis. Similar results were seen for the variables outer diameter of the median rib, outer diameter of the xylem, proportion of both outer diameters, and the number and internal diameter of the vessels, which were lower in the plants with the water deficit (Table 2). However, the stomatal density in the plants with the water deficit was higher (Table 2).
Table 2 Anatomical variables measured in Passiflora tripartita var. mollissima plants under two irrigation treatments: control and water deficit.
Anatomical Characteristics | Control | Water Deficit | Test | P-value |
Mesophyll (μm) | 143.20 ± 7.04 | 122.6 ± 6.36 | t-test | < 0.01 * |
Upper Epidermis (μm) | 19.90 ± 3.27 | 17.6 ± 2,97 | t-test | 0.01 * |
Lower Epidermis (μm) | 8.71 ± 8.71 | 8.9 ± 8.90 | t-test | 0.66 |
Palisade Parenchyma (μm) | 65.42 ± 5.52 | 52.5 ± 3.83 | t-test | < 0.01 * |
Spongy Parenchyma (μm) | 48.36 ± 6.40 | 43.7 ± 4,34 | t-test | < 0.01 * |
Average Outer Midrib Diameter (μm) | 475.7 ± 38.4 | 399.3 ± 9.1 | Mann-Withney | < 0.01 * |
Outer Xylem Diameter (μm) | 93.8 ± 7.28 | 54.2 ± 3.37 | t-test | < 0.01 * |
Xylem Outer Diameter: Midrib outer diameter Ratio | 20.0 ± 0.769 | 13.6 ± 0.772 | t-test | < 0.01 * |
Number of vessels | 44 ± 3 | 31 ± 1 | Mann-Withney | < 0.01 * |
Inner Diameter of vessels (μm) | 17.3 ± 1.3 | 11.8 ± 0.7 | t-test | < 0.01 * |
Stomatal Density (mm-2 stomata) | 674.0 ± 229.2 | 1003.0 ± 26.9 | t-test | < 0.01 * |
* Asterisks indicate significant differences between treatments (P ≤ 0.05).
As depicted in the correlation matrix (Fig. 4), positive correlations were observed between growth variables and certain anatomical parameters associated with vascular tissue. A negative correlation was identified between the root: shoot ratio and leaf area, number of leaves, stomatal conductance (gs), Fv/Fm, and certain tissue measurements (mesophyll, palisade parenchyma, outer xylem diameter, outer xylem diameter: outer midrib diameter ratio, number of vessels, and inner diameter of vessels). Additionally, a robust positive correlation was evident among xylem parameters (outer xylem diameter, outer xylem diameter: outer midrib diameter ratio, number of vessels, and inner diameter of vessels).
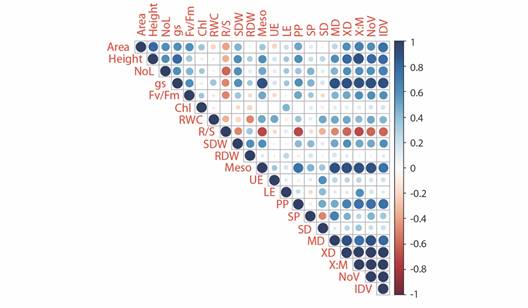
Fig. 4 Correlation matrix for physiological and anatomical parameters measure in banana passionfruit plants 49 after irrigation treatments began. NoL: number of leaves; gs: stomatal conductance; Fv/Fm: maximum quantum yield of PSII II; Chl: total chlorophyll content; RWC: relative water content; R/S: root: shoot ratio; SDW: shoot dry weight; RDW: root dry weight; Meso: mesophyll; UE: upper epidermis; LE: lower epidermis; PP: palisade parenchyma; SP: spongy parenchyma; SD: stomatal density; MD: outer midrib diameter; XD: outer xylem diameter; X:M: outer xylem diameter: outer midrib diameter ratio; NoV: number of vessels; IDV: inner diameter of vessels.
Discussion
Water deficit in banana passionfruit plants (Passiflora tripartita var. mollissima) results in a negative effect on growth, leaf number, leaf area, biomass and leaf area index. These same positive relationships between growth variables and stomatal conductance were evident in the correlation matrix. This decrease is attributed to the reduction of cell expansion and elongation because of a loss of turgor in the leaves, along with a low water potential and decrease in stomatal conductance (Jaleel et al., 2009), which are tolerance mechanisms to restrict water loss, decrease the area exposed to radiation, and, in turn, decrease the transpiration surface. In other species such as Passiflora edulis Sims f. edulis (Lozano-Montaña et al., 2021), P. alata, P. edulis, P. gibertii, P. setacea, and P. ccincinnata (Souza et al., 2018), similar results with changes in growth rate, gas exchange and foliar morphological characteristics under water deficit conditions have been reported.
The leaf area index (LAI) is a good indicator of plant growth since it is directly related to the capture of solar radiation and the production of dry biomass (Dalirie et al., 2010). As seen here, a water deficit decreases LAI and the accumulation of dry biomass (Table 1) since, when the leaf area is reduced, the photosynthetic efficiency is decreased, which causes a lower translocation of assimilates to the sink (Anjum et al., 2017). The increase in the R/S ratio in the plants with water deficit resulted from an increase in the root development, which is related to the translocation of photoassimilates to this organ as an avoidance strategy for water deficit to increase the intake of water by the plant (Jaleel et al., 2009). The correlation matrix indicated that as the R/S ratio increased, the growth variables and size of certain vascular tissues decreased (negative correlation). Similar results reported by Lozano-Montaña et al. (2021) for Passiflora edulis Sims f. edulis.
The results showed a decrease in the RWC of the plants subjected to the water deficit, a possible response to an osmotic adjustment to maintain a low turgor in the cells. Similar results were seen in Passiflora edulis Sims f. edulis (Lozano-Montaña et al., 2021), and in various crops of agricultural interest (Anjum et al., 2017). The gs values at day 42 (150 mmol H2O m-2 s-1) indicated that there was stomatal closure resulting from the effect of the water deficit, which meant a decrease in gas exchange (Liu et al., 2022). Stomatal closure is mediated by abscisic acid (ABA), and, with a water deficit, this growth regulator is produced quickly, which causes an increase in cytosolic calcium in the guard cells, causing the release of anions that leads to the depolarization of the membrane, so cells lose turgor, producing stomatal closure in the leaves (Liu et al., 2022).
No significant changes were observed in the variable Fv/Fm during the experiment. The values ranged between 0.81 and 0.83, which is typical in plants that do not present stress or damage at the level of the PSII, suggesting that banana passionfruit plants could have some protection mechanisms for photosystem II from water deficit (Maxwell & Johnson 2000; Murchie & Lawson 2013). The stability in the Fv/Fm values, from the day on which the water deficit treatment started until day 35, is consistent with the report by Gomes et al. (2012) for Passiflora edulis Sims, which suggests that the maximum photochemical efficiency of PSII will only decrease in banana passionfruit plants in the face of possible severe stress. Additionally, when evaluating the total chlorophyll content, no significant differences were observed between the treatments although there was a tendency to decrease from 21 dat, which suggests a possible relationship with the lower performance of the maximum potential quantum efficiency of photosystem II in the subsequent days (Fig. 2C, Fig. 2A, respectively). Similar results have been reported in P. edulis f. edulis (Lozano-Montaña et al., 2021). A slight reduction in total chlorophylls (Fig. 2C) could suggest that there was a low capacity for light harvesting and probably degradation or unmasking of the accessory pigments of the light harvesting antenna (Mafakheri et al., 2010).
Among the physiological variables, the most sensitive to water deficit was the gs, which controls stomatal opening, an immediate response to irrigation reductions. Similar results were reported by Lozano-Montaña et al. (2021) for P. edulis Sims f. edulis, by (Souza et al., 2018) for P. alata, P. edulis, P. gibertii, P. setacea, and P. cincinnata, and by Gomes et al. (2018) for P. edulis Sims f. flavicarpa. Banana passionfruit plants, when faced with a water deficit, close stomata to minimize water loss and, therefore, decrease the accumulation of aerial biomass.
The anatomical analysis showed a decrease in the leaf tissues in the banana passionfruit plants subjected to water deficit, resulting in a denser arrangement of cells (Fig. 3B, Fig 3D). The reduction in the thickness of the tissue (Table 2) implied a decrease in the diffusion of CO2 inside the leaves, a reduction of stomatal conductance, and a reduced rate of water transpiration to ensure an efficient use of water by the plants (Chartzoulakis et al., 2002). These changes in the leaf lamina tissue thickness can also affect CO2 conductance in the mesophyll (Cousins et al. 2020). Similar results were reported by Souza et al. (2018) for Passiflora spp. and by Chartzoulakis et al. (2002) for two avocado cultivars under water deficit stress.
In addition, increased stomatal density was observed in the banana passionfruit plants subjected to water deficit, which is a strategy to improve stomatal opening in less time, resulting in balanced gas exchange, better response under water scarcity conditions (Souza et al., 2018), and better transpiration control to minimize water loss (Ennajeh et al., 2010). Similar results for stomatal density changes resulting from water deficit have been reported in other species, such as olive trees (Ennajeh et al., 2010) and Astragalus gombiformis (Boughalleb et al., 2014).
Another strategy in the banana passionfruit plants for the water deficit was a decrease in the diameter of the xylem vessels (Table 2). This mechanism is positively correlated with the volume of water transported in plants and is inversely related to the vascular system (Inoue et al., 2019). Narrower vessels probably increase resistance to water flow, decrease the probability of cavitation in xylem vessels, and keep water columns under greater tension because they have a greater surface area in relation to the volume and a greater proportion of water molecules adhered to the wall (Boughalleb et al., 2014; Vasellati et al., 2001).
Our data showed that Passiflora tripartita var. mollissima plants had different tolerance mechanisms for the water deficit when compared to the control plants. They decrease the shoot dry biomass and invest in root growth, with high R/S values. These plants also carried out water adjustments with stomatal closure, as evidenced in the low stomatal conductance values, decreased RWC, and thinner tissues, along with a decreased xylem vessel diameter, probably to avoid cavitation, tolerate water deficits and perform better.
Ethical statement: the authors declare that they all agree with this publication and made significant contributions; that there is no conflict of interest of any kind; and that we followed all pertinent ethical and legal procedures and requirements. All financial sources are fully and clearly stated in the acknowledgments section. A signed document has been filed in the journal archives.