Introduction
Digestion involves cooperation between the gastrointestinal tract (GIT) and accessory secretory organs, such as the pancreas and liver. Food in the GIT stimulates the production of digestive enzymes from the stomach and pancreas, and bile from the liver. Digestive enzymes, including proteases, lipases, and amylase, are secreted by the pancreas into the intestine for the final breakdown and absorption of nutrients (Chikwati et al., 2013). Pepsins are one of the main proteases found in the stomach and are responsible for breaking down proteins to peptides. These enzymes are secreted in the form of pepsinogens, which are activated by the acidic pH generated by hydrochloric acid poured into the stomach (Clarks et al., 1985). Pepsin has a broad specificity. The optimum pH of pepsins is around 2, which maximises the digestion process because the substrate (protein) is under an acid denaturation (Rawlings and Salvesen, 2013). The two primary alkaline digestive proteases responsible for the initial protein hydrolysis in the intestine are trypsin and chymotrypsin. The exocrine pancreas synthesizes both proteases and stores them as non-active zymogen forms (trypsinogen and chymotrypsinogen). In the intestinal lumen, enterokinase activates trypsinogen by cleaving a short peptide, converting it to trypsin (Solovyev et al. 2023). The breakdown of di- and tripeptides is facilitated by Leucine aminopeptidase (LAP). It represents peptide digestion and leucine release, as well as amino acid absorption in the small intestine. The function of this exopeptidase is poorly understood, aside from its secretion by the mucosa of the small intestine (Bhardwaj, 2013).
In crocodiles, the digestion process begins when crocodiles swallow small preys, and larger preys are masticated before deglutination, though an excessively larger prey is reduced by ripping off bits and limbs. Next, reduction is performed in the stomach where the swallowed food is exposed to the action of hydrochloric acid and pepsin to digest protein and dissolve bones. Then, digestion continues in the upper small intestine under the action of bile and pancreatic secretions (Huchzermeyer, 2003). Digestive enzymes in reptiles have not been studied as much in other groups, for example, mammals and fishes. Unlike digestive enzymes from other groups, some reptiles’ digestive enzymes are not in the form of zymogens before ingestion, for example, in the water snake (Natrix tessellata), acinar cells of the pancreas are loaded with zymogen granules after 24 h of ingestion (Zhalka & Bdolah, 1987).
Body size in the different life stages of a species affects an organism’s energetic requirements and nutrient necessities (Radloff et al., 2012). Studies point out that nutrition in the first life stages of crocodiles is the most important for their development and future use if they want to reach a commercial size more quickly (Huchzermeyer, 2003). Proteases play a very important role in the survival and growth of any living organism, since they hydrolyse peptide bonds (Klomklao, 2008). In this sense, there are few studies that address the digestive physiology in crocodiles, including the functionality and type of proteases in these species.
In general, the digestive physiology and nutrition of crocodiles are poorly studied. In the spectacled caiman (Caiman crocodilus), when fed with 5 % of the body weight, complete gastric emptying takes an average of 99 h at 30 °C, to an average of 315 h at 15 °C, increasing the frequency and amplitude with temperature or upon feeding (Diefenbach, 1974). For the American alligator (Alligator mississipiensis), a diet based only on gizzard for 9 to 15 months reduced the levels of thiamine in blood and muscle, and over 40 % of the crocodiles died, probably because of the nutritional deficiencies in the diet (Ross & Honeyfield, 2008). In another work, the American crocodile (Crocodylus acutus) showed that when feeding with fish, hatchlings grew 1.35 times more (3.5 mm per day) than with a mixture of fish and cow liver and lung, and a diet with fly larvae (Pérez-Gómez et al., 2009). For crocodilians, growth rates differ between life stages; during the first months of life, crocodiles feed at least 6-7 times a week, and sub-adult crocodiles only need to consume 8-10 % of their body weight once a week (Whitaker & Andrews, 1998). In the broad-snouted caiman (Caiman latirostris), the growth and efficiency of food conversion are higher at 33 °C compared to 29 °C. Specimens maintained at 33 °C for 72 days were longer (9.2 ± 1 cm) and heavier (79.6 ± 13.2 g) than those maintained at 29 °C (6 ± 0.9 cm, 42.2 ± 8.5 g) (Parachú-Marcó et al. 2009).
In general, we know very little about crocodiles compared to other species, and research into their biology has been carried out for biological studies and conservation efforts. Crocodiles belong to the Crocodylidae family and are classified as reptiles, together with lizards, snakes, tuataras, tortoises, terrapins, and turtles (Huchzermeyer, 2003). Morelet’s crocodile (Crocodylus moreletii) inhabits mainly freshwater areas (marshes, swamps, ponds, rivers, lagoons, and artificial water bodies in the Atlantic lowlands of the Gulf of Mexico (Mexico) and the Yucatán Peninsula (Mexico, Belize, Guatemala) (Platt et al., 2010).
Platt et al. (2006) classified Morelet’s crocodiles by hatchlings, small juveniles, large juveniles, subadults, and adults, and found that natural preys include aquatic and terrestrial insects, arachnids, aquatic gastropods, crustaceans, fish, amphibians, reptiles, birds, and mammals, finding that smaller crocodiles fed mostly on insects and arachnids, followed by large juveniles which fed on aquatic gastropods, crustaceans, fish, and non-fish vertebrates. Adults feed on aquatic gastropods, fish, and crustaceans, however, there are reports of adult Morelet’s crocodiles consuming large mammals (over 15 kg) by kleptoparasitism (Platt et al., 2007).
In this work, we attempt to increase the basic knowledge of the digestive process of crocodiles by using Morelet’s crocodile (Crocodylus moreletii) as a model to describe digestive proteases in three life stages. This information could help in the future design of balanced diets as well to the sustainable management and production of this species.
Materials and methods
Sampling: Crocodylus moreletii (Family: Crocodylidae, Order: Crocodylia) samples, one sample per stage, came from crocodiles that underwent unexpected death associated with the cold season or attacks by organisms of the same species (cannibalism), donated from the Research Centre for the Conservation of Threatened Species in the División Académica de Ciencias Biológicas of the Universidad Juárez Autónoma de Tabasco. The donated crocodiles corresponded to three different stages: one hatchling (H), one juvenile (J) and one adult (A). All crocodiles were weighed and measured (Total length) before making a longitudinal cut in the abdomen from the beginning of the larynx to the beginning of the cloaca, extracting the complete digestive system (Fig. 1). Stomachs and intestines of the three stages of crocodile were separated. The intestine of each crocodile was measured by length and the relative intestine length (RIL) was determined by using the formula RIL = Total Length of the intestine / Total length of the crocodile (Moreira et al. 2020). Biometrics are shown in Table 1. The samples obtained were frozen (-20 °C) until processing.
Table 1 Biometrics of hatchling, juvenile and adult of Morelet’s crocodiles (Crocodylus moreletii) used for digestive proteases characterization. RIL: relative intestine length.
Crocodile Stage | Total length (cm) | Total weight (Kg) | Intestine length (cm) | RIL |
Hatchling | 33 | 0.14 | 34 | 1.03 |
Juvenile | 94 | 2.3 | 100 | 1.06 |
Adult | 184 | 28 | 182 | 0.99 |
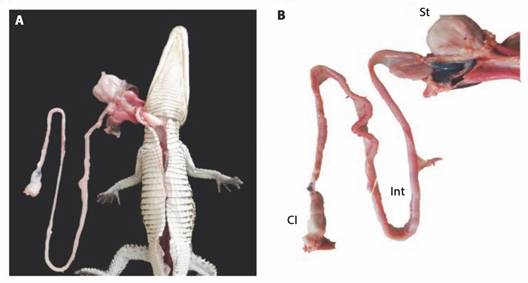
Fig. 1 Digestive system of a Morelet’s crocodile (Crocodylus moreletii) hatchling. St: stomach; Int: intestine; Cl: sewer.
Digestive enzyme activities: Dissected tissues (stomach and proximal small intestine) were homogenised in a 1:10 (w/v) ratio with distilled water using an Ultra Turrax disperser (model IKA T18 Basic). The homogenised samples were centrifuged at 12 000 rpm for 15 min at 4 °C, and subsequently, the supernatant was distributed in 2 ml tubes and stored at -20 °C until further analysis. The soluble protein concentration of the supernatant was determined by the technique of Bradford (1976).
The total acid protease activity of the stomach extracts was quantified using 1 % haemoglobin as substrate in 100 mM glycine-HCl buffer at pH 2.0, the reaction comprised 5 µl of the sample and 300 µl of the substrate-buffer mixture. After 15 min of incubation at 37 °C, the reaction was stopped with 200 µl of 10 % trichloroacetic acid (TCA), then, the samples were centrifuged at 10 000 g for 15 min at 4 °C and the absorbance of the tyrosine released in the supernatants was measured at 280 nm (Anson, 1938). Total alkaline protease activity in the intestine was determined using the technique described by Walter (1984), using 1 % casein in 100 mM Tris-HCl buffer + 10 mM CaCl2 at pH 9.0. The reaction comprised 10 µl of the sample and 300 µl of the substrate-buffer mixture. After 30 min of incubation at 37 °C, the reaction was stopped with 200 µl of 10 % TCA, then, the samples were centrifuged at 10 000 g for 15 min at 4 °C and the absorbance of the tyrosine released was measured at 280 nm. The product absorbance of both enzymes was quantified in a Genesys 10S UV-Vis spectrophotometer (ThermoScientific, Waltham, MA) and a molar extinction coefficient (ε) of 0.005 µM-1 cm-1 was used for calculations.
Chymotrypsin activity was quantified using SAApNA as substrate (Cat. No. S7388, Sigma-Aldrich, Saint Louis, MO). A 50 mM stock solution of the substrate was prepared with DMSO, then the working solution was prepared at a concentration of 1.25 mM with 50 mM Tris-HCl, pH 8.0. Fifteen microliters of each intestine extract were mixed with 135 µl of the buffered substrate to measure the absorbance of the released nitroanilide at 410 nm in a kinetics of 30 min (García-Carreño et al., 1994). Trypsin activity was quantified using BAPNA as a substrate (Cat. No. B4875, Sigma-Aldrich). A stock solution of 122.78 mM of the substrate was prepared with DMSO and the working solution was prepared by diluting the stock solution to a concentration of 2 mM with 50 mM Tris-HCl, pH 8.0. Fifteen microliters of each intestine extract were mixed with 135 µl of the substrate to measure the absorbance of the nitroanilide released at 410 nm in a kinetics of 15 min (García-Carreño et al., 1994).
Leucine aminopeptidase activity was quantified using L-leucine-p-nitroanilide as substrate (Cat. No. L9125, Sigma-Aldrich). A 250 mM stock solution of the substrate was prepared with DMSO, and the working solution was prepared at a concentration of 4 mM diluted with 50 mM sodium phosphate, pH 7.2. Fifteen microliters of each intestine extract were mixed with 135 µl of the substrate and buffer mixture to measure the absorbance of the released nitroanilide at 410 nm over a 30 min kinetics (Maroux et al., 1973). Elastase activity was measured using the method of Ishida et al. (1987) with some modifications.
In this work we quantified the activity using N-Succinyl tri-L-alanine-4-nitroanilide (SAAANA; Cat. No. S4760, Sigma-Aldrich) as substrate in a kinetics instead a final point measurement. A 50 mM stock solution of the substrate was prepared with DMSO, and the working solution was prepared at a concentration of 1.5 mM diluted with 100 mM Tris-HCl buffer at pH 8. Fifteen microliters of each intestine extract were mixed with 135 µl of the substrate and buffer mixture to measure the absorbance of the released nitroanilide at 410 nm over a 30 min kinetics. The product absorbance was quantified in a xMark spectrophotometer (Bio-Rad, Hercules, CA), and a molar extinction coefficient (ε) of 0.0088 µM-1 cm-1 was used for calculations. Total activity (Units/ml) = ( Δabs*reaction final volume (ml)) / (MEC*time (min)*extract volume (ml)) and Specific activity (Units / mg prot) = Total activity / soluble protein (mg / ml), where Δabs represent the increase in absorbance, and MEC represents the molar extinction coefficient (García-Carreño et al., 1994).
Optimum pH and temperature: For acid proteases, total alkaline proteases, trypsin, and leucine aminopeptidase, the optimum temperature was determined using the enzymatic techniques described above, changing the temperature to 10, 20, 30, 40, 50, 60, 70, and 80 °C. Optimum pH was also determined for the same enzymes mentioned above using previously described enzymatic techniques at 37 °C but adjusted with the following buffers: 100 mM glycine-HCl for pH 1, 2, 3 and 4; 100 mM sodium acetate for pH 5 and 6; 100 mM Tris-HCl for pH 7, 8 and 9; 100 mM glycine-NaOH for pH 10 and 11.
Electrophoretic analyses: Electrophoretic analyses were performed in a Mini-PROTEAN 3 Cell electrophoretic chamber (Bio-Rad) using vertical gel plates (8 × 10 × 0.1 cm). For the analysis of acid proteases, the stomach extracts of C. moreletii were separated by non-denaturing native conditions (Native-PAGE) using a continuous acrylamide gel (10 %) in 25 mM Tris and 192 mM glycine buffer at pH 8.3. After the electrophoresis, the gels were incubated in haemoglobin to reveal protease isoforms according to the procedure of Díaz-López (1998). The gels were soaked in 100 mM glycine-HCl buffer at pH 2.0 to activate acid proteases. After 15 min, the gels were immersed for 60 min at 4 °C in 2 % haemoglobin in 100 mM glycine-HCl buffer, pH 2.0, and then placed for 60 min in the same solution, but at 37 °C. After the incubation, the gels were washed with distilled water and fixed in a 12 % trichloroacetic acid (TCA) solution for 15 min.
For the analysis of alkaline proteases, the intestine extracts of C. moreletii were used. The electrophoresis gel was prepared with a 4 % polyacrylamide (PAA) stacking gel and a 10 % PAA resolving gel. Electrophoresis was performed under denaturing conditions (SDS-PAGE) with SDS in 0.1 % in 25 mM Tris and 192 mM glycine buffer at pH 8.3, adapted by García-Carreño et al. (1993). After alkaline SDS-PAGE electrophoresis, the gels were immersed for 60 min at 4 °C in a 2 % casein solution in 0.1 M Tris-HCl buffer, pH 9. Then, gels were incubated for 60 min in the same solution at 37 °C, then washed and fixed with TCA as described above. To detect enzyme activity, after incubation with the substrates, the gels were stained using a Coomassie R-250 brilliant blue solution at 0.1 %, while destaining was carried out in a 35:10:55 solution of methanol-acetic acid-water. Clear bands revealed protease activity within a few minutes, although well-defined bands were only obtained after a 2-4-hour staining period.
Electrophoresis with stomach extracts was complemented with the use of the specific inhibitor pepstatin A (PepA, 1 mM), incubating for 1 hour at 37 °C. A set of molecular weight markers (Broad range leader, Cat #161-0318, Bio-Rad) was applied on each Native-PAGE and SDS-PAGE (5 μl per well) and the molecular weight (MW) of each band was calculated using a linearly fitted relationship between Rf and log10 of the molecular weight markers, using Quality One software version 4.6.5 (Bio-Rad, Hercules, CA).
Statistical analysis: Even though this is a descriptive study using an organism per stage, all enzymatic assays were performed in triplicate. Data of specific activity of the different proteases between the different stages, as well as the characterisation of pH and temperature between each stage were analysed for normality and homoscedasticity, and in the case of fulfilling these assumptions, a parametric one-way ANOVA analysis was used. If the aforementioned assumptions were not met, a non-parametric Kruskal-Wallis analysis was performed. The tests showing differences between the variables were analysed with a Tukey’s posterior test. All analyses were performed in the Sigma Plot 11.0 program with a significance of P < 0.05.
Results
Biometrics of hatchling, juvenile and adult of Morelet’s crocodiles (Crocodylus moreletii) used for digestive proteases characterisation are shown in Table 1. The relation between total length and intestine length, reported as relative intestine length, was near to one in the three stages.
The specific activity of acid proteases showed significant differences between the three stages, presenting greater activity in the juvenile and less activity in the hatchling. Total alkaline protease activity showed significant differences between the three stages, with higher activity in the hatchling and lower activity in the adult. Trypsin, chymotrypsin, elastase, and leucine aminopeptidase activities showed higher activity (P < 0.05) in hatchling and less activity in juvenile and adult (Table 2).
Table 2 Specific activity (U/mg protein) of acid and alkaline proteases, trypsin, chymotrypsin, elastase, and leucine aminopeptidase (mean ± SD) in hatchling, juvenile and adult of Morelet’s crocodiles (Crocodylus moreletii). *, **, *** indicate differences between each enzyme activity and stage.
Specific activity (U/mg protein) | Hatchling | Juvenile | Adult |
Acid proteases | 69.68 ± 6.19*** | 5 819.7 ± 6.12* | 518.44 ± 18.35** |
Alkaline proteases | 43.04 ± 0.59* | 19.22 ± 4.14** | 2.02 ± 0.31*** |
Trypsin | 22.12 ± 0.8* | 0.7 ±0.3** | 0.9 ± 0.4** |
Chymotrypsin | 1.1 ± 0.2* | 0.2 ± 0.2** | 0.1 ± 0.1** |
Elastase | 0.9 ± 0.1* | 0.1 ± 0.1** | 0.3 ± 0.1*** |
Leucine aminopeptidase | 29.5 ± 0.8* | 15.1 ± 1.1** | 13.9 ± 0.3** |
In Fig. 2A, an optimum pH of 2 is observed for acid proteases of the juvenile and adult crocodiles, while, for hatchling, its optimum activity was found at pH 2 and 3. It is worth noting the decrease in activity at pH 4 and 5 for the adult stage, while hatchling and juvenile stage showed activities near to 50 % of relative activity. Fig. 2B shows the maximum of alkaline protease activity between pH 8 to 11 for hatchling. The juvenile presented its maximum peak of activity at pH 9 also showing a peak of activity at pH 5, with 80 % of relative activity, while the adult stage presented the maximum peak of activity at pH 8, also showing a peak of activity at pH 5, with 60 % of relative activity.
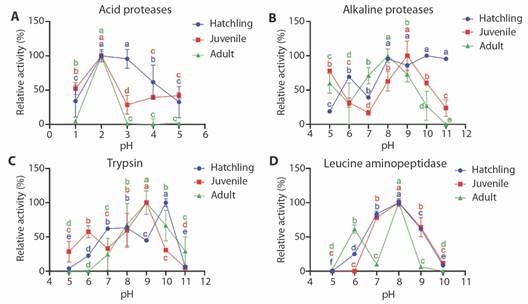
Fig. 2 Effect of pH on the relative activity (%) of acid proteases (A), alkaline proteases (B), trypsin (C) and leucine aminopeptidase (D) of digestive enzymes obtained from hatchling, juvenile and adult of Morelet’s crocodiles (Crocodylus moreletii).
Fig. 2C shows that trypsin from hatchling has a maximum activity at pH 10, showing a peak of activity at pH 7 and 8 with a relative activity of approximately 60 %. Trypsin activity in the juvenile has a maximum activity at pH 9, showing a peak of activity at pH 6 with a relative activity of approximately 60 %, while the adult stage presents its maximum peak of trypsin activity at pH 9. Fig. 2D shows the activity of leucine aminopeptidase with a maximum peak in the three stages at pH 8, while in adult stage showed a second peak at pH 6 with a relative activity higher than 60 %.
In Fig. 3A, differences were observed in the optimum temperature for acid protease activity in the three stages, finding the maximum activity at 30, 40 and 50 °C for hatchling, juvenile and adult, respectively. Fig. 3B shows differences in the optimum temperature for alkaline proteases between the three stages, finding the maximum activity at 50 and 60 °C for juvenile and hatchling, respectively, where the juvenile shows approximately 60 % of the total activity at 10 °C. The adult stage showed two peaks of maximum activity (40 and 60 °C). Fig. 3C shows two peaks of trypsin activity in hatchling and adult at 30 and 50 °C, finding almost no activity for the three stages at 40 °C, while the juvenile showed maximum activity at 30 °C, and as observed for alkaline proteases, trypsin activity in the juvenile shows approximately 50 % of the total activity at 10 °C. Fig. 3D shows the differences in the optimum temperature for leucine aminopeptidase activity between the three stages, showing maximum activity at 40 and 50 for juvenile and hatchling, respectively, and between 50 and 60 °C for adult stage. As observed in alkaline proteases and trypsin, the relative activity of LAP in juveniles shows approximately 75 % of the total activity at 10 °C.
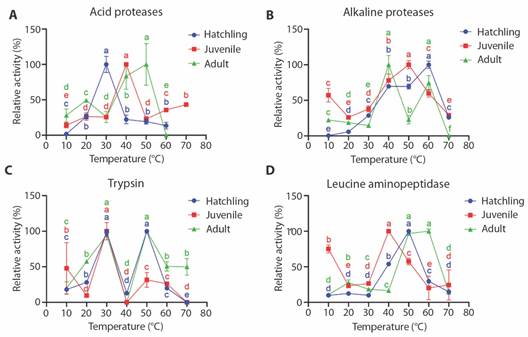
Fig. 3 Effect of temperature on the relative activity (%) of acid proteases (A), alkaline proteases (B), trypsin (C) and leucine aminopeptidase (D) of digestive enzymes obtained from baby, juvenile and adult of Morelet’s crocodiles (Crocodylus moreletii).
The results of the electrophoresis (Native-PAGE) are shown in Fig. 4, which shows four bands with acid protease activity from the hatchling and juvenile stomachs with calculated weights of 51.2, 39.4, 30.9 and 21.2 kDa, while the adult only revealed two bands (39.4 and 30.9 kDa). The same figure shows that the incubation with pepstatin A inhibited the four bands in all the crocodile stages. In Fig. 5, the results of SDS-PAGE revealed six bands with alkaline protease activity from the hatchling proximal intestine with calculated weights of 51.2, 41.7, 33.1, 26.3, 22.2, 15.2 kDa. The juvenile stage showed no activity bands, while in the adult, two bands were revealed (26.3 and 15.2 kDa).
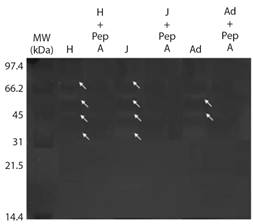
Fig. 4 Native-PAGE electrophoresis (Native-PAGE) of acid proteases obtained from the hatchling, juvenile and adult stomach of Morelet’s crocodile (Crocodylus moreletii) at pH 2.
Discussion
Pepsins are very important endopeptidases in carnivorous species, as they initiate protein digestion, releasing peptides and some free amino acids (Gilberg et al., 1990). In fasted painted turtles (Chrysemys picta), the pH varies between cardiac and pyloric stomach (1.8 and 2.1, respectively), and after feeding, the pH increases, reporting average values from 2.2 to 4.0 (Fox & Musacchia, 1959). To date, there are no reports of pepsin characterization in crocodiles, this being the first report in C. moreletii, where the optimum pepsin activity found agrees with that reported in most fishes, showing two or three forms of pepsin with optimum activity between 2 and 4 (Klomklao, 2008). Unfortunately, we were not able to measure the pH of the sampled stomachs nor having more organisms to sample, however, the optimum pH found for pepsins agrees with the pH of the stomach after feeding in other reptile species such as white turtle (Dermatemys mawii) and the red-eared turtle (Trachemys scripta elegans) that show optimum acid protease activity at pH 2 and pH 2.5, respectively (Rangel-Mendoza et al., 2018; Sun et al., 2007).
Pepsins reported in marine fish range between 27 and 42 kDA (Simpson, 2000). In the present study, the electrophoresis results showed four bands with proteolytic activity in the stomach (21.2, 30.9, 39.4 and 51.2 kDa), which were inhibited with pepstatin A. The four bands were found in extracts from hatchling and juvenile, while in adult, only two bands were revealed (30.9 and 39.4 kDa). In this case, the presence of four pepsin isoforms can generate digestive adaptations as found in the hatchling, where the optimal pH of pepsin was found at 2 and 3, while in juvenile and adult, the optimum pH was 2. This diversification was also observed for optimum temperature, which was different for the three stages.
Pancreatic digestive proteases play a central role in digestion by hydrolysing proteins into peptides and amino acids, which are essential for proper growth, development, repair, energy, and every type of body function (Whitcomb & Lowe, 2007). Pancreatic digestive enzymes in snakes increase after ingestion, for example, in the water snake (Natrix tessellate), the activity of chymotrypsin and elastase increased over three-fold after 24 h of ingestion (4.1 ± 1.1 to 13.0 ± 3.4 U/mg for chymotrypsin, and 4.1 ± 2.5 to 13.8 ± 7.1 U/mg for elastase) and in the sand viper (Vipera ammodytes), the activity increased tenfold after 24 h of ingestion (1.1 to 12.7 U/mg for chymotrypsin, and 0 to 17.8 U/mg for elastase) (Alcon & Bdolah, 1975). In our work, the activity of chymotrypsin and elastase was lower (Table 2), however, the activity of trypsin and leucine aminopeptidase were in similar values for the ones reported for snakes. Chymotrypsin and elastase are enzymes with a specific range of cleavage sites, so their low activity could suggest that Morelett’s crocodile need protein with a high content of lysine and arginine corresponding to carnivorous diet (Rawlings and Salvesen, 2013).
Coulson & Coulson (1986) reported that temperature affects the rates of digestion, amino acid absorption, and assimilation in alligators (Alligator mississipiensis); at 25 °C, digestion was slow and incomplete, at 28 °C, digestion was about 50 %, and 31 °C, was the optimum temperature for digestion since the initial rate of the rise in total and essential amino acids in plasma, and specifically, alanine, was greater, since at 31 °C, the highest concentration of alanine in plasma was found after 40 min after ingestion, compared to 28 °C (70 min) and 25 °C (100 min). Those results could be related to the efficiency of digestive proteases, in this work, the optimum temperature for trypsin was found at 30 °C for the three animals, even though, physiological temperature does not determine the optimum activity of an enzyme, for C. moreletii, its natural habitat varies between 26 and 28 °C, which opens new questions about the optimum temperature for the species cultivation (Casas-Andreu et al., 2011).
Total alkaline protease activity in C. moreletii hatchling was the highest (43.04 ± 0.59 U/mg) compared to the other stages. This high activity is also reflected in pancreatic (trypsin, chymotrypsin, and elastases) and cytosolic (leucine aminopeptidase) proteases, denoting a high diversity of proteases in hatchling. The results of optimal pH of alkaline protease activity in C. moreletii hatchling show stability between pH 8 and pH 11, suggesting that the sum of the optimum pH of several types of proteases, confer the ability to the hatchling to have high activity in a wide pH range. This assumption is corroborated by the optimum activity obtained for trypsin and leucine aminopeptidase, which shows the highest activity at pH 10 and pH 8, respectively. Studies in reptiles such as the white turtle (Dermatemys mawii), show optimum alkaline protease activity at pH 9 (Rangel-Mendoza et al., 2018), while the red-eared turtle (Trachemys scripta elegans) shows optimum pH in pancreas, and foregut, midgut, and hindgut of 8.0, 7.0, 8.0, and 8.5, respectively (Sun et al., 2007). Tracy et al., (2015), reported the digestive morphology, the activity of membrane enzymes (aminopeptidases, maltase and sucrase) and the absorption of nutrients in Alligator mississippiensis and Crocodylus porosus, where it was found that the villi of the proximal intestine varied in the two species, having A. misisipiensis the highest absorption and aminopeptidase activity compared to C. porosus. For C. moreletii, the activity of leucine aminopeptidase was detected, having a higher activity compared to other digestive proteases for this species, denoting the importance of the cytosolic hydrolysis of peptides for the digestibility of proteins in the species.
In addition to the above, the results of SDS-PAGE in C. moreletii hatchling showed six bands with proteolytic activity with molecular weights of 15.2, 22.2, 26.3, 33.1, 41.7, 51.2 kDA, of which two (22.2 and 26.3 kDa) are within the molecular weights reported for trypsins in fish (Jesús-De la Cruz et al., 2018) and they show the highest activity in the zymogram. No bands were revealed in the juvenile, while two bands (15.2 and 26.3 kDA) were revealed in the adult, which are also present in the hatchling, where one of these bands corresponds to the molecular weights reported for trypsins.
Some species have better catalytic efficiency at low temperatures (Klomklao, 2008). In the case of C. moreletii, it is denoted in the juvenile stage that presents a peak of activity of alkaline proteases, trypsin, and leucine aminopeptidase at a temperature of 10 °C, an effect not found in hatchling and adult. This result denotes the importance of studying the diversification of enzymes and/or isoforms that improve the digestion capacity under extreme conditions, especially in ectotherms.
The current investigation was carried out using only the stomachs and intestines of a single specimen of each stage, this is because the species is regulated (NOM-059-SEMARNAT-2010), which generates difficulty in the process of obtaining the species’ biological material. Working with only one organism of each stage can influence the results, since these will not only depend on age but also on the nutritional status and possible digestive phenotypes in reptiles (Venesky et al., 2013). However, the results obtained lay the foundations for understanding the digestive physiology of the Morelet’s crocodile (C. moreletii), opening opportunities for future research. In this sense, it is recommended to carry out research related to the activity and functionality of lipases and carbohydrases, as well as to evaluate the in vitro digestibility of different commercial flours and oils, with the aim of selecting ingredients as the basis for the formulation of specific diets for the species.
Conclusion
There are differences in the specific activity of the Morelet’s crocodile between the three stages, presenting a greater activity in acid proteases in the juvenile unlike the other stages, while the hatchling presented greater activity for alkaline proteases, trypsin, chymotrypsin, elastase, and leucine aminopeptidase compared to the other stages, denoting greater protein digestion capacity in the alkaline digestive phase. There are differences in the optimum pH and temperature for the activity of acid, alkaline, trypsin, chymotrypsin, and leucine aminopeptidase proteases between the three stages, an effect that demonstrates the diversification of the enzymes according to different ages, as well as the presence of specific isoforms in each age of C. moreletii. The acid phase zymogram showed four bands with pepsin-like acid activity in the hatchling and juvenile crocodiles, while in the adult, only two of the four bands were present. The zymograms showed that the hatchling crocodile presented the highest number of bands in the alkaline phase compared to the other stages, which corresponds to the high specific activity reported in the alkaline phase.
Ethical statement: the authors declare that they all agree with this publication and made significant contributions; that there is no conflict of interest of any kind; and that we followed all pertinent ethical and legal procedures and requirements. All financial sources are fully and clearly stated in the acknowledgments section. A signed document has been filed in the journal archives.