Introduction
Scleractinian corals are the main engineers of coral reef ecosystems, due to their capacity to precipitate calcium carbonate (CaCO3), forming tridimensional structures that constitute the base of the physical reef-framework and providing ecosystem services and habitat to associated biodiversity (Álvarez-Filip et al., 2009; Sheppard et al., 2010). Coral reef ecosystems have been threatened by natural events (heatwaves, hurricanes, diseases, and others), and human-derived activities (coastal-development, nutrient input, overfishing, and marine pollution), causing large coral mortality (up to 50 %) in Eastern Tropical Pacific (ETP) over the last three decades (De’ath et al., 2009; Rinkevich, 2015). As natural recovery occurs slowly (decades or centuries), many active restoration tools have emerged as an alternative strategy to accelerate coral recovery and mitigate the rapid coral reef degradation (Rinkevich, 2020). Direct coral transplantation is one of the most used techniques as it avoids the early-stage of coral farming/nursering and post-outplanting stress, which have resulted in high survival and growth rates (Harriott & Fisk, 1988; Tortolero-Langarica et al., 2014). Also, artificial substrate (typically comprised of ceramic, concrete, or terracotta, among others) is most likely to aid in reef conservation and restoration by providing nursery habitat for target species or recruitment substrate for corals and other organisms (Hylkema et al., 2021; Monchanin et al., 2021). A coral reef restored not only leads to an increase in coral coverage but also improves the increase of structural heterogeneity and calcium carbonate production, facilitating the recovery/maintenance of coral reef habitats (Lindahl, 2003; Tortolero-Langarica et al., 2014).
Branching coral species are commonly used for testing the efficiency of coral restoration techniques due to their fast-growth and 3-D properties (Rinkevich, 2019; Rinkevich, 2020), and also allows to determine the long-term potential success of restoration initiatives (Tortolero-Langarica et al., 2019). However, branching corals are considered one of the most sensitive species to abrupt environmental changes such as temperature anomalies, light irradiance, including extreme hydrodynamic conditions (tropical storms and swells), causing negative effects on coral calcification (Allemand et al., 2011; Prachett et al., 2015). Hence, coral growth assessment provides insights to understand the species’ response to environmental fluctuations of seasonal seawater temperature, thermal-stress events, and substrate availability during and after the restoration (Grigg, 2006; Lough & Cooper, 2011; Rinkevich, 2019).
Along the ETP, pocilloporid corals comprise the most abundant coral genera in shallow reef areas (Glynn & Ault, 2000), but also has been the most affected by heatwaves events causing massive bleaching and high mortalities (> 90 %) (Carriquiry et al., 2001; Glynn, 2000; Glynn, 2001), with different recovery trajectories among ETP’s coral reef locations (Cruz-García et al., 2020; Romero-Torres et al., 2020). Coral reef recovery has been quicker after a bleaching event in some sites because of its oceanographic conditions (Cruz-García et al., 2020). For example, Marietas islands are in a place with seasonal upwellings and internal waves, so seawater temperature does not rise as much as Isabel island, which is on the continental shelf with shallow water and higher positive thermal anomalies (Godínez et al., 2010). Therefore, the total live coral cover on Isabel island is lower than on Marietas islands (Hernández-Zulueta et al., 2017). Despite these differences in coral recovery, few attempts of coral restoration actions have been tested along the region (Liñán-Cabello et al., 2011; Muñiz-Anguiano et al., 2017; Nava & Figueroa-Camacho, 2017; Tortolero-Langarica et al., 2014; Tortolero-Langarica et al., 2019; Tortolero-Langarica et al., 2020). In particular, in the Central Mexican Pacific (CMP), there is still insufficient information on the effectiveness of different restoration techniques, based on coral growth and survival data (Tortolero-Langarica et al., 2019). This study presents the first restoration approach at the Isla Isabel National Park, Mexico, using two different substrates (natural and artificial) and the comparisons of three pocilloporid morpho-species (P. cf. damicornis, P. cf. capitata, and P. cf. verrucosa) through linear extension and tridimensional (3D) growth (live tissue area) during a one-year period of restoration. Due to the different characteristics of both substrates, three morpho-species, and the environmental condition variation along the year, we expected coral growth differences in all these factors.
Materials and methods
Study area: Isla Isabel National Park (IINP) (21°50’50’’ N - 05°53’10” W) is a volcanic island with a surface area of 82.1 ha, located within the CMP (Fig. 1). The study site is located in a transitional oceanographic region, seasonally influenced by the California Current, the North Equatorial Current, and the Gulf of California water mass (Badan, 1997). In summer, seawater temperature ranges from 23.5 to 32.7 ºC, while in winter ranges from 18.6 to 29.7 ºC (CONANP, 2005). The IINP harbors an important coral community, with small fringing and rocky reefs with a high coverage of hermatypic corals (10.7 %) of the genera Pocillopora, Pavona, and Porites (Galván-Villa et al., 2010; Hernández-Zulueta et al., 2017; Ríos-Jara et al., 2008; Tortolero-Langarica et al., 2016). These coral ecosystems are characterised by a high associated biodiversity (Galván-Villa et al., 2010; Hermosillo-Núñez et al., 2015; Rodríguez-Zaragoza et al., 2011), and act as a “stepping stone” that contributes to the connectivity of marine species along the CMP (Briggs & Bowen, 2013; Galván-Villa et al., 2010; Glynn & Ault, 2000).
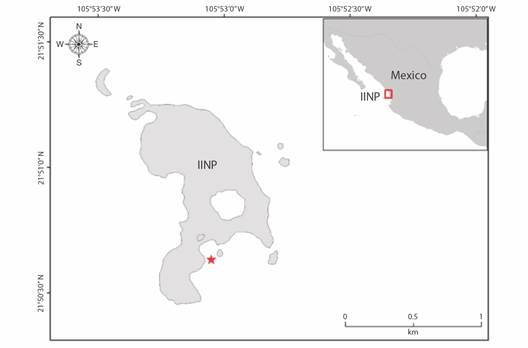
Fig. 1 Study area in Isla Isabel National Park (IINP) at Mexican Pacific Coast. Red star: coral restoration area.
Coral samples: The coral restoration was conducted from August 2010 to August 2011 using two substrata types: 1) steel-stacks stabilized into the natural substrate, and 2) semispherical concrete modules (Ø = ~1 m) with steel-stacks, considered as artificial substrate. Both treatments were installed at 2-4 m depth with a distance of 2-3 m between them (Fig. 2). Coral fragments of opportunity (~10 cm) were hand collected with SCUBA from the nearby area. Each fragment was visually examined and selected to avoid those with partial dead or bleaching but also the invasion of algae and sponges (i.e., Cliona spp. and Thoosa spp.) (Nava & Carballo, 2008).
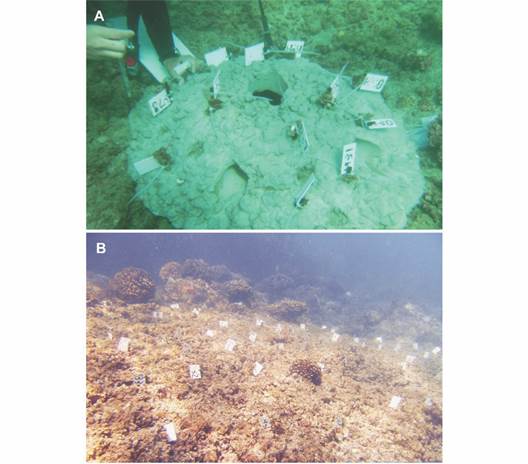
Fig. 2 Coral restoration of Pocillopora species using A. artificial and B. natural substrata at Isla Isabel National Park.
Every three-months (August 2010, November 2010, February 2011, and May 2011), 72 coral fragments were installed and then stained with alizarin red at a concentration of 0.02 gl l-1 (Sigma®) for 15 hours and fixed with plastic ties in both natural and artificial substrata (P. cf. damicornis n = 12, P. cf. capitata n = 12 and P. cf. verrucosa n = 12, for each substrate; These species were chosen because they have the highest coverage and frequency in the CMP). After each growth period (three-monthly = quaternary-1), coral fragments were extracted and bleached with 10 % sodium hypochlorite for 12 hours for further growth analyses. Coral surface area growth was estimated by evaluating the surface area increase (mm2) using the aluminium foil technique described by Marsh (1970), and the extension growth (mm) fragments were cut into slices (~30 x 20 x 10 mm) using a tipped diamond saw blade (Qep®). Each coral slice was individually photo-documented with a Panasonic DMC-FS7 camera with a high resolution (300 dpi) using a common rule as standard reference (precision of 0.1mm); growth was determined as apical height measured from the alizarin mark to the top of each coral sample with ImageJ (v.1.41) software (Rasband, 2012).
The seawater temperature (SWT) was recorded using Onset HOBO® (precision of ± 0.5 °C) thermographs installed in situ with a set record of 20 minute intervals. Data were pooled during the warm season (July-November 2010), and cold season (December 2010-June 2011), and used to relate to coral growth parameters.
Data analysis: To determine the effect of time, substrate and temperature into the growth parameters, extension rate and surface area were analysed using a three-way crossed analyses of variance based on permutations (ANOVA), which was built with an Euclidean distance matrix following Anderson et al. (2008). These unrestricted analyses were used as data were not parametric. Differences among species, between substrates and across to periodicity were tested for each technique based on the next model:
where Y is the analyzed variable (i.e., linear extensions and surface area), µ is the variable’s average, Sp i is coral Morphospecies factor (P. cf. damicornis, P. cf. capitata and P. cf. verrucosa), ST j is Substrata Type (artificial or natural), P k is sampling periodicity (four sampling periods) and e ijk is the accumulated error.
All factors were fixed (model type I). Statistical significance was tested using a sum of squares type III (partial) and 10 000 permutations of residuals under a reduced model and a sum of squares type III (partial). Pairwise comparisons (permutational t-tests) were used when significant differences were found in the factors or their interaction. The analyses were performed using PRIMER 7.0.21 software (Anderson et al., 2008). Finally, simple linear regressions were performed to determine the relationship between temperature, extension rate, using a coefficient of determination (r2). For all regression models, 95% confidence intervals were estimated and global test was evaluated using a least-squares procedure in SigmaPlot Ver. 11 software (Systat Software, Inc.).
Results
Extension growth rate: The factors of Morphospecies and Periods, and the triple interaction explained most of the variation observed in coral growth rates (Table 1). Those differences were attributed to the Period factor (Appendix 1), where temperature influenced most of these differences in warmer seasons (min. 23.5 °C, max. 32.7 °C) rates varied between 2.45 to 7.49 mm quarterly-1, meanwhile in cold seasons (min. 18.6 °C, max. 29.7 °C) varied between 1.28 to 6.20 mm quarterly-1 (Table 2, Fig. 3, Fig. 4). Morphospecies factor showed that P. cf. damicornis has the lowest growth rate in all periods (3.39 ± 1.8 mm quarterly-1), meanwhile P. cf. capitata (4.33 ± 2.3 mm quarterly-1) and P. cf. verrucosa (4.24 ± 2.0 mm quarterly-1) resulted with the highest growth rate (Table 2, Fig. 4, Appendix 2). The accumulated annual growth rates were of 12.38 mm/yr-1 for P. cf. damicornis, 16.25 mm yr-1 for P. cf. capitata, and 16.33 mm yr-1 for P. cf. verrucosa (Fig. 5). Substrata Type did not influence differences in coral growth (Fig. 5, Appendix 3).
Table 1 Results of three-way PERMANOVA with crossed and fixed factors.
Source of variation | ER | SAG | ||||
Pseudo-F | P(perm) | Perms | Pseudo-F | P(perm) | Perms | |
Spp | 2.65 | 0.0001 | 9 955 | 4.82 | 0.0001 | 9 950 |
P | 61.77 | 0.0001 | 9 948 | 18.9 | 0.0001 | 9 956 |
ST | 13.15 | 0.5111 | 9 844 | 0.23 | 0.2680 | 9 938 |
Spp x P | 7.09 | 0.0001 | 9 935 | 8.05 | 0.0001 | 9 936 |
P x ST | 3.61 | 0.4061 | 9 955 | 0.55 | 0.0184 | 9 952 |
Spp x ST | 0.78 | 0.198 | 9 949 | 1.5 | 0.4780 | 9 957 |
Spp x P x ST | 4.39 | 0.0078 | 9 938 | 2.19 | 0.3679 | 9 931 |
Table 2 Coral growth rates per period, substrata type, and species.
Aug-Nov (cold season) | Nov-Feb (cold season) | Feb-May (warm season) | May-Aug (warm season) | ||||||
Natural substrate | Artificial substrate | Natural substrate | Artificial substrate | Natural substrate | Artificial substrate | Natural substrate | Artificial substrate | ||
Linear extension | mm | mm | mm | mm | mm | mm | mm | mm | |
P. cf. damicornis | 4.10 ± 0.70 | 4.74 ± 0.96 | 1.28 ± 0.39 | 1.98 ± 1.89 | 2.52 ± 0.86 | 2.45 ± 0.74 | 5.16 ± 2.07 | 4.35 ± 1.56 | |
P. cf. capitata | 4.53 ± 1.13 | 4.69 ± 1.61 | 2.95 ± 2.0 | 2.18 ± 0.82 | 2.99 ± 1.46 | 2.43 ± 0.97 | 6.82 ± 1.20 | 7.49 ± 1.51 | |
P. cf. verrucosa | 4.81 ± 1.01 | 6.20 ± 1.87 | 2.60 ± 0.81 | 1.95 ± 0.74 | 3.39 ± 1.81 | 3.97 ± 0.66 | 4.65 ± 2.40 | 5.79 ± 1.93 | |
Surface area | mm2 | mm2 | mm2 | mm2 | mm2 | mm2 | mm2 | mm2 | |
P. cf. damicornis | 93.65 ± 36.02 | 138.57 ± 73.94 | 23.78 ± 15.35 | 60.71 ± 81.80 | 97.23 ± 51.16 | 79.79 ± 33.27 | 128.06 ± 71.84 | 79.17 ± 63.27 | |
P. cf. capitata | 92.44 ± 55.25 | 223.89 ± 166.0 | 90.27 ± 58.98 | 64.94 ± 37.91 | 105.29 ± 137.7 | 98.2 ± 76.36 | 328.32 ± 145.24 | 411.31 ± 120.23 | |
P. cf. verrucosa | 248.27 ± 224.23 | 299.95 ± 232.82 | 151.92 ± 104.18 | 65.54 ± 26.94 | 143.66 ± 100.88 | 120.68 ± 58.79 | 138.11 ± 107.67 | 179.79 ± 97.12 |
Mean ± standard deviation. Codes: Aug = August, Nov = November, Feb = February, May = May, NS = Natural substrate, AS = Artificial substrate.
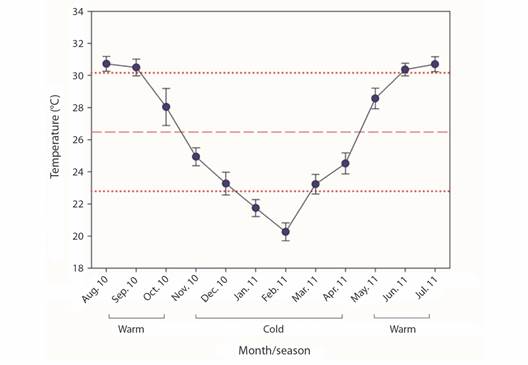
Fig. 3 Monthly seawater temperature (mean ± SD) of Isla Isabel National Park, Mexico. Red dash and dotted lines represent mean annual ± SD temperatures, respectively.
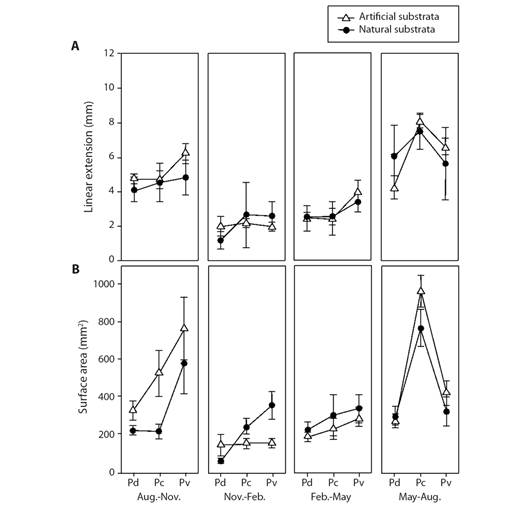
Fig. 4 Comparison of coral growth rates between natural and artificial substrata. Black circles are natural substrates; white circles are artificial substrates. Codes: Pd = Pocillopora cf. damicornis, Pc = Pocillopora cf. capitata, Pv = Pocillopora cf. verrucosa.
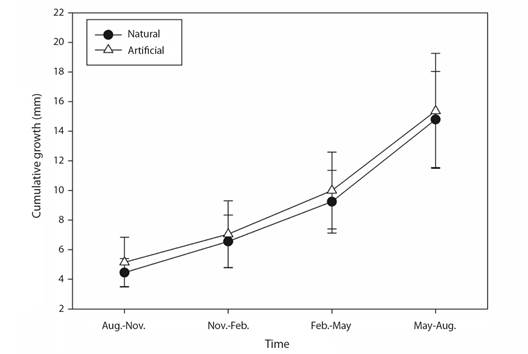
Fig. 5 Cumulative coral extension growth (mean ± SD) of Pocillopora species over one-year restoration using two different substrates. Black circles = natural, and white triangles = artificial substrate.
Surface area: The results showed that double interactions “Morphospecies x Period” and “Period x Substrata Type” explained the surface area variation (Table 1). The morphospecies x period interaction showed differences in coral growth rates during the warm period and among morphospecies varied between 79.17 ± 33.27 mm2 quarterly-1 to 411.31 ± 120.23 mm2 quarterly-1, and during the cold period, growth rates varied from 23.78 ± 15.35 mm2 quarterly-1 to 226.48 ± 227.24 mm2 quarterly-1 (Table 2, Fig. 3, Fig. 4, Appendix 4). Regarding morphospecies factor, P. cf. damicornis showed the lowest annual tissue area increase (34.14 mm2 yr-1) compared with P. cf. capitata (70.73 mm2 yr-1) and P. cf. verrucosa (65.67 mm2 yr-1) (Table 2, Fig. 3, Fig. 4, Appendix 5). Substrata Type x Period interaction showed the same pattern tissue growth was higher during the warm season (158.0 mm2 yr-1 in natural substrate and 158.2 mm2 yr-1 in artificial substrate) than cold season (108.63 mm2 yr-1 in natural substrate and 146.69 mm2 yr-1 in artificial substrate) (Table 2, Fig. 3, Fig. 4, Appendix 6). Nevertheless, substrata type factor exhibited non-significant influences in the live coral tissue area (P > 0.05).
Linear regression models showed that extension rate and tissue area growth were positively related to SST in both substrate types (Fig. 6). The corals P. cf. damicornis and P. cf. capitata showed a significant relation with SST in both methods, while P. cf. verrucosa were significant only with extension rate (Fig. 6).
Discussion
Direct transplantation is one of the most successful methods used for the coral restoration of damaged coral reefs (Boch & Morse, 2012; Boström-Einarsson et al., 2020; Edwards, 2010; Edwards & Gomez, 2007; Rinkevich, 2014; Rinkevich, 2019; Young et al., 2012), along both coastal and insular areas. (Ishida-Castañeda et al., 2019; Tortolero-Langarica et al., 2020). The direct outplanting of fragments of opportunity in IINP resulted as efficient in terms of coral growth, regardless of the substrate, confirming the feasible and potential of using Pocillopora coral species fixed to natural or artificial substrata as a technique for coral restoration along the CMP (Nava & Figueroa-Camacho, 2017; Tortolero-Langarica et al., 2014; Tortolero-Langarica et al., 2019).
During 2010-2011, a period of negative thermal stress (La Niña event) influenced the study area, with temperatures < 1.3 °C for a period of eight months (NOAA, 2022). Thermal stress conditions elicit the expulsion of the algae-symbiont, which provides 90 % of the energetic budget used for the coral growth and reproduction process (Van Oppen & Blackall, 2019). The optimal temperature for coral growth rates in the ETP region is ranged from 26-29 °C during a neutral ENSO period, yet at La Niña event, there are sub-optimal temperatures that could promote coral growth rate decay from 20-50 % (Tortolero-Langarica et al., 2016). Nevertheless, coral growth may vary among reef locations due to different local and temporal environmental conditions (Tortolero-Langarica et al., 2017). In this study, Pocillopora species increased two times compared with their initial size (rising from 178 to 442 %), which agrees with Tortolero-Langarica et al. (2017), whose study site is near our study site and has similar environmental conditions. Even various authors report similar growth rates for Pocillopora corals in several studied years throughout the ETP (Glynn, 1977; Guzmán & Cortés, 1989; Jiménez & Cortés, 2003; Manzello, 2010; Medellin-Maldonado et al., 2016). These comparisons indicate that the growth of the three coral species studied in our study was not affected by La Niña, perhaps because they are acclimated to changes in temperature.
The overall tissue growth area among pocilloporid morphospecies was different, P. cf. capitata and P. cf. verrucosa had the highest superficial growth compared with P. cf. damicornis (Appendix 5). These results evidenced that P. cf. capitata and P. cf. verrucosa could have a greater competitive advantage than P. cf. damicornis in terms of live tissue growth, and perhaps, it can be more considerable to increase live coral cover (Tortolero-Langarica et al., 2017). It is known that the coral growth is sometimes moderated by the need to increase skeletal density mass to withstand hydrodynamic forces and recovery rates (Chindapol et al., 2013; Hughes, 1987; Lirman et al., 2010). The latter is relevant because the water motion and wave exposure effect strongly influences the Isla Isabel area, so P. cf. capitata and P. cf. verrucosa would have greater recovery, and possibly better substrate colonization. Thus, the coral morphology may be an important factor influencing growth rates during coral restoration, depending on physical environmental factors to efficient metabolic pathways (Wild et al., 2005). For example, P. damicornis has more branching and three-dimensional growth shape, while P. capitata and P. verrucosa keep all their energy growing vertically, so this different morphology could affect their growth rates. In other words, some corals grow vertically to find the sun faster, and other corals expand their branches to increase their volume and surface to capture more solar energy. However, depending on the hydrodynamic conditions, the coral colonies also modify their shape; Thus, Pocillopora species could change their morphology in months under different flow regimes (Paz-García et al., 2015).
Similar coral growth was found between natural and artificial substrata types through using three coral morphospecies; However, coral self-attachment was different (personal authors’ observation) may be due to biofilms and crustose coralline algae (CCA) that could induce better coral self-attachment in natural substrate. This pattern coincides with the reported in other restoration studies where natural substrate promotes higher attachment rates over any other substrate (Forrester et al., 2011; Schlancher et al., 2007; Tortolero-Langarica et al., 2014; Yap, 2004). However, the artificial substrate could be an alternative option to incrementing habitat structural complexity, promoting shelter, and improving biodiversity (Schuhmacher et al., 2000; Tortolero-Langarica et al., 2014). In this study, we used artificial hollow hemispheres made with holes on the sides with corals attached to steel-stacks, which enhanced the habitat heterogeneity and favored the coral growth and the coral-associated fauna.
The current context of coral reefs declining worldwide and the use of active coral restoration strategies can mitigate the potential coral ecosystem degradation (Manzello, 2010; Rinkevich, 2015). This work has shown that developing coral reef restoration efforts using Pocillopora coral fragments could be feasible in CMP and potentially effective everywhere in the ETP region. Our results correspond to and support what was found by other studies carried out in many sites in this region. In the CMP, the transplanted Pocillopora fragments’ growth rates are similar to our estimates (Liñán-Cabello et al., 2011; Tortolero-Langarica et al., 2014) in natural (Liñán-Cabello et al., 2011) and artificial substrata (Tortolero-Langarica et al., 2014), as such as under ENSO conditions (Tortolero-Langarica et al., 2017). Therefore, the results of this work, and other studies that have previously been done in many sites of the ETP, allow recommending: i) to start the restoration in the summer season because there were the highest coral growth rates; ii) to use P. cf. capitata and P. cf. verrucosa because these species are more resistant to heat-waves exposure. This information can increase yield and effectiveness during coral restoration programs.
Ethical statement: the authors declare that they all agree with this publication and made significant contributions; that there is no conflict of interest of any kind; and that we followed all pertinent ethical and legal procedures and requirements. All financial sources are fully and clearly stated in the acknowledgements section. A signed document has been filed in the journal archives.