Introduction
Coral reefs are the most diverse ecosystems on Earth in terms of higher taxonomic classification. Coral reefs harbor 32 of the 34 animal phyla, compared to 12 that live in terrestrial environments (Reaka-Kudla, 1997; Stella et al., 2011). The extraordinary biodiversity of coral reefs can be attributed to the structural complexity of the corals and the framework that they construct. This structural complexity corresponds to a great diversification and quantity of ecological niches that, in turn, attract a large diversity of fauna (Stella et al. 2011). The cryptofauna, the animals that live in between the branches of the coral and the reef structure, comprise the greatest number of animal species on a reef but are often overlooked when studying reef ecology (Cortés et al., 2017; Glynn, 2013; Stella et al. 2011). Corals host a variety of cryptic organisms, many of which are symbionts that live exclusively on particular species of corals (Abele & Patton, 1976; Glynn, 1980; Glynn, 1983; Sin, 1999; Stella et al., 2010; Stella et al. 2011). The cryptofauna depend on the corals for protection, food, and reproduction (Abele & Patton, 1976; Castro, 1988; Stella et al., 2011). In return, cryptofauna carry out critical ecological processes within the reef ecosystem such as protecting coral colonies and capturing and recycling nutrients (Glynn, 2013; Glynn & Enochs, 2011; Stella et al., 2011).
A great number of fish species also have an ecological association with, or dependence on, coral reefs for shelter, as direct or indirect food sources, and as sites of reproduction (Froehlich et al., 2021; Moeller et al., 2023). Some fish live in closer association with the corals than others, ranging from cryptic species living within the branches to pelagic species that rely on food sources derived from reefs (Reaka-Kudla, 1997). The reef fish, in turn, provide a variety of critical services to the reefs they inhabit (Burkepile & Hay, 2010; Hixon, 1997; Hixon & Bronstoff, 1996). Herbivore fish and invertebrates graze on algae and reduce its coverage so that the algae do not compete with corals for space and resources (Burkepile & Hay, 2010; Hixon & Bronstoff, 1996; Hughes et al., 2017; Humphries et al., 2014). Invertebrate-eating fish protect the colonies from corallivorous invertebrates, and predatory piscivorous fish maintain healthy balances of reef species by controlling the populations of coral predators and bio-eroding herbivores (Hixon, 1997).
One of the most important contributing factors to supporting cryptofauna is the health of the coral itself. Live corals have greater diversity and abundance of fish than dead corals (Bell & Galzin, 1984). Bleached corals have less abundance and fewer species of cryptic fauna than healthy corals (Tsuchiya, 1999). On dead coral, there is an initial increase in biodiversity as boring invertebrates colonize the exposed carbonate skeleton, which is unprotected by the coral's stinging polyps (Enochs, 2012). This diversity is not sustained, however, as eventually the skeleton is eroded, and with it, the structural complexity sustains the biodiversity of a living coral reef (Enochs & Manzello, 2012a).
Coral restoration is essential to the facilitation and acceleration of natural successional processes through the reintroduction and management of key species (Horoszowski-Fridman & Rinkevich, 2017; Horoszowski-Fridman et al., 2015; Walker, et al., 2007; Young, 2001). Thus, the best sites for coral reef restoration are those sites where corals previously flourished but where they are unlikely to settle and grow under the current conditions (Rinkevich, 2005). Likewise, the best candidates for species to be transplanted are those that might not colonize the restoration area unassisted (Society for Ecological Restoration International Science & Policy Working Group (SER), 2004). Pocillopora coral is a good candidate for reef restoration in that their branched skeletons create conditions that allow other organisms to colonize an area where they previously lacked habitat (Clark & Edwards, 1994; Darling et al., 2012).
Except for a survey of coral borers (Fonseca & Cortés, 1998), no published survey information exists that describes the species of cryptic fauna associated with Pocillopora in Golfo Dulce. However, the coral-associated fauna expected to be present can be predicted from surveys conducted in other reefs in the Eastern Tropical Pacific (ETP) including Bahía Culebra, Costa Rica (Alvarado & Vargas-Castillo, 2012; Salas-Moya et al., 2021), Uva Island in Panama (Abele & Patton, 1976; Glynn, 1980; Glynn, 2013; Gotelli & Abele, 1983; Gotelli et al., 1985), and the Pacific coast of Mexico (Hernández et al., 2009; Hernández et al., 2013). The species composition of ichthyofauna of Golfo Dulce is similar to but statistically different from that of the rest of the Pacific coast of Costa Rica (Alvarado et al., 2014).
The timing of recruitment of the associated fauna onto a coral colony appears to also be of importance for the reef's survival and health. For example, it is known that some cryptic fauna is recruited to corals quickly and begin to reduce the effects of sedimentation and provide protection from predation (Glynn, 2013; Stewart et al., 2006). This is particularly important for juvenile corals compared to larger, more mature corals (Toh et al., 2014). In laboratory settings, juvenile host corals have higher survival rates when colonized with juvenile Trapezia crabs, which offer the host coral protection from predation (Rouzé et al., 2014). However, it is not known when and in what order cryptic fauna are recruited in coral transplants.
Although non-coral animals such as fish and invertebrates make up the majority of a reef's biomass and diversity (Cortés et al., 2017; Stella et al., 2011), most studies addressing the success of a restoration effort focus on the growth and survival of the corals themselves (Ladd et al., 2019; Stella et al., 2011). While the corals are critical to forming the basic structure of coral reefs that allows them to harbor such great biodiversity, the effect of reef restoration on the community structure of coral-associated animals is relatively understudied by comparison. Most of the available literature on the succession of fish following restoration efforts focuses on non-living artificial reefs (Becker et al., 2017; Russell, 1975; Santos et al., 2011). Similarly, studies concerning the succession or community structure of cryptic fauna on reefs have used non-coral substrates (Breitburg, 1985) or the collection and destruction of coral colonies (Enochs & Hockensmith, 2008; Enochs & Manzello, 2012b; Enochs et al., 2011).
Given the importance of reef-associated animals to the health and survival of the coral, the ecosystem services provided by the associates, and the wide-ranging impacts of reef communities on the ecology of the oceans, more research on the effects of reef restoration and rehabilitation on coral-associated animals is essential. Ecological restoration and rehabilitation are considered to be successful when they can regain the full complement of native species and recuperate ecological processes essential for the long-term persistence and self-sustainability of the reef (SER, 2004; Walker et al., 2007).
To understand the processes of the succession of the cryptofauna and fishes in a coral reef of the ETP, this project aims to: 1) Describe the community of cryptofauna living in the coral transplants; 2) monitor changes in the community of cryptofauna in Pocillopora colonies in the 8 months following transplantation; and 3) explore changes in the reef fish community following transplantation.
Materials and methods
Golfo Dulce is a narrow-mouthed embayment (8° 27'-8° 45'N, 83° 07'-83° 30'W) oriented northwest to southeast between the Osa Peninsula and the southern Pacific coast of Costa Rica (Fig. 1). The Gulf has an approximate length of 50 km and width between 10 and 15 km, and covers an area of 680 km2 with a maximum depth of 200 m. The average yearly rainfall in Golfo Dulce is 4 000 to 5 000 mm and temperatures range from 18 ºC to 35 ºC with an average of approximately 26.5 ºC. The dry season lasts from December to March with an average rainfall of 100 to 160 mm per month. The wet season peaks in October with an average monthly rainfall of 800 mm (Cortés, 1990; Quesada-Alpízar & Cortés, 2006). For this study, data collection months were grouped by ''seasons” that roughly correspond to seasonal ranges in Golfo Dulce as described by Cortés (1990). The ''Wet” season includes September, October, November, & December, the ''Dry” season includes January, February, and March, and the ''Transition” season includes April, May, June, July, & August. During the period of this study (July 2019 - June 2020), there was a total rainfall of 3 687 mm based on meteorological data from the Fundación Neotrópica station of the Instituto Meteorológico Nacional, near Golfo Dulce (08º 42'02.9” N, 83º 30'49.4” W, at 80 m above sea level) (Fig. 2). The area-averaged daytime sea surface temperatures during the study period ranged from 29.3 ºC in October to 31.4 ºC in February based on the NASA GIOVANNI satellite (Fig. 3).
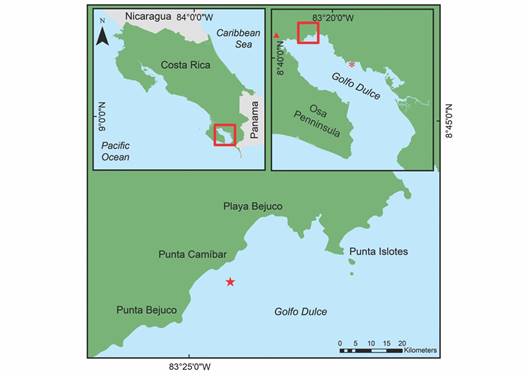
Fig. 1 Location of Golfo Dulce within Costa Rica: Punta Bejuco (transplantation site) is marked with a red star and Nicuesa (coral nursery), is marked with a red asterisk. The red triangle in the top-right quadrant marks the meteorological station.
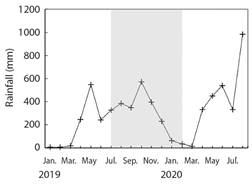
Fig. 2 Rainfall (mm month-1) data from the Instituto Meteorológico Nacional de Costa Rica, near Golfo Dulce (08º42'03”N, 83º30'49”W, at 80 m above sea level). The gray shaded area represents the timeframe of the study.
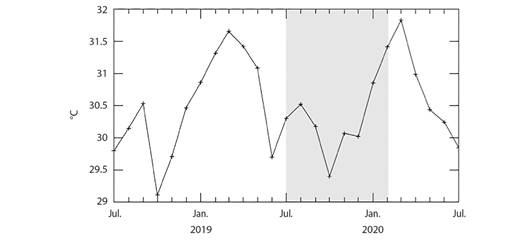
Fig. 3 Area-averaged daytime sea surface temperatures (ºC) from the northern tip of Golfo Dulce from July 2018 to July 2020. The timeframe of the experiment is shaded in gray. Data from the NASA GIOVANNI satellite.
Timeframe: The experiment took place between July 2019 and February 2020. Twelve months of data collection were planned, but due to the COVID-19 global pandemic, field trips were terminated in February 2020, resulting in eight months of data.
Geographically and ecologically, Golfo Dulce is part of the ETP marine biological province (Glynn et al., 2017). It is often referred to as a tropical fjord due to its anoxic deep waters and bathymetry (Cortés, 1990; Hebbeln & Cortés, 2001; Wolff et al., 1996). The anoxic waters prevent the energy contained in detritus that sinks to the depth of the gulf to be recycled back into higher trophic levels of the ecosystem (Wolff et al., 1996).
Experimental coral colonies: Thirty Pocillopora damicornis (Linnaeus, 1758) and Porites evermannii Vaughan, 1907 colonies were selected at random from a crop of colonies grown in the coral tree nurseries in Nicuesa, Costa Rica (Fig. 1). These nurseries form part of a restoration initiative that started in 2016 as a project of the non-governmental organization Raising Coral Costa Rica and the Center for Research in Marine Science and Limnology (CIMAR) of the University of Costa Rica (UCR) (Kleypas et al., 2021; Villalobos, 2019). The two species were not distinguished for this study and are referred to as ''Pocillopora” for the rest of this article.
Transplantation site: Punta Bejuco reef (8° 43'39” N, 83° 24'30” W) (Fig. 1) was selected as the transplantation site for the Pocillopora colonies. Punta Bejuco consists of three reefs with steeply sloping edges separated by channels of sand. The reef substrates are mostly made up of dead Pocillopora and Psammocora rubble. The live coral species Porites lobata Dana, 1846 and P. evermanni are predominant in this site (Boulay et al., 2014; Cortés, 1990). No live colonies of Pocillopora have presently been encountered in Punta Bejuco, but the presence of live Pocillopora in the past can be inferred from coral skeletons (Cortés, 1990). Pocillopora corals have low rates of natural recruitment in Golfo Dulce (Villalobos, 2019), and small colonies are more likely to die as a result of predation (Toh et al., 2014) and competition with algae (Kuffner et al., 2006).
Out-planting of nursery-grown Pocillopora colonies and experimental design: The colonies were anchored with nylon zip-ties to steel nails, and driven into the calcium carbonate substrate in a six by five rectangular arrangement of the colonies with a distance of 30 cm from neighboring colonies. The rectangular transect extended 15 cm from the centroids of the fringing nails to measure 1.5 by 1.8 m in total (Fig. 4). To understand how the presence of the transplanted coral affects the fish and benthic invertebrate communities and the sea floor cover, a control transect was designated and monitored using the same methods as the transplantation site, e.g., with 30 steel nails but devoid of Pocillopora outplants (Fig. 4). The control transect is located at Punta Bejuco at the same depth as the transplant area in a relatively flat region with a (visually) similar substrate composition.
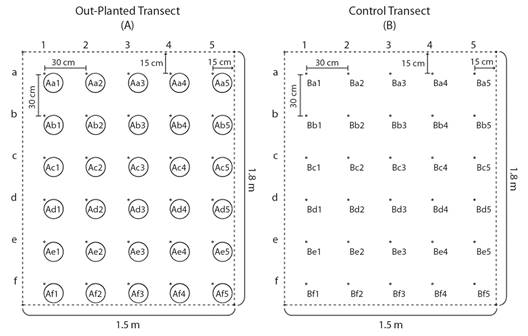
Fig. 4 The arrangement of experimental colonies and nails within transects on the sea floor. The black dots represent nails used as attachment points for the colonies (left) or as controls (right). The circles represent Pocillopora colonies. The dotted line represents the edge of the experimental and control patches. Each colony or nail position is given a procedural name so that it can be easily located.
Monitoring of cryptic fauna: In the transplanted transect, the cryptofauna in each colony were identified and counted by visual census every month. The observer identified and recorded all of the visible cryptofauna occupying the space between the branches or on the surface of each colony. The observer positioned themselves about 3 to 10 cm from each colony and viewed it from every angle to ensure that they recorded all cryptofauna therein before moving to the next colony. Most methods used to investigate the cryptofauna living between the branches of corals require the corals to be removed from the study site and destroyed (Abele & Patton, 1976; Alvarado & Vargas-Castillo, 2012; Enochs & Hockensmith, 2008; Enochs & Manzello, 2012a; Enochs & Manzello, 2012b; Stella et al., 2010). In situ visual identification provides a low-cost, non-destructive method for the quantification of the cryptic community over time, although it is not as effective as a destructive collection in the identification of very small and burrowing cryptic species (Alzate et al., 2014; Caldwell et al., 2016) which were not the focus of this study.
Monitoring of ichthyofauna communities in transplanted and control transects: To identify and count the reef-associated fish in either patch, each month a diver observed ichthyofauna from a fixed position approximately 2 m away from the deepest border of the out-planted transect and the control transect. For 10 minutes on each experimental patch, the observer identified and counted fish within an imaginary rectangular prism extending the length and width of each transect and 3 m above the sea floor (Fig. 5). The icthyofauna communities associated with the Pocillopora outplants and control transect were summarized by three measurements: Shannon-Weiner diversity index (H'= -Sp i ln(p i)), total abundance of fish, and the specific abundance of each species.
Data analysis
Cryptofaunal community: The diversity and abundance of cryptic organisms are described in terms of operational taxonomic units (OTUs) instead of species according to Enochs & Manzello (2012b), to prevent overestimation. The cryptic faunal community associated with the Pocillopora outplants is summarized in terms of the number of OTUs, the total abundance of cryptic organisms, and the specific abundance of each OTU in each colony and within the experimental patch as a whole. The diversity of the cryptofauna was defined as the number of unique OTUs present in each colony.
Generalized linear models (GLMs) in Poisson distribution were used to identify trends in both abundance and diversity over time in the eight months following transplantation. To explore changes in the cryptofaunal community living between the branches of the transplanted Pocillopora colonies, the similarity of community composition in terms of the presence and abundance of cryptofauna OTUs between and across months and seasons (dry, transition, and rainy) was quantified with cluster analysis performed in PRIMER 7 (Clarke & Gorley, 2015). First, the average abundance of each cryptic species each month was square root transformed to enhance the impact of less common OTUs and to diminish that of the most common OTUs. The transformed data were then used to build a Bray-Curtis similarity matrix (Clarke & Gorley, 2015). This similarity matrix was used to represent the similarity of the cryptic community each month in two (2) dimensions using non-metric multidimensional scaling (nMDS) (Clarke & Gorley, 2015) and to build a dendrogram showing the similarity of the cryptic community of the transplanted colonies between sample months. Additionally, the data were used to generate a heat plot that displays the contribution of each OTU to the cryptic community each month.
To test for significant differences in the cryptic community between seasonal groupings of months in terms of the average abundance of OTUs, an analysis of similarity (ANOSIM) was conducted using the Bray-Curtis similarity matrix. To determine which OTUs contributed most to any dissimilarity in the cryptofaunal community amongst groupings, similarity percentage analysis (SIMPER) was conducted using the Bray-Curtis similarity matrix in PRIMER 7 (Clarke & Gorley, 2015).
Ichthyofaunal community: Student's t-tests were performed in R to detect differences between the experimental and control transects in the abundance (number of individuals per patch) and Shannon-Weiner diversity index (H') of the ichthyofauna over the eight months of data collection. The ordination method of nMDS was performed in PRIMER 7 (Clarke & Gorley, 2015) to explore differences in the reef fish community between the experimental and control patches. The abundances of fish species each month were square root transformed to enhance the impact of less common species and to diminish that of the most common species. The transformed data were then used to build a Bray-Curtis similarity matrix (Clarke & Gorley, 2015). This similarity matrix was used to represent the similarity of the fish community each month in two dimensions using non-metric multidimensional scaling (nMDS) (Clarke & Gorley, 2015). Differences in the reef fish community between experimental and control patches were explored with ANOSIM and SIMPER tests in PRIMER 7(Clarke & Gorley, 2015).
Results
Description of the cryptofaunal community: Over the eight months of the study, a total of 17 OTUs of cryptofauna were identified and counted (Table 1). These OTUs were split into four categories based on their taxonomy and ecological role in the reef ecosystem: (1) decapod crustaceans, (2) cryptic fish that permanently occupied the spaces between the coral branches, (3) reef fish that are usually encountered in the water column around corals, and (4) encrusting animals attached to the coral transplants. The decapod crustaceans included Trapezia spp., Alpheus lottini, Harpiliopsis depressa, Palaemonella holmesi, Palaemon ritteri, the family Paguridae, and the superfamily Majoidea. All of the cryptic fish were within the order Blenniiformes, and included Axoclinus lucilae, Acanthemblemaria exilispinus, Elacatinus punticulatus, Plagiotremus azaleus, and individuals that were not identified at the species level within the family Tripterygiidae. Reef fish included Abudefduf troschelli, Scarus spp., Stegastes acapulcoensis, and fish within the family Chaetodontidae. The encrusting metazoan group included polychaetes within the family Sabellidae, ascidians, gastropods within the family Vermetidae, and bivalves within the genus Spondylus.
Patterns in the community of cryptofauna in Pocillopora colonies following transplantation: The dominant cryptofauna throughout the experiment were symbiont decapods in the OTUs Trapezia spp., Harpiliopsis depressa, and Alpheus lottini. They comprised on average 81 % (43 %, 30 %, and 18 % respectively) of the fauna identified within the 30 colonies over the eight months of monitoring (Table 1). The rest of the cryptofauna identified within the colonies were cryptic fish, transient fish, and encrusting metazoans, accounting for 19 % of the associated fauna found between the branches of the Pocillopora transplants.
Table 1 Counts and percentages of cryptofauna OTUs identified within the transplanted corals each month following transplantation at Punta Bejuco, Golfo Dulce.
Group | O T U (symbol) | Jul19 | Aug19 | Sep19 | Oct19 | Nov19 | Dec19 | Jan20 | Feb20 | ||||||||||
n | % | n | % | n | % | n | % | n | % | n | % | n | % | n | % | ||||
Decapod | Trapezia spp. * (tra) | 168 | 54.7 | 117 | 50.9 | 153 | 45.0 | 128 | 30.8 | 153 | 49.7 | 181 | 51.9 | 252 | 37.9 | 250 | 38.9 | ||
Decapod | Harpiliopsis depressa* (hde) | 103 | 33.6 | 73 | 31.7 | 83 | 24.4 | 183 | 44.0 | 79 | 25.6 | 50 | 14.3 | 238 | 35.8 | 157 | 24.4 | ||
Decapod | Alpheus lottini (alo) | 24 | 7.8 | 38 | 16.5 | 90 | 26.5 | 89 | 21.4 | 46 | 14.9 | 102 | 29.2 | 119 | 17.9 | 91 | 14.2 | ||
Encruster | Spondylus sp. (spo) | 8 | 2.6 | ||||||||||||||||
Decapod | Palaemonella holmesi (pho) | 4 | 1.3 | 46 | 7.2 | ||||||||||||||
Decapod | Majoidea (maj) | 2 | 0.9 | ||||||||||||||||
Cryptic fish | Blenniiformes (blenny) | 4 | 1.2 | 4 | 1.0 | 18 | 2.7 | 2 | 0.3 | ||||||||||
Decapod | Paguridae (pagu) | 4 | 1.2 | 8 | 1.9 | 10 | 3.2 | 4 | 1.1 | 10 | 1.5 | 8 | 1.2 | ||||||
Encruster | Vermetidae (verm) | 2 | 0.6 | 2 | 0.5 | 10 | 3.2 | 4 | 1.1 | 2 | 0.3 | ||||||||
Encruster | Ascidea (asci) | 2 | 0.5 | 2 | 0.6 | 6 | 1.7 | ||||||||||||
Encruster | Sabellidae (sab) | 8 | 2.6 | 2 | 0.6 | ||||||||||||||
Reef fish | Scarus spp. (scar) | 24 | 3.6 | 27 | 4.2 | ||||||||||||||
Reef fish | Stegastes acapulcoensis (sac) | 4 | 0.6 | 8 | 1.2 | ||||||||||||||
Decapod | Palemon ritteri (pri) | 42 | 6.5 | ||||||||||||||||
Reef fish | Chaetodontidae (chaet) | 8 | 1.2 | ||||||||||||||||
Reef fish | Abudefduf troschelli (atr) | 2 | 0.3 | ||||||||||||||||
TOTAL Abundance | 307 | 100 | 230 | 100 | 340 | 100 | 416 | 100 | 308 | 100 | 349 | 100 | 665 | 100 | 643 | 100 | |||
Total OTUs | 5 | 4 | 6 | 7 | 7 | 7 | 7 | 12 |
Symbols in parentheses are used in Fig. 8. Asterisks (*) indicate OTUs that are obligate symbionts of Pocillopora spp. OTUs are arranged by order of first observ ation and percent contribution to the cryptofaunal community each month.
Abundance: In July 2019, the day following the transplantation of the colonies, an average of 10 individual cryptic organisms were observed per colony (ind colony-1). In February 2020, an average of 21 ind colony-1 were observed. Based on the GLM analysis, the abundance of cryptofauna living within the transplanted coral colonies increased significantly with time (P < 0.001, df = 7) over the eight months following transplantation (Fig. 6).
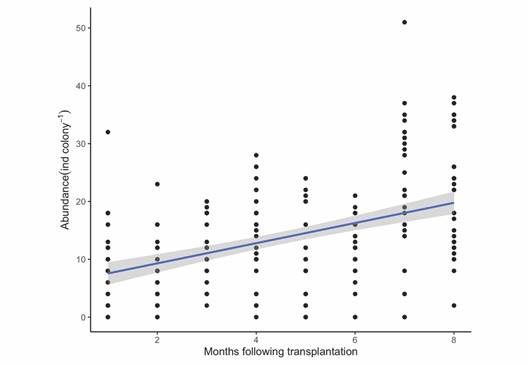
Fig. 6 Number of individuals of cryptofauna living within the branches of each Pocillopora colony (ind colony-1) over the eight months of data collection after transplantation. The blue line is a generalized linear model of the data based on Poisson distribution. The gray area around the line represents the 95 % confidence interval.
Diversity: The diversity of cryptofauna within the transplanted coral colonies increased significantly with time (P < 0.01, df = 230) in the eight months following transplantation (Table 1). In the entire experimental patch, five OTUs were present at the initial observation and increased to 12 OTUs by the end of the eight months of data collection (Table 2).
Table 2 SIMPER table showing the similarity/dissimilarity within and between seasons and the percentage difference the most significant OTUs contribute.
Season | % Similarity/Dissimilarity | OTU | % Contribution |
Dry | 82.3 % Similar | Scarus spp. | 37.9 |
Trapezia spp. | 22.2 | ||
Harpilliopsis depressa | 17.6 | ||
Transition | 85.3 % Similar | Trapezia spp. | 45.8 |
Harpilliopsis depressa | 36.2 | ||
Wet | 84.3 % Similar | Trapezia spp. | 35.4 |
Harpilliopsis depressa | 25.1 | ||
Alpheus lottini | 24.9 | ||
Dry & Transition | 46.1 % Dissimilar | Scarus spp. | 38.7 |
Trapezia spp. | 15.7 | ||
Harpilliopsis depressa | 10.1 | ||
Palaemonella holmesi | 4.7 | ||
Palemon ritteri | 4.4 | ||
Dry & Wet | 44.2 % Dissimilar | Scarus spp. | 38.1 |
Trapezia spp. | 12.2 | ||
Alpheus lottini | 10.4 | ||
Harpilliopsis depressa | 7.4 | ||
Vermetidae | 4.7 | ||
Transition & Wet | 23.6 % Dissimilar | Alpheus lottini | 15.2 |
Paguridae | 14.6 | ||
Vermetidae | 11.8 | ||
Harpilliopsis depressa | 11.6 | ||
Spondylus sp. | 8.7 | ||
Ascidea | 7.6 | ||
Trapezia spp. | 7.1 |
Monthly change in cryptofaunal community composition: Non-metric multidimensional scaling (nMDS) (Fig. 7) and the corresponding cluster analysis dendrogram (Fig. 8) showed three distinct groupings of average monthly cryptofauna community compositions (> 80 % similarity within groups) corresponding to seasonal groupings ''Wet”, ''Transitional”, and ''Dry”. Analysis of similarity (ANOSIM) was used to detect differences between these groups. The groups were shown to be well separated (R statistic) but not significantly different (p statistic) in composition (global R = 0.988, global P = 0.05; Dry vs. Trans.: R = 1, P = 0.33; Dry vs. Wet: R = 1, P = 0.07; Trans. vs. Wet: R = 0.96, P = 0.07). Results of similarity percentage analysis (SIMPER) show that the three groups differ more from each other than within each group (Table 2).
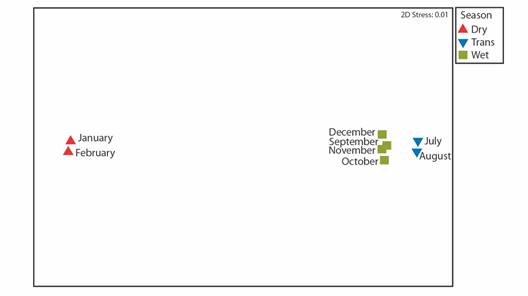
Fig. 7 Non-metric multidimensional scaling of the composition of cryptofauna within the transplanted Pocillopora colonies in the experimental patch, Punta Bejuco, Golfo Dulce for each month. nMDS based on Bray-Curtis similarity matrices built on square-root transformed monthly average abundances of cryptofauna OTUs and by the ''season” as a factor.
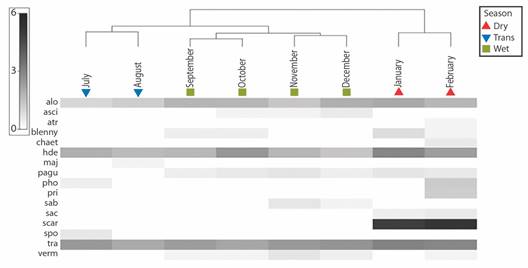
Fig. 8 Average union dendrogram showing the similarity of the cryptofaunal community of the Pocillopora transplants between months in the experimental patch, Punta Bejuco, Golfo Dulce. Months are arranged chronologically from left to right and grouped by the factor season. Below the dendrogram is a heatplot showing the square-root transformed abundances (ind colony -1) of cryptofauna each month. OTU symbols are assigned in Table 1.
Changes in the reef fish community following transplantation: Although there are trends toward greater abundance and diversity of reef fish in the experimental compared to the control patch, these trends were not statistically significant: Abundance (Student's t-test: Df = 11.72, P > 0.05) (Fig. 9A) and Shannon diversity (Student's t-test: df = 13.03, P > 0.05) (Fig. 9B). Twenty-two and 17 species were observed in the experimental and control patches respectively. The average numbers of individual fish observed each month were 44.9 and 33.1 in the experimental and control patches, respectively (Table 3). The species with the greatest number of observations was Stegastes acapulcoensis. The ichthyofaunal community did not differ significantly between the control and experimental patches based on the presence and abundance of fish species during the monitoring time (ANOSIM R = 0.143, P > 0.05) (Fig. 10).
Table 3 Species observed and average monthly observations in the experimental and control patches following transplantation.
Species | Ecological role | Average observations per month | ||
Experimental | Control | |||
Stegastes acapulcoensis | Detritivore | 10.6 | 14.0 | |
Stegastes flavilatus | Detritivore | 1.5 | 0.1 | |
Anisotremus taeniatus | Invertebrate eater | 0.0 | 0.1 | |
Arothron hispidus | Invertebrate eater | 0.3 | 0.1 | |
Bodianus diplotaenia | Invertebrate eater | 0.0 | 0.8 | |
Canthigaster punctatissima | Invertebrate eater | 1.9 | 0.8 | |
Gnathanodon speciosus | Invertebrate eater | 1.5 | 0.0 | |
Haemulon steindachneri | Invertebrate eater | 1.8 | 0.0 | |
Halichoeres dispilus | Invertebrate eater | 2.3 | 6.0 | |
Halichoeres nicholsi | Invertebrate eater | 0.1 | 0.0 | |
Johnrandallia nigrirostris | Invertebrate eater | 0.1 | 0.0 | |
Pomacanthus zonipectus | Invertebrate eater | 0.1 | 0.1 | |
Sphoeroides lobatus | Invertebrate eater | 0.8 | 0.8 | |
Thalassoma lucasanum | Invertebrate eater | 2.9 | 1.8 | |
Kyphosus elegans | Macroalgae feeder | 0.1 | 0.0 | |
Scarus compressus | Macroalgae feeder | 0.3 | 0.8 | |
Scarus ghobban | Macroalgae feeder | 6.6 | 3.0 | |
Chaetodon humeralis | Omnivore | 0.1 | 0.0 | |
Kyphosus ocyurus | Omnivore | 0.8 | 0.0 | |
Cephalopholis panamensis | Piscivore | 1.9 | 0.3 | |
Haemulon maculicauda | Piscivore | 3.4 | 2.9 | |
Haemulon sexfasciatum | Piscivore | 0.1 | 0.0 | |
Lutjanus argentiventris | Piscivore | 3.9 | 0.4 | |
Lutjanus novemfasciatus | Piscivore | 0.0 | 0.5 | |
Abudefduf troschelii | Planktivore | 4.0 | 0.9 | |
Total | 44.9 | 33.1 |
The gray shading highlights which species were observed in greater abundance in each patch.
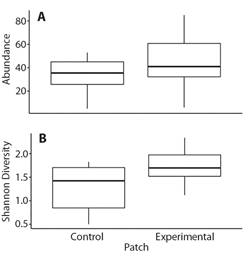
Fig. 9 A. Average abundance and B. average Shannon diversity (H'= -Sp i ln(p i)) of fish in experimental and control patches throughout the experiment.
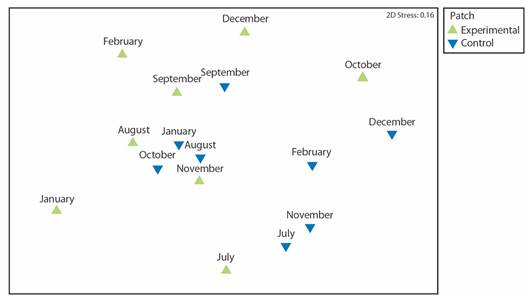
Fig. 10 Non-metric multidimensional scaling of the composition of the fish community within the experimental and control patches at Punta Bejuco, Golfo Dulce each month based on Bray-Curtis similarity matrices built on square-root transformed monthly averages of fish species abundances. The experimental and control patches are indicated with different symbols and point colors.
Discussion
Over the eight months following transplantation to the restoration site, the cryptofaunal community was dominated by obligate decapod symbionts: Trapezia spp., Harpiliopsis depressa, and Alpheus lottini. This is consistent with the hypothesis that these species only live within Pocillopora (Abele & Patton 1976; Castro, 1988; Glynn, 1980; Glynn, 1983; Glynn, 2013; Glynn & Enochs, 2011; Stella et al., 2011) and maintain a constant presence independent of the season (Gotelli et al., 1985). Likewise, the Pocillopora colonies require the symbionts to survive and grow (Glynn, 1983; Glynn, 2013; Stella et al., 2011). The abundance of cryptofauna more than doubled over the observational period, from an average of 10 individuals in each of the 30 transplanted corals on the day after transplantation to 21 individuals eight months later. The diversity of the experimental patch also increased significantly, with five OTUs in the first month and 12 OTUs in the final month.
The cryptofaunal community observed at the study site reflects the known ecology of reef fauna reported in the literature. Decapod crustaceans are the most abundant and diverse cryptic symbionts (Glynn, 2013; Reaka-Kudla et al. 1997; Stella et al. 2011). The brachyuran crabs of the genus Trapezia and snapping shrimp of the genus Alpheus are common decapod symbionts associated with the highly branching coral Pocillopora. The decapods defend their host coral from coralivorous invertebrates like the sea star Acanthaster plancii (Glynn, 1983; Glynn, 2013; Pratchett, 2001) or the snail Drupella (McKeon & Moore, 2014; Samsuri et al., 2018) by snapping at intruders. Symbiotic decapods reduce coral mortality due to sedimentation (Stewart et al., 2006; Stier et al., 2012) and disease (Pollock et al., 2013) by actively ''kicking” and ''shoveling” unwanted particles from Pocillopora colonies, thus reducing stress on the coral (Stewart et al., 2006). The presence of Trapezia crabs induces the Pocillopora host to produce nutrient-rich mucus with fat globules to encourage the mutualism between host coral and crab (Glynn, 1983; Stimson, 1990).
The change in the diversity of the fauna living within Pocillopora colonies over time appeared to represent different recruitment periods of the ecological groups (Table 2). The initial recruits were the obligate symbiont decapods that were recruited to the colonies while they grew in the coral nurseries located about 18 km from Punta Bejuco (personal observation) and remained present following transplantation. The next recruits, first observed three months post-transplantation (September 2019), were blennies and other cryptic fish, followed by encrusting animals. Others have observed that as branching coral colonies grow, the tissue on the bases of the branches begins to die and sessile encrusting and boring animals can colonize the exposed carbonate skeleton (Abele & Patton, 1976; Enochs & Manzello, 2012a). The final group of animals observed to be recruited to the transplanted colonies were juveniles of larger reef fish in January 2020.
The increase in abundance and diversity of cryptofauna over time could be explained by multiple processes. The first regards the growth of the coral. As corals grow, more space is available between their branches to harbor symbionts, thus reducing competition for space and allowing fauna abundance to increase over time with the size of the coral colonies (Abele & Patton, 1976). However, intense and complex inter- and intraspecific aggressive interactions in the common Pocillopora symbionts Trapezia and Alpheus keep the diversity of the obligate symbionts constant and prevent other organisms from being recruited (Castro, 1996; Huber, 1987; Stier et al., 2012). This could explain why for the experiment, obligate symbionts in just three OTUs comprised 81 % of the total number of individuals that occupied the experimental coral transplants.
Other explanations could, as implied by the seasonal differences in cryptofaunal community compositions, be the result of seasonal changes in the environment. In the ETP, the recruitment of associated fauna is greater in the warmer months due to the increased metabolic rate and reproduction of invertebrates (Rodríguez-Troncoso et al., 2018). The environmental factor that most distinguishes the seasons in Golfo Dulce is precipitation (Cortés, 1990; Quesada-Alpízar & Cortés, 2006), leading to greater sedimentation in the gulf during the rainy season. Sedimentation has physiological consequences for the coral such as mucus production and necrosis that could have effects on the associated fauna (Fabricius, 2005).
Although not significantly different, the results of the nMDS, cluster analysis, and SIMPER (Fig. 10) suggest that the cryptic communities were similar between months that roughly correspond to commonly held ranges of the three seasons in Costa Rica, as defined by the amount of precipitation of that period of the year (Table 1). Alvarado & Vargas-Castillo (2012) found that the diversity of cryptofauna in Pocillopora colonies in Bahia Culebra is greater in the dry season than in the wet season. This trend was also observed in this study. Although, due to the timeframe of the study which only included one dry and one wet season, it is unclear whether the upward trend would continue in the next seasonal cycle.
Ecological succession of species through facilitation could be another explanation for the increase in diversity and the sequential appearance of different groupings of fauna following the transplantation of the Pocillopora colonies. Although this study examines succession only insofar as to describe the order of appearance of different species, the mechanisms behind these patterns can be speculated. The presence of cryptofauna, for example, may attract larger species looking to feed on small invertebrates living within the coral whom themselves bring symbionts and nutrients to the growing ecosystem.
As expected, greater diversity and abundance of fish were observed in the experimental patch containing colonies rather than the control patch without colonies although the differences were not statistically significant. Fish are attracted to structural complexity because it provides numerous microhabitats that coincide with a greater diversity of prey animals and shelter whether it is a live reef (Bell & Galzin, 1984) or an artificial reef (Becker et al., 2017; Russell, 1975; Santos et al., 2011). Herbivorous fish control the population of leafy and fleshy algae that can impede the settlement and growth of corals (Burkepile & Hay, 2010; Hixon & Bronstoff, 1996; Humphries et al., 2014). There may be species-specific effects of different herbivores on different types of algae, meaning that fish diversity is important for the health of reefs (Burkepile & Hay, 2010).
The cryptofaunal community in this study behaved differently in some respects when compared to descriptions in the literature. For example, intraspecific competition normally limits species in the genera Alpheus and Trapezia to two individuals of the same species per colony, a male and female reproductive pair (Castro, 1996; Huber, 1987; Stier et al., 2012). In this study, we observed an average of 5.8 Trapezia individuals per colony, and 2.5 Alpheus individuals per colony. This could be explained by the limited population of Pocillopora in Golfo Dulce resulting in fewer places for competing for decapod symbionts to move (Castro, 1978).
The data collected for this project was used to add to our understanding of the interrelationships between associated fauna and corals, the patterns of biodiversity, and ecological succession in Pocillopora transplants in the restoration project in Golfo Dulce, South Pacific, Costa Rica. The results may be useful for helping to plan future restoration projects and to guide expectations regarding the magnitude of the number and variety of cryptic organisms in the immediate aftermath of coral transplantation and the expected timing of the recruitment of associated fauna. Multiyear studies that include extensive environmental monitoring are required to tease out the relative contribution of the environment, interspecies interactions, and the other multiple contributions to the complex reef colony. This type of understanding of coral reef ecosystems is one of the most critical ways to combat the ecological crisis of coral decline (Bellwood et al., 2004).
Ethical statement: the authors declare that they all agree with this publication and made significant contributions; that there is no conflict of interest of any kind; and that we followed all pertinent ethical and legal procedures and requirements. All financial sources are fully and clearly stated in the acknowledgements section. A signed document has been filed in the journal archives.