Introduction
Agricultural production accompanying population growth has increased worldwide since the beginning of the 20th century (Carvalho, 2017). Such increasing was possible due to monoculture practices of genetically modified species, a technology that led to using multiple phytosanitary products (Bindraban et al., 2009). The frequent use of pesticides is currently considered a cause of environmental pollution due to the high entrance rates these substances into agroecosystems. Although these products were designed to have a toxic effect at low concentrations on target organisms, many of them have high toxicity on non-target organisms, and they also have potential for bioaccumulation and persistence in different environmental compartments such as soil, water and sediment (Evenson & Gollin, 2003; Primost et al., 2017). This situation constitutes a risk for the species that inhabit these ecosystems, in particular anurans that have characteristics in their modes of life that make them prone to exposure and interaction with environmental pollutants (Agostini et al., 2013; Brodeur et al., 2011; Natale et al., 2018; Pérez-Iglesias et al., 2015). Furthermore, the low migratory capacity of anurans (Sinsch, 1990) makes them good indicators of the environment they live (Brodeur & Vera-Candioti, 2017; Blaustein & Wake, 1995; Guzy et al., 2012).
For more than two decades studies have detected a decrease of amphibian populations at a global level (Croteau et al., 2008; Collins et al., 2009; Houlahan et al., 2000; Sparling et al., 2010; Young et al., 2004). Several factors have been proposed as possible causes for this phenomenon, such as destruction, fragmentation, and pollution of the habitat caused mainly by the advance of the agricultural frontier, and by intensive agricultural practices (crops under cover) and extensive agricultural practices of monocultures (Beebee & Griffiths, 2005; Davidson et al., 2002).
Environmental levels of pesticides can generate sublethal effects from short-term (acute) and long-term (chronic) exposures. These effects can be evaluated with biomarkers at different levels such as biochemical, physiological, histological, and morphological (Agostini et al., 2013; Brodeur et al., 2011; Lajmanovich et al., 2011; Natale et al., 2018; Pérez-Iglesias et al., 2015; Venturino et al., 2003). Effects that occur at higher levels usually have a greater ecological relevance (Baird et al., 2007) as may do the occurrence of morphological abnormalities, which are expressed at an organism level, but could have population-level effects (Johnson et al., 2001). Most of the studies that evaluated the occurrence of abnormalities were performed in the laboratory because of exposure to pesticides, detecting abnormalities especially during the embryonic and larval stages (Aronzon et al., 2011; Brunelli et al., 2009; Boccioni et al., 2020). Field studies of abnormalities are scarce and are mainly focused on adult anurans (Hopkins et al., 2000; Peltzer et al., 2011; Silva & Toledo, 2010). Henle and Dubois (2017) synthesize the information on abnormalities in the external morphology based on anecdotal observations of a few individuals. Reeves et al. (2008), Lunde and Johnson (2012), and Anzaldua and Goldberg (2019) report the presence of abnormal individuals in large numbers and percentages, generally from areas called ''hotspot'', which, according to Lannoo (2008) correspond to areas with a prevalence of abnormalities equal to or greater than 5 %.
Within the framework of a long-term monitoring project of anurans in Northeastern Buenos Aires province (La Plata and its surroundings) that started in 1996, our team observed a high frequency of severe abnormalities in the early larval stages of the common toad Rhinella arenarum (Hensel, 1867) that inhabited ponds located in agroecosystems. At present, there are few histopathology reports associated with abnormalities in anuran larvae. The objective of the present work is to report the occurrence of abnormalities in larvae of the common toad in agroecosystems by description through macroscopic and histopathological analysis.
Materials and methods
In two consecutives springs (2015 and 2016), reproductive events of the common toad were monitored in two temporary pond systems located near the city of La Plata (Buenos Aires, Argentina) (Fig. 1A). The first pond (P1, Fig. 1B) is in an area without agriculture (34°59'4.74'' S, 57°51'21.98'' W), dominated by grasses and surrounded by renewals of native Celtis tala (Gillies ex Planch, 1848) trees and Cyperus spp. (Linnaeus, 1753). The other pond (P2, Fig. 1C, Fig. 1D), is in an area with intensive agriculture and floriculture around the headwaters of Carnaval stream (34°55'11.67'' S, 58° 6'39.00'' W). All of them are in low areas that collect rainwater and can be dried out.
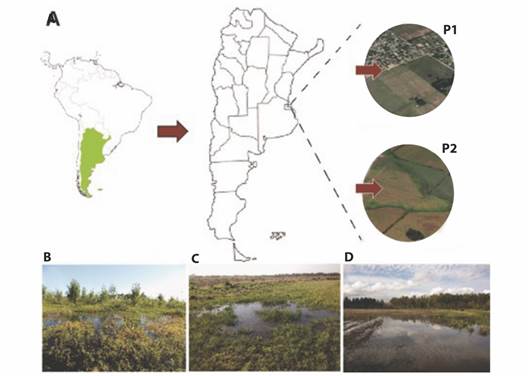
Fig. 1 Sampling sites for Rhinella arenarum population. A. Location of both temporary ponds near of La Plata city (Buenos Aires, Argentina), P1: non-agriculture area, P2: agriculture area. B. Photography of pond without agricultural activity. C.-D. Photography's of ponds surrounded by cultivated areas.
The determination of the physicochemical parameters (temperature, conductivity, dissolved oxygen, and pH) was carried out in situ at the moment in which the tadpoles were found with a WA-2017SDu multiparametric.
Diurnal and nocturnal visual encounter studies (Heyer, 1994) of R. arenarum were conducted to verify presence and reproduction of the species at each site. The reproductive sites were located by auditory censuses during the surveys of the study areas. Once the reproductive sites were detected, stage of each reproductive event was recorded, such as the numbers of adult toads, amplexus and clutches. Larvae were anesthetized with MS 222, fixed in 10 % formaldehyde, and carried out to the laboratory. Subsequently, each larva was photographed and the body length in mm was recorded, using version 1.46r of ImageJ® program (Rasband, 1997), and the stage of development was determinate according to Gosner (1960). In addition, they were examined macroscopically to record possible anomalies using the atlas of abnormalities proposed by Bantle (1991). All procedures were performed under an Olympus CX31 stereomicroscope.
In addition, representative specimens (both normal and abnormal) of each studied pond were processed for histopathological analysis. The fixed larvae were routinely processed and embedded in paraffin wax (Plaul et al., 2017). Histological sections were obtained using a sledge microtome, prepared according to standard protocols, and then stained with hematoxylin and eosin (H&E) and Masson's trichrome techniques. The stained slides were observed and photographed under a Leica DM500 microscope with an integrated Leica camera (model ICC50 W).
Statistical analyses were performed according to Zar (2013) using version 3.1.1 of R software (R Development Core Team, 2014). Physicochemical parameters were evaluated using descriptive statistics and compared using ANOVA. Descriptive statistics were applied for the evaluated variables (length of the body, stage of larval development and abnormalities).
Comparisons the body length the tadpoles between sites were made using a t-test for mean differences. Normality and homoscedasticity were corroborated by Shapiro-Wilk and Bartlett tests. In the cases where the assumptions were not fulfilled and for the larval stage of development, the comparisons were made using a non-parametric Kruskal-Wallis test. The level of significance was set at 0.05 for all tests.
Results
The physicochemical parameters of both studied sites are in Table 1. In the two years sampled, the water temperature and conductivity of P2 were significantly higher (F (12, 13.52) = 20.989, P < 0.001, F (3, 8) = 52.384, P = 0.00001), and dissolved oxygen was significantly lower in P2 only in the second year of sampling (F (3, 8) = 25.652, P = 0.00019).
Table 1 Physicochemical parameters measured in situ of the temporary ponds P1 (non-agricultural) and P2 (agroecosystem) during the sampling years (2015-2016).
Temporary ponds | Years | Temperature °C | pH | ConductivityµSiemens/m | OD mg/L |
P1 | 2015 | 21.5 ± 0.44 | 7.43 ± 0.15 | 0.670 ± 0.05 | 6.47 ± 0.15 |
2016 | 22.5 ± 0.55 | 7.20 ± 0.20 | 0.638 ± 0.11 | 5.73 ± 0.35 | |
P2 | 2015 | 23.3 ± 1.06 | 6.89 ± 0.78 | 0.879 ± 0.04 | 7.06 ± 0.40 |
2016 | 24.9 ± 0.10 | 7.43 ± 0.21 | 0.877 ± 0.02 | 4.63 ± 0.45 |
In P1 we registered 28 adult toads and seven amplexus in the first year, and 50 adult toads and 17 amplexus in the second year. Larvae from this site have shown normal swimming behavior like as they distributed in dense groups of active swimming and moved browsing in the bottom and between rocks and plants. In P2 a smaller number of adults (14-20) and amplexus (4-5) were observed during the first and second year of study respectively. Larvae shown abnormal swimming behavior, they were scattered, floating on the surface of the water and did not move or sink.
In spring of 2015 and 2016 a total of 529 larvae were collected from P1 and 1 381 from P2. Larvae from P2 presented a size (t (1908) = 29.56, P < 0.00) and staged (H (1, 1910) = 1295.87, P < 0.001) significantly lower than larvae from P1 (Table 2).
Table 2 Body length (mean ± SD) and larval development stage (mean ± SD) of R. arenarum collected in 2015 and 2016 in the temporary ponds P1 and P2.
Years | Total of individuals | Temporary ponds | Body length (mm) | Stage development |
2015 | 200 | P1 | 4.84 ± 0.26 | 26 ± 0.26 |
1 000 | P2 | 3.30 ± 0.11 | 24 ± 0.10 | |
2016 | 329 | P1 | 4.55 ± 0.48 | 25 ± 0.48 |
381 | P2 | 4.40 ± 0.43 | 25 ± 0.43 |
Larvae collected in P1 did not present any kind of abnormality (Fig. 2A, Fig. 3A) whilst 672 of the 1381 (48.7 %) larvae collected in P2 have presented abnormalities at both macroscopic and microscopic levels.
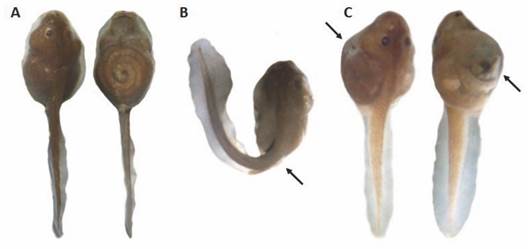
Fig. 2 Abnormalities detected in larvae of Rhinella arenarum. A. Dorsal and ventral views of a normal larva without morphologic abnormalities. B. Severe tail flexure (arrow). C. dorsolateral and ventrolateral views of the abdominal bloating (arrows).
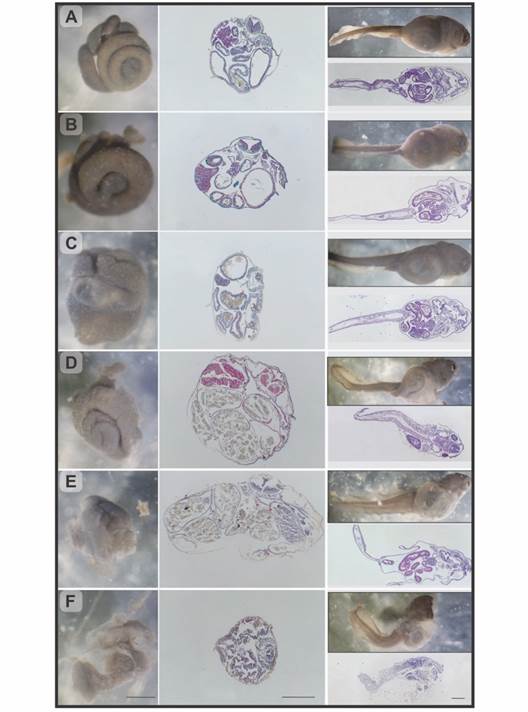
Fig. 3 Rhinella arenarum larvae, observation under stereoscopic microscope and longitudinal and transverse histological sections. A. Grade 0, normal larva. B. Grade 1, mild abdominal bloating. C.-D. Grade 2/3, moderate abdominal bloating, respectively. E. Grade 4, severe abdominal bloating. F. Grade 5, severe abdominal bloating, and compact intestine. H&E and Masson's trichrome techniques. Scale bar: 500 µm.
Macroscopic abnormalities observed were mainly of two types: severe tail flexure (Fig. 2B) and abdominal bloating (Fig. 2C). The analysis of these abnormalities showed that 29.7 % of the abnormal larvae had severe tail flexure in 2015 and 29.6 % in 2016; and in the case of the abdominal bloating, the percentage was 64.8 % in 2015 and 4.19 % in 2016.
The observation under stereoscopic microscope of the individuals with abdominal bloating allowed identification several degrees of severity which, through histopathological studies, were classified into 5 categories (Fig. 3B to Fig. 3F):
Grade 0 - Normal individuals. Spiralized intestine, size, and arrangement normal. In the microscopic analysis the organs did not show morphological alterations. (Fig. 3A, Fig. 4A).
Grade 1 - Individuals with mild abdominal bloating. The intestine shown a normal spiral structure but with thickened intestinal loops. Histologically, a slight displacement of the intestine into one side was observed with a slight bloating of the bowel and stomach (Fig. 3B).
Grade 2 - Individuals with moderate abdominal bloating. Intestine with digestive content, displaced to the right side of the abdominal region, a slight deformation of the spiral structure and thickening of the loops were observed. In the histological samples, a hypertrophy of the wall of the digestive tract was observed with an intense hemorrhage in the stomach and in some intestinal loops. Furthermore, the loops were displaced, resulting in an oval shape of the body in cross section (Fig. 3C, Fig. 4B).
Grade 3 - Individuals with moderate abdominal bloating and loss tissue architecture. Although the observation of the organs in terms of their location and appearance was very similar to the previous grade differences were found at histological level. Stomach and intestine were not differentiated from each other, due to the thinning of the digestive tract wall, which results in the loss of the distinctive features of each organ, such as villi and folds. The entire digestive tract was filled with food (Fig. 3D, Fig. 4C).
Grade 4 - Individuals with severe abdominal bloating. The intestine lost the typical spiral structure and presented thickened and displaced loops. Histologically, as in grade 3, the wall of the digestive tract lost its characteristic architecture and thins, in addition, edema between the intestinal loops and the body wall was observed (Fig. 3E, Fig. 4C).
Grade 5 - Individuals with severe abdominal bloating and compact intestine. Under stereoscopic microscope, the tegument at level abdominal region was shown extremely thin. Due to transparency, reduction or compaction of the intestine and a partial loss of its spiral shape were observed. Free digestive content could also be seen within the abdominal cavity. Histological samples corroborated the rupture of the walls of the digestive tract with great cell desquamation and hemorrhage (Fig. 3F, Fig. 4D).
The percentages presented by each degree (G) of abdominal bloating can be seen in Table 3.
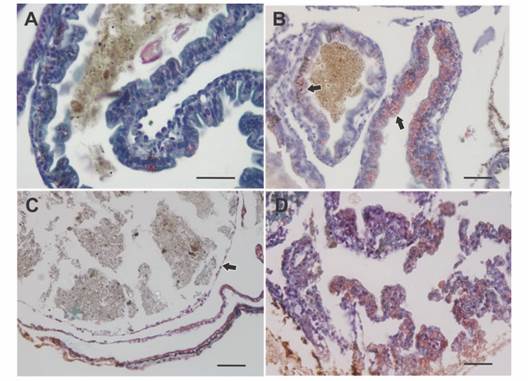
Fig. 4 Histology of the intestinal wall in larvae of Rhinella arenarum. A. Normal intestine. B. Bowel with grade 2 abnormality, there is intense bleeding (arrows) in the digestive tract. C. Bowel with grade 3/4 abnormality, note thinning of the bowel wall (arrow). D. Bowel with grade 5 abnormality, digestive tract wall rupture, loss of tissue architecture, cell peeling, and bleeding. Masson's trichrome technique. Scale bar: 50 µm.
Discussion
The wetlands of the Pampean plain cover a great part of the Buenos Aires province and sit on soils of high productive potential that experiences frequent annual and interannual cycles of flooding and drainage. The intensive agriculture in the pampean plain brings an increase in the trophic state of the lagoons that putatively could lead to a simplification of biotic communities and a significant loss of biodiversity (Quirós et al., 2002). In the surroundings of the La Plata city, farming, floriculture, and intensive and extensive agriculture are practiced. In recent years, studies have determined presence of environmental pollutants in the area, both in water and in sediments (Agostini et al., 2013; Camilión et al., 2003; Demetrio, 2012; Mac Loughlin et al., 2017; Natale, 2006; Peluso, 2011; Peluso et al., 2011; Sansiñena et al., 2018). Published analyses performed in our both sampling sites that surround La Plata city showed that water from P1 has very low concentrations of heavy metals, mainly iron and manganese, which are not detectable or close to the detection limit with the 3050 digestion method of EPA SW 846. In addition, no traces of pesticides in the water or in the sediment were found at this site (Demetrio, 2012; Natale, 2006; Peluso, 2011; Peluso et al., 2011). In contrast, in P2 a high concentration of lead and zinc were detected in the sediment samples (Camilión et al., 2003) as well as pesticides (Agostini et al., 2013; Mac Loughlin et al., 2017; Sansiñena et al., 2018). In addition, it was corroborated that 13 types of pesticides were applied in the P2 during the study period (pers. com.). Based on all this information we are in position to suspect that the high frequency of larvae abnormalities observed in P2 is surely a consequence of intensive agricultural activity that takes place in this site, which causes deterioration in the quality of environment due to contamination of water and sediments with phytosanitary products (mainly pesticides).
In the two reproductive events studied (2015 and 2016) we found, in P2 a lower number of amplexus and clutches than P1, a question that denotes that population of R. arenarum toads are possibly lower in ponds surrounded by cultivated areas.
In 2015 the larvae from P2 presented smaller size and development than those observed in P1. Although it may be due to population differences in the development and growth rates, we know that effects of climate are the same for both sites studied, since there are distanced by no more than 20 km in a straight line. Thus, we must consider that the high amounts of abnormal larvae and the high level of mortality we observed in P2 are due to extrinsic factors. The detected abnormalities had an evident effect on the behavior of the larvae which presented several difficulties to swim, fed, and finally caused his death. Therefore, the reduced fitness may be the reason why the larvae presented less growth and development (Denoël et al., 2012). The total percentage of abnormal larvae observed by these authors far exceeds 5 %. This number is, according to Read (1997), the basal level of natural occurrence of abnormalities in anuran larvae. Severtsova et al. (2012) reported a higher percentage of larval abnormalities, 18 % in Rana temporaria (Linnaeus, 1758) and 16.5 % in Rana arvalis (Nilsson, 1842). These authors associated these high levels of abnormalities with urban impacts. Furthermore, Anzaldua and Goldberg (2019) described a high concentration of Osteopilus septentrionalis (Duméril & Bibron, 1841) larvae with morphological abnormalities living in drainage ditches that had been treated with insecticide. Some of the abnormalities these authors report were severe tail flexure and spinal deviations or severe spinal curvatures that clearly affect the motility of the larvae and consequently their survival (Wilbur & Semlitsch, 1990). This abnormality was reported in several laboratory works, and it was linked to the effect caused by pesticides (Agostini et al., 2010; Brunelli et al., 2009; Svartz et al., 2016) and by heavy metals (Haywood et al., 2004; Natale, 2006; Pérez-Coll et al., 1988). In field work, the observation of this type of abnormality is scarce, and is generally associated with different types of contamination, such as waste from coal combustion (Hopkins et al., 2000), wastewater (Ruiz et al., 2010), chlorides and metals (Severtsova et al., 2012), and pesticides (Anzaldua & Goldberg, 2019). According to Bantle (1991), severe abnormalities in the tail are associated with defects during the formation of the notochord but with no effects in other body parts and organs. In the case of the abnormal larvae studied by us they presented tail flexure associated to abdominal bloating.
Lajmanovich et al. (2012) reported larvae of Dendropsophus nanus (Boulenger, 1889) with abdominal edema and inverted swimming (belly up) coinciding with our observations in R. arenarum. A similar case was registered by Natale et al. (2018) in larvae of Boana pulchella (Duméril & Bibron, 1841) exposed to the pesticide Aficida®. Toxicological tests performed by Sansiñena et al. (2018) also showed subcutaneous edema in larvae of B. pulchella exposed to agroecosystem sediments. This overview suggests that the abdominal bloating observed in larvae of different species is related to exposure to agrochemicals.
The analysis carried out in this work provides evidence on the occurrence of a series of deformities, such as alterations in swimming caused by abnormalities in the tail, abdominal bloating, or both. This abdominal bloating would initially manifest itself as a thickening of the intestinal loops, generating a space between the organs of the abdominal cavity and the tegument, and in severe cases could induce the rupture of the digestive tract wall. All these events would lead to an inability to digest and absorb food, with the consequent accumulation and decomposition of food in the digestive tract. This would cause, on the one hand, a slower growth of the individual and, on the other, a dysfunction of the whole organism, which would trigger the death of the individual.
In conclusion, this work reports of an event of singular relevance: the high frequency and severity of abnormalities observed in the early stages of R. arenarum larvae living within an agroecosystem. The work achieves a detailed description of the abnormalities observed at both anatomical and histological level and proposes a distinction of the several degrees that the larvae suffer through during abdominal bloating, and that could culminate in death. Furthermore, it gives evidence on the negative impact that agricultural activity causes on aquatic ecosystems that are surrounded by crop areas, on the population dynamics, among other population parameters, causing a population decline. In addition, the finding in the field of abnormal larvae is an event of great relevance on the biology and ecology of the species, due to its severity, and in the ecotoxicological context raised, it is an indicator of the existence of stress on the ecosystem, highlighting the role of amphibians as indicators of the health of the environment. Given the percentages of occurrence of malformations observed in the present study, P2 could be a ''hotspot'' according to criteria of Lanoo (2008), for which it is necessary to continue with frequent systematic and continuous monitoring at this site to achieve an early detection and a complete record of this type of events. Finally, it is necessary to be aware of these events, which undoubtedly occur and are not easy to detect unless prolonged and detailed sampling is carried out in the field.
Ethical statement: the authors declare that they all agree with this publication and made significant contributions; that there is no conflict of interest of any kind; and that we followed all pertinent ethical and legal procedures and requirements. All financial sources are fully and clearly stated in the acknowledgements section. A signed document has been filed in the journal archives.