Introduction
A globalized market for dental products through online purchasing venues have provided the opportunity for dentist all around the world to get access to more brands and manufacturing origins of biomaterials, sometimes at significantly different market prices. Despite the clinical results which a biomaterial may have proved, its physico-chemical composition could greatly vary between different brands because of differences in the manufacturing process, local legislation, and/or quality of the raw materials. These variations may alter the clinical properties of the biomaterial and sometimes the clinician could be unaware of these differences when treating a patient. Moreover, patient’s safety may be at risk as some of these materials may have not been properly analyzed and its clinical effectiveness and safety parameters have not necessarily been established (1). All the scientific evidence supporting a biomaterial like Mineral Trioxide Aggregate (MTA), even if it has been widely studied for many years, may pose a threat to the expected biomaterial’s properties, if they vary from one brand to another.
Since the introduction in the dental market of MTA in 1999, it becomes one of the most studied materials in endodontics; used mostly for direct pulp capping, management of endodontic perforations, and as retro-filling material (2,3,4,5). MTA is similar in composition to Type 1 Portland cements, composed of calcium oxide (50-75%) and silicium dioxide (15-25%) (6). To improve its radiopacity properties, MTA has bismuth oxide added to its composition and it undergoes a purification process to enhance its biocompatibility and reduce its biotoxicity, containing fewer potential toxic elements (i.e., arsenic) and resulting in a smaller particle size when compared to other Portland cements (7,8).
ProRoot MTA (Dentsply Tulsa Dental Specialties, TN, USA) was the first commercially available MTA in the market (3). A few years later, the same company introduced a White MTA version, in response to a clinical concern of the staining potential of the dental surface caused by the grey color of the original product. Since then, other companies have presented different versions for MTA, claiming similar clinical performance of their products. For example, MTA Angelus (Angelus, Londrina, Brazil) became available in the United States market after receiving FDA approval in 2011. More recently, a new presentation of this bioceramic with a semi-liquid vehicle of water-soluble silicone-based gel was made available, with promising biological properties and different physical presentations (9).
As new products, under the same label of bioceramics have been introduced in the dental market, their properties have been compared in vitro and in vivo (10). Information about the properties of different brands of MTA and comparative analyses of these products are limited and mostly looking into the biocompatibility and cytotoxicity of these materials (11,12,13). One study analyzed the biological effects of MTA-based endodontic bioactive materials and compared them in an in vitro study (14). A similar behavior was found between the tested materials when analyzing cell migration, cell proliferation, and cell attachment. A review of the literature evaluating only the cell attachment properties of Portland cement-based biomaterials also demonstrated favorable properties of these materials even when assessing a wider range of commercial brands (15). However, little information is available on the physico-chemical composition of different MTA cements, including products manufactured in developing countries without specific quality and safety certifications. In a 2008 study, researchers evaluated the presence of arsenic in different types and brands of MTA, white Portland Cement and gray Portland cement (16). Their findings demonstrated the great variability between the tested biomaterials, which, in some cases proved levels of this carcinogenic element above the ISO-recommended limit for these materials. Another study confirmed a great variability in the elemental composition of Portland Cements and MTA products (17). A flow particle image analyzer study compared the particle size and shape of commercially available MTA and Portland cements, once more finding variabilities between different brands (18). All these reports demonstrate that bioceramic products widely vary in their physical composition according to the brand and sometimes the origin of the product.
Few studies have compared the chemi- cal characteristics of MTA and Portland cements (19,20,21), nonetheless these studies included only ''popular''commercial brands which are commonly used for in vitro and clinical studies, failing to include ''less-popular''brands manufactured in developing countries which are also commercially available and used daily in patients around the world. Hence, the aim of this in vitro study was to analyze and compare the physical and chemical properties of four commercially available MTA cements of different origin. The working hypothe- sis was that the different brands of MTA presented differences in their physico-chemical properties.
Materials and methods
Preparation of samples
The MTA cements included in this in vitro study were ProRoot MTA (Denstply Sirona, Tulsa, USA), Angelus MTA (Angelus Dental, Paraná, Brazil), MTA Flow (Ultradent Inc, Utah, USA), and MTA Viarden (Viarden, Mexico), bought directly from commerce. Pure powder and mixed hydrated specimens of the 4 brands were prepared following the manufacturers indications. The powder samples were evaluated by Scanning Electron Microscopy (SEM), Thermogravimetric Analysis (TGA) and Energy-Dispersive X-ray Spectroscopy (EDS). Mixed samples were prepared following manufacturer’s instructions and evaluated by SEM analysis.
ProRoot MTA samples were obtained placing the complete content of a 1g package in a mixing pad and using the plastic mixing stick provided, the liquid micro-dose ampule was gradually incorporated and mixed for one minute until an even mass was obtained. Angelus MTA samples were obtained by mixing one spoon of powder with one drop of the distilled water in a sterile glass slab using a metal spatula for 30 seconds until obtaining a homogeneous mixture. MTA Flow samples were prepared to obtain a thick consistency by mixing two big ends of powder using the provided measuring spoon with two gel drops in the mixing pad using a sterile metal spatula. Finally, MTA Viarden samples were obtained by mixing one pre-dosed capsule of powder with the solution provided in a sterile glass slab for one minute. All mixed samples were placed in a desiccator for 24 hours before preparation for microscopic examination.
TGA analysis
Thermogravimetry determines the sample weight changes in function of temperature. Unhydrated samples were evaluated placing appro- ximately 5mg of the MTA powder in the built-in high-sensitive balance of the TGA unit (Q500, TA Instruments, New Castle, DE, USA). Samples were automatically weighted and tared. Once the furnance was sealed, the equipment was progra- med to run a thermal ramp, initiating at 50ºC and ending at 1000ºC; with a heating rate of 10ºC per minute. TGA curves showing the final percentage of mass loss and the inflection point (derivative of the TGA curve (%/ºC)) (Tp) were calculated.
SEM analysis
Pure powders and prepared samples were analyzed using a SEM unit (ASEM Microscopy JEOL JSM-6390LV, USA). Three samples of each product were placed in aluminum cylindrical mounts covered with carbon conductive tape. For unhydrated samples, 100mg of each MTA were evaluated at 1000X, 3000X, and 7000X. Hydrated samples were evaluated at 500X, 2000X, and 5000X. Representative images were obtained to describe the superficial appearance of the samples.
EDS analysis
Unhydrated samples from of each MTA were prepared for EDS analysis, using the same mounts and preparation procedure used for SEM. These analyses were conducted at 500X by triplicate to compare the proportion of Calcium (CA), Silicon (Si) and the Ca/Si proportion. Each lecture was comple- ted until an approximate number of 1000-1200 counts was reached. The data obtained was catego- rized, and statistical analysis was performed.
Statistical analysis
Shapiro-Wilk and Levene’s tests were conducted to determine the distribution and variance of the data. ANOVA and post-hoc Tukey tests at a 0.05 significance value were used to compare the Ca and Si content; and the Ca/Si proportion for each product. SEM and TGA analy- ses were qualitatively described.
Results
Figure 1 presents the TGA thermograms for each unhydrated MTA sample showing thermal decomposition over time and the final mass loss percentage at 1000°C. While ProRoot MTA, MTA Angelus, and MTA Flow showed similar thermal profiles and final mass losses below 2%, MTA Viarden showed a higher mass loss percentage (9.94%). Also, the thermal profile of MTA Viarden is different form the other samples exhibiting a sudden drop at 650°C not perceptible in the TGA analyses. Figure 2 shows the plot for the derivate thermograms (DTG), indicating mass loss rates depending on the temperature increase. For ProRoot MTA, MTA Angelus and MTA Flow, Tp can be observed before the 650ºC. After this temperature the DTG shows minimal changes. Nonetheless, for the MTA Viarden sample, Tp was observed at 650°C, showing a different pattern when compared with the other three products.
Figures 3 and 4 show the microscopical analysis of pure powders and prepared samples of the four cements at different magnifications. ProRoot MTA in Figure 3 exhibited homogeneous particles under the 10µm size. A very similar aspect can be appreciated for the MTA Flow particles. Angelus MTA showed instead a heterogeneous combination of particles; some of them with similar appearance to the ProRoot MTA and MTA Flow particles but bigger in size and also a standout presence of crystalline rectangular prisms with larger sizes (40 to 60um). MTA Viarden revealed an irregular particle pattern with big clusters where other smaller particles seem attached. Figure 4 showing the prepared cement samples was different in appearance to the pure samples. ProRoot MTA and MTA Flow showed a similar homogenous granular aspect at low magnification (500x). MTA Angelus sample showed larger particle sizes when compared to ProRoot MTA and MTA Flow with smooth irregular structures and the rectangular prisms observed in the unhydrated sample could still be appreciated in the hydrated sample. MTA Viarden, as in the pure sample, displayed the most irregular particle presentation in size and also in shape. The bigger particles observed in Figure 3 could also be observed for this sample.
Figure 5 shows the EDS analyses for pure powder samples of the 4 cements. Histograms of the elemental analysis revealed similar distribution of elements such as Ca, O, Si, Al and C. However, the MTA Viarden sample showed the presence of technetium (Tc), an absent element in the other samples. The difference between groups of the Ca percentage was significant with ANOVA (F-value=22.1, Df=3, p=0.00031). After pairwise comparison, the Ca proportion was statistically similar between ProRoot MTA, MTA Flow and MTA Angelus, and only the MTA Viarden is significative different among other groups (Tukey contrast p<0.05). The difference between groups of the Si was also significant with ANOVA (F-value=31.4, Df=3, p=0.00009). Once again, the pairwise comparison showed that only the MTA Viarden was significative different among other groups (Tukey contrast p<0.05). The difference between groups of the Ca/ Si proportion percentage was significant with ANOVA (F-value=10.3, Df=3, p=0.0041). Once again, only the MTA Viarden was significative different among other groups (with Tukey contrast p<0.05).
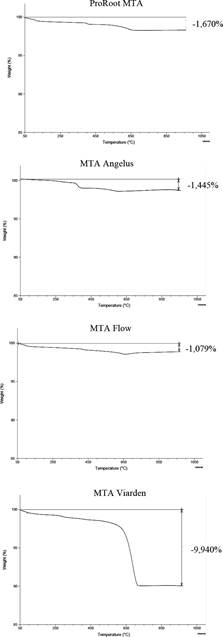
Figure 1 TGA analysis thermal ramp, initiating at 50ºC and ending at 1000ºC, with a heating rate of 10ºC per minute. Mass loss percen- tages are shown for each sample.
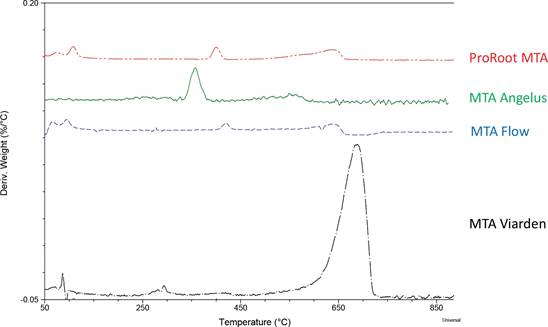
Figure 2 TGA analysis. The first derivative of the weight loss curve (%/ºC) shows the inflection point for each sample.
Discussion
The materials evaluated in this study included four brands of MTA available in the Latin American dental market. MTA Flow and ProRoot MTA are manufactured in the United States; MTA Angelus is manufactured in Brazil and, as the first two, also has FDA approval (9). MTA Viarden is manufactured and marketed almost exclusively in Mexico. TGA, SEM and EDS techniques were used to compare the physico-chemical properties of these MTAs.
Thermogravimetry has been widely used in the construction industry to investigate hydrated Portland cement, which is the main component of MTA (80% Portland cement, 20% bismuth oxide) (22,23). Because it constitutes a complex material which may contain minor hydration products, calcium carbonate, and other supplementary cementitious materials, by means of thermogravimetry it is possi- ble to identify the phases present in hydrated concrete, such as aluminates, portlandite (calcium hydroxide), calcite, calcium silicate hydrate (CSH) and unreacted clinker. In this investigation, however, MTA unmixed powders were analyzed to determine the mass loss in function of temperature changes of the samples. At 1000°C, less than 2% mass losses were observed for all brands except for MTA Viarden, which displayed a greater mass loss (almost 10%). Generally, the mass loss at lower temperatures in hydrated Portland cement could be explained due to dehydration of water chemically bound to the mineral phases, nonetheless at temperatures above the 400°C, mass loss is due to phase decomposition (24). The inflection point for MTA Viarden coincides with the decarbonation of calcium carbonate which occurs between 600°C and 850°C (25), suggesting that this compo- nent may be present in this biomaterial; however further chemical analyses are needed to confirm its presence. The addition of this filler constitutes a common technique to decrease manufacturing costs by replacing part of the clinker with calcium carbonate. During the hydration of Portland cement, a portion of the clinker remains unhydrated and is kept as filler. However, it has been determined that the addition of this mineral interferes with the hydration reactions of Portland cements and is not an inert filler. This mineral may alter the mineralogy of the hydrated phases of concrete by interacting with the aluminous phases, called monosulfate (Afm) and ettringite (Aft), which form during the initial hydration of the cement. As a result, these are transformed into carboaluminous phases (26). Calcium carbonate if added in low amounts (less than 15%), has beneficial economic effects as it decreases the amount of calcium sulfate needed to make cement, reduces the setting time, as is an easier mineral to grind than clinker, increasing also the hydration rate by acting as a nucleation site for CSH growth. At higher percentages, however, it negatively affects the compressive strength and increases the porosity. Because of these undesired effects, many countries consent a maximum percentage replacement of clinker with limestone, so as not to negatively affect the properties of this material. For example, in China and Brazil, up to 10% is permitted, in Argentina 20%, in the United States from 5% to 15%, depending on the type of cement, and in Mexico and Europe up to 35% (27). As mentioned before, further investigations, using techniques such as X-ray Diffraction Analysis could help to verify this assumption. Other reports have shown the content of calcium carbonate in ProRoot MTA to be as low as 1.4% (28) and no calcium carbonate was found in MTA Angelus (29). The deposition of calcium hydroxide during hydration of tricalcium silicate-based materials is crucial to initiate all biological reactions between the dentin/bone/tissue interface and the MTA (30). It has been concluded that although MTA-based cements with calcium carbonate release calcium ions, the amount of free calcium hydroxide, the main ingredient for a biological interaction with phosphate ions in the tissue fluids and the formation of some kind of mineralized barrier in the adjacent tissues, is decreased (31).
Energy dispersive x-ray spectroscopy has proven to be a useful method to study the elemental composition of MTA (32). This technique has been used to study the calcium, silicon, iron, aluminum and bismuth content in ProRoot MTA and MTA Angelus in other investigations (33,34). As reported, it has been concluded that these two brands of MTA possess similar elemental composition (35,36) The detected percentage of calcium and silicon in the unhydrated powder found in this study is similar to those previously obtained by Camilleri et al. (29). As MTA Flow has been recently introduced in the market and the number of studies characterizing this material are limited, but its physicochemical properties seemed to be similar to MTA Angelus since, when the hydrated cement was analyzed by EDS, it showed a comparable elemental composition to the Brazilian cement (37). Therefore, it is not surprising that in this investigation the elemental analysis of the MTA Flow powder was similar to the MTA Angelus and ProRoot MTA cements.
MTA is manufactured by combining Portland cement with a radiopacifier, such as bismuth oxide, calcium tungstate or tantalum pentaxodium. The manufacture process of Portland cement is achieved through various types of raw materials and depends very much on the materials available in each country and in their own quality standards, as discussed previously. Consequently, one of the most important steps in the production process is the selection of high-quality raw materials. Some of these raw materials are: limestone or chalk as a source of calcium carbonate; shale, clay, marl, sand or mineral debris as a source of silicon and aluminum, and finally iron ore. When calcined, calcium carbonate is transformed into calcium oxide and releases carbon dioxide; the calcium oxide then reacts with the other minerals added in the kiln and, when the temperature reaches 1400-1500C, clinker (tricalcic, dicalcic and aluminate silicate nodules) or the new mineral phases are formed (38). At this point, limestone can be added again to dilute the clinker. As limestone is the main component of Portland cement (source of calcium oxide) and shale or clay the most important secondary component, and as different regions of the world have very different mineral compositions (39), each country regulates the quality of these raw materials differently. The low calcium percentage values in MTA Viarden could be explained due to i) the use of a limestone that does not had the minimum percentages of calcium carbonate concentration (39), ii) variations in temperature and sintering protocol to decrease costs or iii) the use of low-quality fillers to dilute the clinker. All these hypotheses must be analyzed.
With respect to the temperature and sintering time used during cement manufacture, it has been found to affect the mineralogy of the material (C3S and C2S and C3A content) (23). A cement with a low calcium content could suggest a low sintering temperature, which leads to an incomplete chemical reaction and a higher number of oxides remaining unreacted, resulting in a lower amount of tri and di-calcium silicate phases in the final product (29,40). The even lower silica content and the resulting high Ca/Si ratio maybe a result of the use of a silicon oxide mineral source with a low silica modulus (SR or silica ratio). The silica modulus controls the composition of clays and lean (marl); the higher the SR, the higher the amount of alite and belite in the clinker (41), but manufacturing costs are increased as higher kiln temperatures are required (42). As mentioned, another explanation for this low amount of silica and calcium may be the mixing of the main raw material with low quality secondary compounds such as fly ash and blast furnace slag as a costcutting measure. Both materials are commonly used in the concrete industry; fly ash is mixed with clay during the manufacture of Portland cement because it is a cheap source of aluminates and silicates and blast furnace slag is widely used as a filler source of calcium oxide, aluminates and silicates. However, their chemical composition according to ASTM C 618 must meet minimum mineral composition requirements (67% CaO+SiO2+MgO for slag and 70% SiO2+ Al2O3+Fe2O3 for ash) to obtain a high-quality cement (43). During hydration of MTA, a calcium silicate hydrated gel is formed from tricalcium silicate (C3S). This gel has a calcium to silica ratio of 1.7, whereas trical- cium silicate has a calcium to silica ratio of 3. Because of this, the excess calcium precipitates in the form of calcium hydroxide and many of the positive biological properties of MTA are triggered (30). The low content of both elements could affect the release of calcium hydroxide and affect the pulp or periapical response, since calcium ions stimulate pulp cells and facilitate mineralization by activating adenosine, while hydroxyl ions increase the pH, have antibacterial action and activate the enzyme alkaline phosphatase and bone morphogenetic protein BMP (44).
It is also important to discuss the possible presence of technetium in MTA Viarden, a chemical element whose isotopes are considered radioactive. Ninety-nine percent of Earth’s technetium is found as 99Tc and can be found in water, crust, atmosphere, sediments, plants and marine animals; mainly near areas that have experienced direct contamination by atmospheric nuclear testing (mainly during the second half of the 20th century) or by specific sources such as nuclear fuel reprocessing plants. Also, a significant amount has been found in coral formations near nuclear accidents, which has been explained by the presence of carbonate, which is capable of forming carbonate complexes with Tc (IV). Another possible source of technetium in the production chain of the Portland cement could be some type of iron-containing minerals, since these constitute the most important sorbents of Tc sorption (45). This finding, together with the low amount of calcium and silica, raises doubts about the chemical composition of this biomaterial. Further analytical analyses are needed to confirm the permanent presence of Tc in different lots of the same material.
SEM has been widely used to characterize the microstructure and visualize the surface of MTA (30,46,47). In this study, ProRoot MTA powder and MTA Flow were shown to have a more homogeneous composition and smaller particle size, while MTA Angelus exhibited small particles similar in size, but larger particles in the form of rectangular prisms. These findings are similar to what has been obtained in other investigations that have studied the particle size of MTA by laser diffraction and flow particle analyzer. Angelus MTA has been shown to have a lower particle circularity and more irregular particle size distribution (d90=42.84um) than ProRoot MTA (d90=4.32) (18,48) with long spindle-shaped particles similar to those observed in this investigation. EDS analysis have shown these particles to be composed of bismuth (46,48). The more irregular particle pattern for MTA Viarden, with particles larger than 30um, could be a result of the different cement manufacturing process. These differences can modify the particle’s size, which plays a vital factor in setting time and physical properties of MTA (48). The manufacturing temperature (23) and different grinding and sieving methods and protocols (48) contribute in generating a cement with smaller particle size and larger tricalcium silicate, which grant its biological properties. Particle’s size plays an important role in the MTA setting time because larger particles react slower and have a smaller surface area per unit mass, which results in an increase of the setting time (49). This in turn leads to clinical disadvantages because it makes the cement more prone to washout (dislodgment risk) and blood contamination (50). In addition, the material’s penetration into the dentinal tubules (diameter 2-5um) and consequently the hydraulic seal of the material may be affected with the larger particle size (18). The differences between MTA Viarden and the other hydrated cements demonstrate a very irregular and heterogeneous cement, which is to be expected considering the differences observed in the non-hydrated phase. The lower porosity observed in the Angelus MTA can be explained by the lower concentration of bismuth oxide (14%), which results in a lower water:powder ratio compared to ProRoot MTA (20%) (51). Although, the bismuth concentration for MTA Flow has been shown to be similar to MTA Angelus (37), MTA Flow is mixed with a diffe- rent vehicle which enhances the handling of the cement and may also alter its physical properties and hydration (52).
In summary the findings of this study revealed the physical and chemical properties of four tricalcium silicate-based cements which can be found and bought commercially under the same name. When comparing all the products, it is evident that Viarden MTA offers different physicochemical characteristics. This material is available for less than half the price of any of the other three brands and because it can be purchased over the internet, it could eventually constitute a gray market material. MTA Flow, ProRoot MTA and MTA Angelus showed similar characteristics to each other, however MTA Viarden shows lower calcium and silica content, higher thermal instability, and a more irregular particle size distribution pattern. Further research is needed to determine if these physical and chemical differences can affect the other MTA characteristics such as biocompatibility, pH, solubility and bioactivity, compressive strength, and flexural strength.
Conclusion
The four MTAs demonstrated to vary significantly in their chemical composition and physical characteristics. Clinicians must be aware of the differences between different brands of a same material, and future research should focus on the clinical implications of these differences.
Author contribution statement
Conceptualization and design: M.M.A.,A.P.G. and D.CH.B.
Literature review: M.M.A., E.U.R. and D.CH.B.
Methodology and validation: M.M.A., J.V.B., R.P.R. and D.CH.B.
Formal analysis: J.V.B., R.P.R. A.P.G. and D.CH.B.
Investigation and data collection: M.M.A., J.V.B., R.P.R., E.U.R. and D.CH.B.
Resources: M.M.A. and D.CH.B.
Data analysis and interpretation: M.M.A., A.P.G.,
E.U.R. and D.CH.B.
Writting original draft: M.M.A., E.U.R. and D.CH.B.
Writting review and editing: J.V.B., R.P.R., A.P.G.,
E.U.R. and D.CH.B.
Supervision: J.V.B., A.P.G. and D.CH.B.
Project administration: D.CH.B.
Funding acquisition: M.M.A., J.V.B. and D.CH.B.