Introduction
Coral reefs are in decline globally due to many factors including climate change, pollution, inadequately planned coastal development, mismanagement of fisheries, and eutrophication (Sandin et al., 2008, Van Oppen et al., 2017). In addition to the ecological impact, this decline has an economic impact on societies that depend on coral reefs for their livelihood (Costanza et al., 2014). The marine conditions responsible for this decline in reef ecosystems can inhibit the recuperation of a coral reef following a disturbance to the reef environment (Anthony et al., 2020; Good & Bahr, 2021; Rinkevich, 2005). Coral reef restoration is one form of human mediation that aims to rebuild coral reef communities through active propagation of coral in order to promote the recovery of the ecosystems'natural complement of diversity and ecological functionality (Hancock et al., 2017; Horoszowski-Fridman et al., 2015). The most common method of coral reef restoration is through coral gardening and transplantation (Bayraktarov et al., 2019).
Coral restoration is essentially a facilitation of natural successional processes through the reintroduction and management of key species (Walker et al., 2007; Young, 2001). Ecological succession is the process by which an ecosystem undergoes a predictable sequence of the replacement of communities that reach a stable climax and remains reasonably unchanged over time until the next disturbance (Odum, 1964). The early succession of a reef is critical to the establishment, survival and resilience of coral reef communities (Doropoulos et al., 2016). For example, algal turf impedes the settlement of coral larvae and the growth of colonies (O'Brien & Schiebling, 2018; Smith et al., 2010). Conversely, coralline crustose algae promote the settlement and growth of corals (Doropoulos et al., 2016).
Coral growth rate is one of the most widely used assessments of coral reef health and restoration success (Edmunds & Putnam, 2020). The growth of corals can be affected by environmental factors including temperature (Jiménez & Cortés, 2003; Manzello, 2010), seawater pH (Guo et al., 2020; Manzello, 2010), nutrients (Toh et al., 2014), light (Wellington, 1982), depth, and biological factors such as the presence of certain fish, invertebrates (Glynn & Enochs, 2010) and algae (Lirman, 2001). Coral growth information is used to assess and predict the environmental conditions and locations where corals can survive and thrive (Edmunds & Putnam, 2020).
The succession of coral reefs occurs at short time scales in the range of months and geological time scales that occur over centuries, millennia or even millions of years (Grigg, 1983; Karlson, 1999). At short time scales, succession is localized on small patches of substrate and is dominated by fast-growing organisms such as algae competing for light, space, and other resources. This short time scale succession is the most relevant to processes of coral recruitment, competition, and growth (Doropoulos et al., 2016). Coral reef succession at geological time scales is characterized by turnovers of species of hermatypic corals and the physical structure of the reef as long-term changes in the ecology, environment, and frequency of disturbance favor the growth of certain species and forms over others (Grigg, 1983). For example, fast-growing branching corals such as pocilloporids and acroporids tend to be early colonizers of a new reef that are sensitive to environmental changes and are replaced by slower growing, more tolerant, massive species that build on the physical and ecological foundations of the branching species, eventually replacing them as the dominant growth forms (Grigg, 1983).
Coral reefs are ecosystems characterized by constant disturbance, including anthropogenic and natural factors. This constant disturbance contributes to the high diversity of life in coral reefs (Grigg, 1983) and also their vulnerability to decline. Disturbances such as high sediment loads, elevated temperatures from El Niño Southern Oscillation (ENSO) events stress corals and cause them to lose their zooxanthellae symbionts a process known as coral bleaching. If a disturbance is strong and persistent, a coral reef ecosystem can settle into alternate stable states dominated by different organisms depending on environmental and ecological factors (Dudgeon et al., 2010; Fung et al., 2011). Thus, as succession follows a disturbance, its trajectory can be affected by these environmental and ecological factors. For example, under conditions of constant disturbance, climate change and other anthropogenic factors tend to push recovering ecosystems to favor the proliferation of macroalgae and turfs instead of reef-forming corals. Algae-dominated marine ecosystems often do not naturally revert to coral-dominated ecosystems without intervention (Smith et al., 2010). This is because reef-forming corals tend to be out-competed by algal turfs that often flourish in ecosystems with high water nutrient concentrations and fewer herbivores; a condition that can occur in ecosystems degraded by eutrophication and poorly regulated fisheries (Smith et al., 2010). However, processes such as herbivory and coral expansion that encourage the ecological trajectory towards a self-sustaining reef ecosystem are also evident in the dynamics of the community of benthic encrusting organisms on the reef bottom (Ceccarelli et al., 2011; Fong et al., 2016).
The benthic sessile community of a reef is a complex and competitive arena. The main competitors are often simplified into the following categories: turf, an overarching term for a multispecies, dense, often filamentous mat of algae; crustose coralline algae (CCA) that can promote the growth and recruitment of coral; and scleractinian corals (Flower et al. 2017; Smith et al., 2010; Steneck & Dethier, 1994). Corals, especially highly branched species such as Pocillopora, provide structural complexity to a reef ecosystem that attracts diverse and abundant populations of fish and other associated fauna (Stella et al., 2011). Herbivorous coral associates control populations of turf and macroalgae that inhibit the growth and settlement of coral and thus encourage the expansion of the reef (Burkepile & Hay, 2010; Hixon & Bronstoff 1996; Humphries et al., 2014). Seasonal shifts in the populations of species associated fauna can influence the interactions between algae and coral (Brown et al., 2019; Doropoulos et al., 2016; Fong et al., 2016; Muthukrishnan et al., 2016).
Favorable sites for coral reef restoration are areas where corals previously flourished and are unlikely to settle and grow at a new site under current marine conditions (Rinkevich, 2005). Likewise, the most appropriate candidates for species to be introduced are those that might not colonize the restoration area unassisted (Society for Ecological Restoration International Science & Policy Working Group (SER), 2004). In Golfo Dulce, an ecologically unique tropical fjord-like embayment on the southern Pacific coast of Costa Rica, historically abundant branching corals in the genus Pocillopora have practically disappeared. The initial loss of Pocillopora was due the change in the path of rivers that brought fresh water and sediments into the Gulf (Cortés, 1991; Cortés & Reyes-Bonilla, 2017). In more recent history, coastal development and deforestation around the rivers liberated sediment and resulted in the further destruction of the gulf's reefs and a near complete loss of Pocillopora corals (Cortés, 1991). With so few colonies remaining, the population of Pocillopora in the gulf could have experienced the Allee effect, a condition of a community where there is not enough population density to sustain sexual reproduction (Allee, 1938; Gascoigne & Lipcius, 2004). A small number of Pocillopora colonies in Golfo Dulce that have demonstrated particular resilience to sedimentation and thermal stress (J. Kleypas, pers. comm., 2020) have become the basis of a coral reef restoration effort to preserve and propagate the genus in the Gulf.
Monitoring the health and growth of transplanted corals and changes to the ecosystem around them provides insight into whether those colonies are functioning ecologically as ''ecosystem engineers,” and thus can inform future reef restoration efforts. This exploratory study aimed 1) to monitor the health and survivorship of a cohort of transplanted Pocillopora spp. colonies in Golfo Dulce, South Pacific Costa Rica, 2) calculate the growth rate of the corals in terms of area, and 3) to describe the effect of the transplantation of the colonies on a short time scale succession of the sessile benthic community. This information will aid reef restoration scientists in understanding how the reef community changes following coral colony transplantation.
Materials & methods
Study area: Golfo Dulce is a narrow-mouthed embayment (8° 27'- 8° 45'N, 83° 07'- 83° 30'W) oriented northwest to southeast between the Osa peninsula and the southern Pacific coast of Costa Rica (Fig. 1). The Gulf has an approximate length of 50 km and width between 10 and 15 km and covers an area of 680 km2 with a maximum depth of 200 m. The dry season lasts from December to March with an average rainfall of 100 to 160 mm per month (Fig. 2). The wet season peaks in October with an average rainfall of 800 mm (Cortés, 1990). The monthly seawater temperature ranging from 18 º to 35 ºC with annual average of approximately 26.5 ºC (Fig. 3).
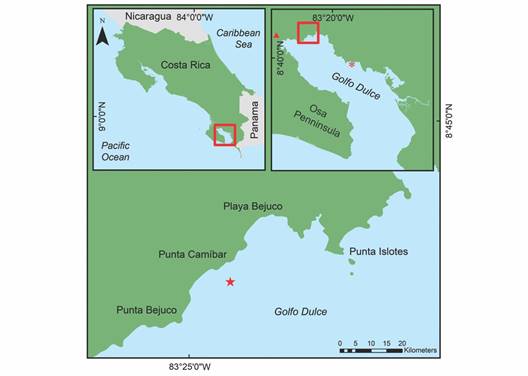
Fig. 1 A. The location of Golfo Dulce within Costa Rica. B. The transplantation site at Punta Bejuco is marked with a red star and Nicuesa site is marked with a red asterisk and the red triangle marks where the meteorological data were collected. C. The location of the transplantation and control sites near Punta Bejuco marked with a red star.
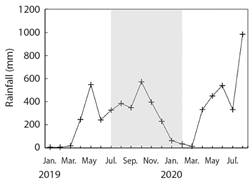
Fig. 2 Rainfall (mm month-1) data from January 2019 through August 2020 from the Instituto Meteorológico Nacional de Costa Rica station, near Golfo Dulce (08º 42'03” N, 83º 30'49” W, at 80 m above sea level). Gray shading indicates the timeframe of the experiment.
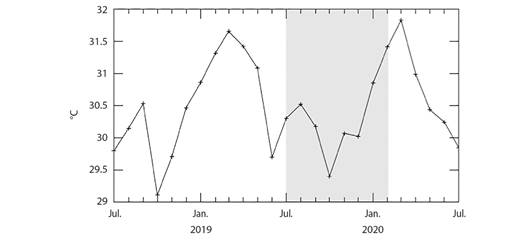
Fig. 3 Area-averaged daytime sea surface temperatures from the northern tip of Golfo Dulce from July 2018 to July 2020, as derived from the MODISA satellite data using the NASA GIOVANNI web application for the coordinate rectangle: - 83° 26'12”, 8° 42'3”, - 83° 24'38, 8° 43'14.88”. Gray shading indicates the timeframe of the experiment.
Geographically and ecologically, Golfo Dulce is part of the Eastern Tropical Pacific (ETP) marine biological province (Glynn et al., 2017; Guzmán & Cortés, 1993). It is often referred to as a tropical fjord due to its anoxic deep waters and bathymetry (Cortés, 1990; Wolff et al., 1996). The anoxic waters prevent the energy contained in detritus that sinks to the depths of the gulf from being recycled back into the higher trophic levels of the ecosystem (Wolff et al., 1996). These unique conditions result in a community structure of fish and invertebrates that differs significantly from other marine ecosystems of the Pacific coast of Costa Rica (Alvarado et al., 2014).
Coral Nurseries: The experiment was performed between July 2019 and February 2020. The coral colonies used in this study were cultivated in in situ nurseries at Playa Nicuesa (Fig. 1) as part of the restoration initiative of the NGO Raising Coral Costa Rica and the Center of Marine Science and Limnology (CIMAR) of the University of Costa Rica (UCR). The coral nurseries are tree-shaped structures with central PVC ''trunk” and radiating fiberglass ''branches” to which the Pocillopora spp. colonies area attached with nylon monofilament lariats. These colonies are derived from wild Pocillopora spp. from various reefs in Golfo Dulce. The colonies are fragmented by breaking off the tips of the branches either manually or using a diamond band saw to multiply the number of individual colonies through reproduction assisted fragmentation (Horoszowski-Fridman et al., 2015; Rinkevich, 2005). The nurseries are located between 3 and 5 m of depth below the water surface and anchored to the sea floor with rope and kept vertical with a floating buoy. The colonies in the nurseries are cleaned of algae each month and their health monitored (T. Villalobos, pers. comm., 2019). The experimental colonies were chosen at random from the coral nurseries before being transplanted at Punta Bejuco.
Study organisms: Pocillopora is a fast-growing genus (Darling et al., 2012) of branching scleractinian coral and an important reef builder in the ETP (Guzmán & Cortés, 1993). Pocillopora spp. can be categorized as pioneer species in the establishment of a coral reef due to the rapid growth rate and highly complex structure of corals in the genus (Clark & Edwards, 1994). Thus, Pocillopora is a popular genus for coral reef restoration (Boström-Einarsson et al., 2018). This coral is highly important to reef ecosystems due to its complex structure that permits the coral to harbor the greatest diversity of associated organisms of all coral genera (Stella et al., 2011). Pocillopora spp. in the ETP are particularly resilient to thermal stress (Manzello, 2010), possibly due to their propensity to harbor the more thermally tolerant Durusdinium glynnii (formerly ''Clade D”) zooxanthellae symbionts (Wham et al., 2017). Pocillopora may be thought of as a pioneer species and is a good candidate in that their branched skeletons create the conditions that allow other organisms to colonize an area where they previously would not be able (Clark & Edwards, 1994; Darling et al., 2012).
The benthic organisms and abiotic components of the sea floor in this study were categorized in operational taxonomic units (OTUs). These OTUs included: rubble, sand, bare calcium carbonate (CaCO3), the corals Pocillopora spp. and Porites spp., dead Pocillopora spp. (Dead Coral), unclassified sponge, the macroalga Caulerpa spp., Cyanobacteria (Cyano.), articulated coralline algae (ACA), crustose coralline algae (CCA), the bivalve mollusk Pinna rugosa and algal turf (Turf).
Transplantation site: Punta Bejuco reef (8° 43'39” N, 83° 24'29” W) (Fig. 1) was selected as the transplantation site for the Pocillopora spp. colonies that were cultivated in underwater coral nurseries near Playa Nicuesa of Golfo Dulce. Punta Bejuco was chosen as a site for transplantation because Pocillopora colonies that had been transplanted there prior to this study were healthy and growing (T. Villalobos, pers. comm., 2020). The species of corals used in this study were P. damicornis and P. elegans although they were not distinguished from one another in the experiment.
Pocillopora spp. currently has low rates of natural recruitment in Golfo Dulce (T. Villalobos pers. comm., 2020). Small Pocillopora colonies are more likely to die as a result of predation (Toh et al., 2014) and out-competition by algae (Kuffner et al., 2006). Punta Bejuco consists of three reef-built platforms with steeply sloping edges separated by channels of sand. The reef substrates are mostly made up of dead Pocillopora and Psammocora rubble. The predominant live coral species are Porites lobata (Cortés, 1990) and Porites evermanni (Boulay et al., 2014) that have an average coverage of 0.97 % of the sea floor (Cortés, 1990). Living colonies of Pocillopora that were not cultivated in coral nurseries are currently absent from Punta Bejuco, but the historical presence of Pocillopora can be inferred from coral skeletons (Cortés, 1990).
Out-planting of nursery-grown Pocillopora colonies and experimental design: A total of 30 Pocillopora spp. colonies were transplanted to the restoration site in Punta Bejuco in July 2019. The colonies were anchored with nylon zip-ties to steel nails, driven into the calcium carbonate substrate in a six by five colony rectangular arrangement with a distance of 30 cm from neighboring colonies. The rectangular transect extended 15 cm from the centroids of the fringing nails to measure 1.5 by 1.8 m in total (Fig. 4). To understand how the presence of the transplanted coral effected the benthic community, a control transect, devoid of coral transplants was designated and monitored using the same methods as the transplantation site. The control transect was located approximately 10m from the experimental patch at Punta Bejuco in a relatively flat region with a (visually) similar substrate composition. This transect was prepared in the same layout of 30 steel nails as the experimental transplanted transect, but contains no Pocillopora transplants (Fig. 4). The control patch contained no corals, living or dead over the course of the experiment. Likewise, the experimental patch never contained P. rugosa. Both the experimental and control patches contained negligible areas of sponge and sand.
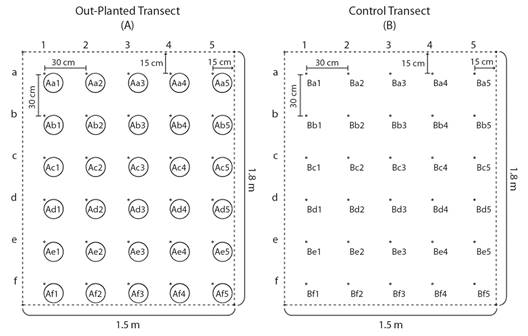
Fig. 4 The arrangement of experimental colonies and nails within transects on the sea floor. The black dots represent nails used as attachment points for the colonies or as markers of transect area and quadrat centers. The white circles represent Pocillopora spp. colonies. The dotted line represents the edge of the experimental and control transects. Each colony or nail position is given a procedural name so that it can be easily located.
Data Collection and Processing: Data from the 30 transplanted Pocillopora spp. colonies were recorded monthly from July 2019 to February 2020 using photography and observation.
Coral transplant survivorship and bleaching: The bleaching and survival status of each Pocillopora spp. colony was recorded each month. Coral could be categorized as bleached (and alive), dead or healthy (neither bleached nor dead). If a transplanted coral colony was visibly completely white yet free of macroalgae, cyanobacteria or loose tissues, the colony was recorded as bleached, having lost its symbiotic microalgae. If a colony had tissues visibly separated from the skeleton and/or the exposed skeleton was completely covered in macroalgae or cyanobacteria, the colony was recorded as dead as it no longer had any significant living, growing coral tissue.
In situ photography: To monitor the behavior of the community of sessile benthic organisms, a quadrat around each coral colony was photographed once each month from above using an Olympus TG-5 camera in a proprietary waterproof housing attached to a polyvinyl chloride (PVC) armature that held the camera 40 cm above the sea floor. The base of the armature formed a frame measuring 30 x 30 cm and was situated so that each anchor nail was positioned at the center of the base using virtually marked center point on the camera's monitor. For each photograph, the camera was set to ''under-water wide mode” with a zoom of 1.1 and set to shoot in RAW and JPEG image file formats. Each image was evaluated using the purpose-made photo analysis software, PhotoQuad (Trygonis & Sini, 2012) in order to calculate the coverage area of the transplanted coral colonies and benthic sessile organisms.
Photo Preparation: The JPEG images from the camera were loaded into Adobe Bridge where they were organized and renamed according to the month and colony number or position within the control transect. The images were corrected for ''fisheye” distortion from the wide-angle settings of the camera and optical properties of water in the Adobe Camera Raw image editor. To correct the distortion effectively, the correction was set to the point where the frame in the image was parallel with the gridlines in Camera Raw. The distortion correction was set to -31 for all of the images.
Quadrat assignment: Once loaded into the PhotoQuad application, distances in the distortion-corrected images of each coral were calibrated individually by selecting the ''calibrate” toggle and dragging the ends of the calibration bar to the bottom inner edge of the T-joints on the left and right of the PVC frame. The length of the calibration bar was then set to 30cm, the true distance between the supports of the PVC frame (Trygonis & Sini, 2012).
Coral area measurement: The quadrat of each image was assigned manually using the ''manual detection” toggle in the PhotoQuad application. The quadrat was defined by dragging the points supplied by the application to the inner corners of PVC frame to draw straight lines on the inner borders and around the joints of the PVC frame. The area of the coral colonies parallel to the substrate was measured with the ''region of interest” (ROI) method in PhotoQuad (Trygonis & Sini, 2012). In this method, the border of the living coral tissue (tissue that was neither visibly separated from the skeleton nor covered in macroalgae nor cyanobacteria) was traced and assigned as Pocillopora damicornis. The data from PhotoQuad were then exported as comma separated values for analysis using R (R Core Team, 2013). The ''SPECIES Area Total cm2 (sic.)” PhotoQuad output variable was used for analysis. This value was defined by the following formula:
Where: A pix is the number of pixels enclosed in the ROI; f clb is the calibration factor of the image based on the distance between the inside edges of the PVC frame in the image.
Benthic coverage measurement: The benthic community of the experimental transects was defined as the area of each encrusting organism or abiotic benthic component (OTU) within the quadrat. The area of each of the encrusting organisms was measured in PhotoQuad using the grid method (Trygonis & Sini, 2012). A grid was drawn over the quadrat with the horizontal grid field set to 150 pixels, equivalent to 2 250 pixels in each cell. Grid cells were selected when positioned over an encrusting organism. When the grid cells fell over the borders of the encrusting organisms, the fraction of the cell occupied by the encrusting organism was estimated and added to other occupied fractions of grid cells. When the fractions added up to one (1), a grid cell was selected. Selected grid cells were assigned to the OTU they covered. The data consisted of the area covered by the OTU in cm2, pixels, and the proportion of the quadrat occupied by that OTU. These data were imported into R for analysis (R Core Team, 2013).
Outliers: Two coral colonies extended beyond the PVC frame in the image. The data from the quadrats that contained these two colonies were excluded from the data set and analysis, but left in situ for other experiments. Data derived from in situ photography during the month of August was not included in the results because of malfunctions with the camera case that negatively affected the quality of the images.
Statistical analysis:
Growth rate: To estimate the rate of cover growth (cm2) of the corals over the eight months of the experiment, the average area (cm2) of each Pocillopora spp. colony were plotted each month with line-of-best-fit from linear regression. The slope of the line-of-best-fit was the areal growth rate of the colonies in cm2 month-1.
Comparison of experimental and control: Non-metric multidimensional scaling (nMDS) from Bray-Curtis similarity matrices was used to detect differences in the benthic community composition in the initial (July 2019) and final (February 2020) months in the experimental and control patches. These similarity matrices were built using the areas of each OTU in each of the photo-quadrats. The area values of OTUs in each patch were square root transformed to ensure that OTUs that occupied small proportions of each photoquadrat were would not ''drowned out” by the OTUs that occupied the majority of the photoquadrat area and could be compared on the same scale. These area values excluded Pocillopora spp. colonies, dead coral and artificial structures (anchor nails, tags and zip ties) to ensure that any differences in benthic community resulted from the presence of transplanted coral rather than simply detecting the growth of the transplants themselves. ANOSIM was performed on the nMDS data to quantify the separation and significance of any difference between initial and final benthic communities. SIMPER was performed to identify which OTUs contributed most to any differences that were detected. Construction, visualization and analysis of nMDS were performed in PRIMER 7 (Clarke & Gorley, 2015).
Temporal changes in sessile benthic community: Changes in the area of OTUs in each patch over time were estimated with linear regression in R (R Core Team, 2013). In the experimental patch, measurements from quadrats where the coral colony had died were analyzed separately from those that survived the eight months of the experiment. The quadrats were separated in this manner to see if temporal patterns in the abundance of benthic organisms were different when the coral transplant had died.
Results
Coral Survivorship and bleaching: In the eight months following transplantation (July 2019 - February 2020), five of the 30 (17 %) colonies died; three (10 %) died in October (four months from planting) and two more in November (7 %) (Table 1). The colonies experienced two episodes of bleaching: the first, in August and September 2019 (13 and 52 % of the original 30 colonies, respectively) and the second, in January and February 2020 (10 and 67 % of the original 30 colonies, respectively) (Table 1). At the end of the experiment, 5 colonies had died and of the remaining 25 living colonies, 20 were bleached and 5 were healthy.
Table 1 Total number of transplanted Pocillopora spp. colonies that were bleached, dead, or healthy (neither bleached nor dead) each month in the experimental patch in Golfo Dulce.
Month | Jul | Aug | Sep | Oct | Nov | Dec | Jan | Feb |
Total n | 30 | 24 | 29 | 30 | 30 | 30 | 29 | 30 |
Total Bleached | 0 | 3 | 15 | 0 | 0 | 0 | 3 | 20 |
Total Dead | 0 | 0 | 0 | 3 | 5 | 5 | 5 | 5 |
% Bleached | 0 % | 13 % | 52 % | 0 % | 0 % | 0 % | 10 % | 67 % |
% Dead | 0 % | 0 % | 0 % | 10 % | 17 % | 17 % | 17 % | 17 % |
% Healthy | 100 % | 87 % | 48 % | 90 % | 83 % | 83 % | 73 % | 16 % |
The number of colonies reported (n) varied from month to month due observational challenges.
Areal Growth: The average area of live Pocillopora spp. colonies increased significantly (linear regression: P < 0.05, R2 = 0.036) from an average of 119.4 ± 72.6 cm2 in July 2019 to 175.9 ± 113.0 cm2 in February 2020, a 68 % growth in area on average from initial to final months. The change in area of the Pocillopora spp. transplants was calculated to be 8.1 cm2 month-1 based on linear regression (R2 = 0.036, P < 0.05) (Fig. 5).
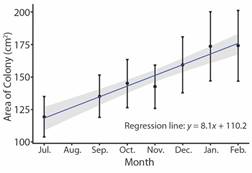
Fig. 5 Monthly average area (cm2) of transplanted Pocillopora spp. colonies in the transplanted site in Golfo Dulce. The plot includes standard deviation and line-of-best-fit from linear regression (line-of-best-fit equation: Area of colony = 110.2 + 8.1 (number of months following transplantation)); the gray area represents 95 % confidence intervals.
Change in the benthic community composition: The composition of the benthic community differed significantly between July 2019 and February 2020 in the experimental patch (ANOSIM; P < 0.01, R = 0.147) but not in the control patch (ANOSIM; P > 0.05, R = 0.031) (Fig. 6). Based on SIMPER analysis, the main contributors to the difference in the benthic community in the experimental patch after 8 months following coral transplantation were turf, CCA and Cyano.; contributing to 34, 27, and 37 % of the difference respectively (Table 2).
Table 2 Similarity of assemblages of benthic organisms in the experimental patch at Punta Bejuco, Golfo Dulce and OTUs with the greatest contribution as the result of SIMPER.
Group | Average Similarity | OTU | % Contribution |
February | 84.68 % | Turf | 90.42 |
July | 81.03 % | Turf | 90.47 |
February vs. July | 80.55 % | Turf | 33.67 |
CCA | 27.32 | ||
Cyano. | 26.39 |
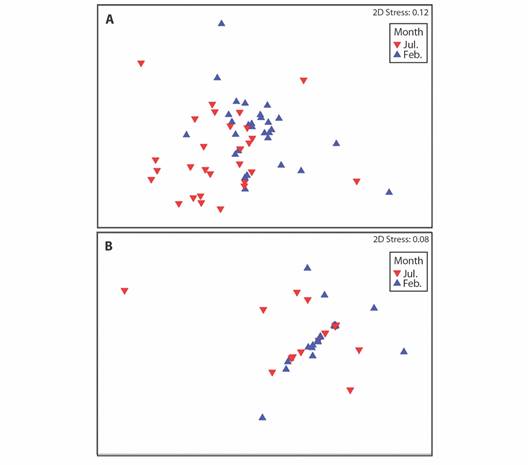
Fig. 6 Non-metric multidimensional scaling of the benthic communities in Punta Bejuco July 2019 versus February 2020. A. Communities in quadrats around Pocillopora spp. transplants in the experimental patch. B. Communities in quadrats around marking nails in the control patch.
Change in area of the benthic communities: In the experimental patch, the areas of turf, cyanobacteria, dead coral, Pocillopora spp. and CCA changed significantly over time (linear regression: P < 0.05) in the 24 quadrats where the colonies remained alive throughout the experiment. The coverage area of turf decreased by an average of 12.1 cm2 per month and the coverage of cyanobacteria, dead coral, and CCA increased by an average of 4.2, 1.0, and 3.1 cm2 per month respectively. In the four quadrats where corals died, the areas of Pocillopora spp., cyanobacteria, and dead coral changed significantly over time (linear regression: P < 0.05). The area of living Pocillopora spp. decreased by 26.3 cm2 per month and the areas of cyanobacteria and dead coral increased by 32.8 and 24.8 cm2 per month respectively (Fig. 7). In the control patch, the areas of OTUs remained constant and none changed significantly over time from their initial values.
Fig. 7. Average areas (cm2) of benthic sessile organisms over time in the quadrats where corals in the experimental patch A. survived for the length of the experiment or B. died before the end of the experiment and C. in the control patch at Punta Bejuco, Golfo Dulce.
The OTU that decreased the most in coverage was Pocillopora spp. in the ''dead” coral quadrats with a decrease from 23.55 to 2.52 % of the total coverage. In the ''dead” quadrats, the coverage of cyanobacteria and dead coral increased substantially as well from 0 to 20.20 % and 0 to 15.20 % respectively, taking up more of the quadrats than the initial coverage of the Pocillopora spp. transplants by 11.85 %. The average coverage of live Pocillopora spp. increased by 7.95 % from 16.89 to 24.84 % in ''live” coral quadrats. In the control patch, no OTU changed more than 1.5 % in average coverage of the quadrats (Table 3). No sand or sponge was present in the control, nor experimental patches.
Table 3 Average percent proportions (SD) of the quadrat area occupied by benthic organism OTUs in the initial (July) and final (February) months of data collection at Punta Bejuco, Golfo Dulce.
Status | n | Rubble | ACA | Bare CaCO3 | Pinna rugosa | Pocillopora spp. | Caulerpa spp. | Porites spp. | Turf | Cyano. | Dead Coral | CCA |
Live Jul Avg | 23 | 0.0(0.0) | 0.0(0.0) | 0.1(0.5) | 0.0(0.0) | 16.9(10.6) | 0.3(0.7) | 0.5(2.4) | 80.5(10.5) | 0.18(0.9) | 0.0(0.0) | 1.6(3.1) |
Live Feb Avg | 17 | 0.0(0.0) | 0.0(0.0) | 0.0(0.0) | 0.0(0.0) | 24.8(13.2) | 0.1(0.4) | 0.8(3.3) | 65.4(11.7) | 3.6(4.2) | 1.0(2.7) | 4.2(3.8) |
Dead Jul Avg | 4 | 0.0(0.0) | 0.0(0.0) | 0.0(0.0) | 0.0(0.0) | 23.6(7.3) | 0.0(0.0) | 0.0(0.0) | 73.5(6.0) | 0.0(0.0) | 0.0(0.0) | 2.9(2.5) |
Dead Feb Avg | 4 | 0.0(0.0) | 0.2(0.3) | 0.0(0.0) | 0.0(0.0) | 2.5(2.9) | 0.0(0.0) | 0.0(0.0) | 60.2(12.8) | 20.2(9.4) | 15.2(7.4) | 1.7(1.5) |
Cons Jul Avg | 21 | 0.2(0.9) | 0.0(0.0) | 1.1(3.7) | 0.1(0.3) | 0.0(0.0) | 0.0(0.2) | 0.0(0.0) | 97.1(6.2) | 0.0(0.0) | 0.0(0.0) | 1.5(2.7) |
Cons Feb Avg | 29 | 0.0(0.0) | 0.0(0.0) | 0.2(0.9) | 0.1(0.5) | 0.0(0.0) | 0.0(0.0) | 0.0(0.0) | 98.5(2.2) | 0.0(0.1) | 0.0(0.0) | 0.9(1.6) |
Averages are grouped by the patch and status of coral outplants as follows: Live - quadrats in the experimental patch where the coral survived the entire 8 months of the experiment; Dead - quadrats in the experimental patch where the outplants died at some point over the course of the experiment; Control - quadrats (Cons) in the control patch.
Discussion
Coral health and survivorship: Over the course of eight months of this exploratory study, there were two bleaching events and 17 % of the transplanted colonies died. It is unknown whether a similar proportion of colonies died in the nurseries where the corals were grown. The first event occurred in August and September 2019. High rainfall in these two months typically causes significant run-off and sedimentation in the gulf as sediment is washed in from the surrounding rivers that feed into Golfo Dulce and the water was visibly turbid during field operations. This sedimentation was one of the primary causes for the loss of Pocillopora spp. colonies in the Gulf in the 1960's-80's (Cortés, 1990). Sediment settles on the coral colonies, blocking sunlight, and stimulating the production of excess mucus, causing stress and bleaching (Philipp & Fabricius, 2003). Alternatively, bleaching could have occurred as the result of the stress of the transplantation process, an event observed in other restoration efforts (Cunning et al., 2014; Forrester et al., 2012).
The second bleaching event occurred in January and February 2020. These months correspond to the dry season when water temperatures are typically elevated and sea surface temperature at the study site averaged 31.2 °C (Fig. 3) during this period of the experimental timeframe. Although temperature tolerance can vary significantly within corals of the same species from different regions (Coles et al., 1977), corals in the ETP have been reported to bleach when sea surface temperatures exceed 30 ºC (Podestá & Glynn, 1997). In general, corals often do not recover following severe bleaching. The majority of the bleached corals at the transplantation site regained some of their original color and grew significantly although none of the coral transplants regained the color they had in the first month of the experiment immediately following transplantation (personal observation).
Given that many of the colonies did not survive in the timeframe of the experiment, a different location and/or different environmental conditions should be considered for future restoration efforts in Golfo Dulce. The relatively shallow depth of the corals at 3 m may have offered little protection for the corals from higher temperatures near the water surface, or higher light intensity; a problem encountered in other coral transplantation efforts (Lohr et al., 2016). Tides in Golfo Dulce can vary between 2 m and 4 m in the inner part of the gulf (Svendsen et al., 2006). The tidal activity at Punta Bejuco was not recorded in this study and it is possible that tides could have exacerbated the colonies'exposure to solar radiation. It is generally recommended to transplant corals at the depth they are encountered naturally in the area. In Golfo Dulce, Pocillopora spp. is encountered from 3 to 5 m below the surface (Cortés, 1990) although most of the native colonies that provided fragments for the nursery were deeper, up to 8 m below the surface (J. Kleypas, pers. comm., 2020.). As of October 2020, almost all of the nearby coral transplants that were not part of the experiment and which were positioned deeper at 5.6m survived the bleaching event in January and February 2020 (T. Villalobos, pers. comm., 2020).
Coral growth: Despite the two bleaching events and non-ideal transplantation site, the average area of the corals grows significantly over the course of the experiment. Other research suggests that the average sizes of the Pocillopora spp. colonies in the experimental patch were in a range of sizes that are likely to grow but less likely to survive over the course of a year (Ishida-Castañeda et al., 2020; Kodera et al., 2020; Tortolero-Langarica et al., 2020). This practical information could be very useful for determining the size of coral transplants to use for the purposes of restoration.
Temporal change in the sessile benthic community following transplantation: The increase in area of live coral, CCA and Cyanobacteria and decrease in area of algal turf in the experimental patch following the transplantation of Pocillopora spp. colonies suggests that the coral colonies affected the succession of the sessile benthic community. Corals, especially structurally complex, highly branched forms such as Pocillopora, attract associated fauna (Lewis, 1986, Smith et al., 2010). Herbivorous coral associates contribute to the health and resilience of coral reefs by preventing algal succession from progressing from a state dominated by early successional CCA which do not compete with corals and fleshy, leafy algae which impede growth of corals (Burkepile & Hay, 2010; Hixon & Bronstoff, 1996; Humphries et al., 2014).
The lack of change in the coverage of sessile benthic organisms in the control patch further supports the conclusion that coral had an effect on the trajectories of succession of the benthic community. Differing trends in the area of turf between experimental and control patches may be the result of greater herbivory in the experimental patch from herbivores attracted to the structural complexity of the transplanted coral (Lewis, 1986; Smith et al., 2010). Conversely, the area of algal turf in the control patch remained constant and high because it is less structurally complex and thus attracts fewer herbivores (Lewis, 1986).
Over the entire experimental patch and particularly in quadrats where corals had died, there was a strong increase in the coverage of cyanobacteria. In quadrats where the coral transplants had died, the area of cyanobacteria, along with dead coral tissue had almost entirely replaced live coral by the end of the experiment. Cyanobacteria flourish in high temperatures and could have contributed to the ultimate death of the coral colonies following bleaching by smothering the weakened coral and benthos (Ford et al., 2018; Kuffner et al., 2006). Cyanobacteria may produce toxins that could deter herbivores that would otherwise control macroalgal populations (Ford et al., 2018; Leão et al., 2012). It is important to understand the characteristics and processes that occur when coral colonies die because it can provide clues and warnings when monitoring the health of reefs and implementing management responses (Goatley et al., 2016). This could be a problem for future coral transplantation in the zone as the environmental conditions in the shallow reefs of Golfo Dulce could favor the growth of Cyanobacteria.
The decrease in algal turf in the experimental patch from ~80 to ~65 % is comparable in magnitude to the decrease in turf coverage at the coral reefs of Coco Island following 20 years of reef recovery after the 1982-83 ENSO event (Guzmán & Cortés, 2007). Coral coverage recovered from an average of 2.99 ± 0.25 % to 14.87 ± 6.78 % (average ± SE) % at Coco Island, a similar magnitude to this study with the experimental patch containing a negligible coverage of coral before transplantation and upwards of 24.8 % coverage eight months following transplantation. Although the study at Coco Island was conducted at a much larger spatial and temporal scales, and under much higher conservation standards than in Golfo Dulce, similar processes may account for the decrease in turf coverage albeit over a much shorter time period in this study. Restoration, together with other coastal management actions, could increase the survivorship of the corals and the recovery of the reef and their ecological functions.
Although one of the shortest times reported for succession to reach a climax in a coral reef ecosystem is approximately ten months (Matsuda, 1989), the process of succession on coral reefs generally takes much longer than the timeframe of this experiment (Karlson, 1999). Over a longer timeframe, further evidence of succession and its associated ecological processes could be detected. Also, following the ultimate bleaching and death of the coral transplants, a longer experiment could explore how succession of the sessile benthic community changes following the death of corals, a process that was beginning to occur with the increase in cyanobacteria. Coral-algae interactions also vary seasonally due to the complex interplay between encrusting organisms, grazing animals, eroding animals, and the environmental variables that effect their health, populations and behaviors (Brown et al., 2019; Doropoulos et al., 2016; Fong et al., 2016; Muthukrishnan et al., 2016). As this experiment lasted less than a year, a longer experiment where seasonal cycles repeat could help discern what changes in the benthic community are due to seasonal shifts versus succession.
Photo-quadrats were positioned directly over the transplanted coral colonies in the experimental patch, resulting in a bias toward greater coral coverage. An experiment using larger quadrats could capture the dynamics of the sessile benthic community at the scale of a reef rather than a colony. The differences observed in the dynamics of the benthic communities in the experimental and control patches may be the result of methodological artifacts. The observed decrease in turf area may be the result of the coral transplants taking up more area of the photoquadrats as their branches grow up and over the sea floor. The branches could be covering turf in the image that may still be present around the bases of the colonies and thus, reduce their apparent area.
Ecological succession is intrinsically important to restoration efforts. Environmental and ecological conditions can direct the succession of a reef towards alternate stable states (Smith et al., 2010). Under current environmental conditions, many coral reefs tend to follow a successional trajectory that favors the dominance of algae and other organisms that inhibit the recruitment and growth of corals (Pandolfi et al., 2005; Rinkevich, 2005). Coral restoration through transplantation is an increasingly common intervention that is used to accelerate the process of succession to favor the growth and persistence of coral reefs.
The transplantation of Pocillopora spp. colonies influenced the succession of a sessile benthic community of a restoration site in Golfo Dulce, as evidenced by the growth of organisms that promote coral growth and the decline of those that compete for space and resources with corals. Additionally, following transplantation, these shifts in the sea floor composition favored a successional trajectory that promotes the growth of coral and may improve the reefs'resilience to future disturbance and the acceleration of ecosystem recuperation. Transplantation and growth of Pocillopora spp. colonies corals modified the local ecosystem around the colonies to promote further coral reef growth and development. These results suggest that the transplantation of Pocillopora spp. could improve the outcomes of coral restoration efforts by changing the trajectory of succession. However, this must be carried out in conjunction with watershed management to reduce sedimentation in the area and associated stress to the corals. A similar experiment conducted over longer temporal and larger spatial scales may reveal if successional trends continue or change.
Ethical statement: the authors declare that they all agree with this publication and made significant contributions; that there is no conflict of interest of any kind; and that we followed all pertinent ethical and legal procedures and requirements. All financial sources are fully and clearly stated in the acknowledgements section. A signed document has been filed in the journal archives.