Introduction
Tlaloc hildebrandi (Miller, 1950), an endemic fish of Chiapas, México (Velázquez-Velázquez & Schmitter-Soto, 2004), is a small-bodied profundulid fish (maximum size: 110.66 mm standard length (Domínguez-Cisneros et al., 2017). Also known as the “escamudo de San Cristóbal” or the “Chiapas killifish”, it has been cataloged as an Endangered species (P) under Mexican legislation (NOM-059-SEMARNAT-2010) (Secretaría de Medio Ambiente y Recursos Naturales, 2019) and also in the International Union for Conservation of Nature (IUCN) Red List of Threatened Species (EN), due to its restricted distribution, invasive species, and habitat vulnerability (Schmitter-Soto & Vega-Cendejas, 2019).
This species is the only native fish to inhabit the mountains of Chiapas, at altitudes ranging from 2 110 to 2 360 m.a.s.l. Tlaloc hildebrandi inhabits the Fogotico River and its tributaries and in the upper reaches of the Grijalva-Usumacinta system, in Chiapas, México (Beltrán-López et al., 2021; Domínguez-Cisneros et al., 2017; Velázquez-Velázquez & Schmitter-Soto, 2004). It exhibits a restricted extent of occurrence, because the number of locations where this species occurs is limited to three, based on the recognition of at least three evolutionarily significant units (Beltrán-López et al., 2021; Velázquez-Velázquez et al., 2016).
The morphology of the mouth and digestive tract of the fishes, together with the identification of the food content in the intestine, allows the interpretation of the trophic biology of the species (Karachle & Stergiou, 2010; Keast & Webb, 1966; Wootton, 1998). Likewise, the analysis and descriptions of its components contribute to understanding its functions and its role in the life history of the organism (Drewe et al., 2004; Hale, 1965; Kapoor et al., 1976).
The digestive system of teleost fishes is composed of the digestive tract and its associated glands (Moyle & Cech, 2000). The digestive tract begins at the mouth and finishes at the anus and may be divided into four general sections based on functional and histological criteria: bucco-pharyngeal cavity, esophagus, stomach, and intestine. The stomach is the most highly diversified region of the gut in teleost fishes and has also undergone a number of independent secondary losses, with stomach-less fishes accounting for approximately 20 % of teleost species (Wilson & Castro, 2011).
Among the few studies on the anatomy of the digestive system of the Mexican Cyprinodontiformes are those of Kobelkowsky (2005) on Goodeidae and Hernández et al., (2009) on Rivulidae, Fundulidae, Poeciliidae, and Cyprinodontidae. Studies devoted to the description of the digestive system of Profundulidae are still lacking. Trophic morphology and food habit studies of fishes are necessary to understand the role they play in the trophic food web (Gerking, 1994). These data are essential to account as an important biotic factor when making conservation and management decisions. Understanding the relationship between diet and morphological traits is critically relevant to the successful management of threatened species such as T. hildebrnadi. The specific aims of this study are: 1) to describe the anatomy of the digestive system and 2) to provide a quantitative description of the diet of T. hildebrandi. In order to establish their feeding habits, we take into account the relationship between diet and trophic morphology.
Materials and methods
Material examined: Specimens deposited in the Fish Collection of the Zoology Museum of the University of Sciences and Arts of Chiapas were analyzed (MZ-UNICACH); twenty individuals of both sexes between 44.63-102.06 mm standard length (SL) were used for anatomical analysis: Tlaloc hildebrandi; MZ-UNICACH 4301, 3; MZ-UNICACH 4346, 4; MZ-UNICACH 4296, 4; MZ-UNICACH 4331, 6; MZ-UNICACH 4348,1; MZ-UNICACH 4330,1; MZ-UNICACH 4328, 3. All specimens from the Amarillo River, in the municipality of San Cristóbal de Las Casas, Chiapas, México. Fish were fixed in a 10 % formalin solution and subsequent preserved in a 70 % ethanol solution.
In order to expose the visceral musculature and the visceral skeleton, manual dissection and disarticulation technique were used (Bemis et al., 2004; Kobelkowsky, 2005). The external mouth morphology was described. In order to expose the visceral musculature, the skin from the cephalic region was removed. After the analysis of these structures to allow the observation of the mandibular apparatus, palatine series, the opercular series, hyoid apparatus and branchial apparatus, the visceral muscles were manually removed. The visceral cavity was exposed, removing the body wall. In order to examine the branchial musculature, the pectoral girdle was removed. Osteological terminology follows Gregory (1933), Parenti (1981) and Costa (2006); the terminology of the visceral musculature follows Winterbottom (1973). The illustrations were done by means of a camera lucida mounted in a stereoscopic microscope Wild M3Z.
The gut content of 60 adult specimens of both sexes between 24.8-114.2 mm standard length (SL) were used for diet analysis. In each case, the food content of the anterior region of the intestine was squashed on a graduated slide to a uniform depth and the area of the squash was measured (Castillo-Rivera et al.,1996), according to the method for measuring small stomach volumes (Hyslop, 1980). Gut contents were sorted and identified to the lowest taxonomical level using standard taxonomic keys (Merrit & Cummins, 1984; Needham & Needham, 1962). Composition of gut content was interpreted using two indices: percent frequency of occurrence (% F) that shows the percentage of guts that had a certain prey type present. Percent numerical importance (% N) gives the proportion of a prey group compared to the total number of prey items examined for each species. These two indices were also used to generate an index of relative importance (IRI) (McCune & Grace, 2002). These values were totaled for all items and a %IRI is presented.
Intestinal index (Ii) was calculated using the equation of Nikolski (1963); which defines this index as the ratio of the length of the intestine to the standard length of the fish. According to this index, fish can be classified into three food categories: carnivores Ii < 1; omnivores Ii between 1 and 2; herbivores Ii > 2.
Results
Visceral skeleton: Jaws: The mouth of T. hildebrandi has strong jaws, with lower jaw strongly upwards (Fig. 1A). Premaxilla and maxilla form the upper jaw, while dentary, anguloarticular, retroarticular and coronomeckelian form the lower jaw (Fig. 1B). The coronoid cartilage is located on the external face of the coronoid processes of the dentary and anguloarticular (Fig. 1B, Fig. 2A, Fig. 2D). The premaxilla has an S shape and a short ascending process with blunt border. The buccal border of the premaxilla carries an external row of slim conical, medium-sized teeth, and a numerous group of smaller internal teeth. The rest of the premaxilla is wide and abruptly descends to form a wide notch on its posterior margin where it contacts the coronoid cartilage (Fig. 2A, Fig. 2B).
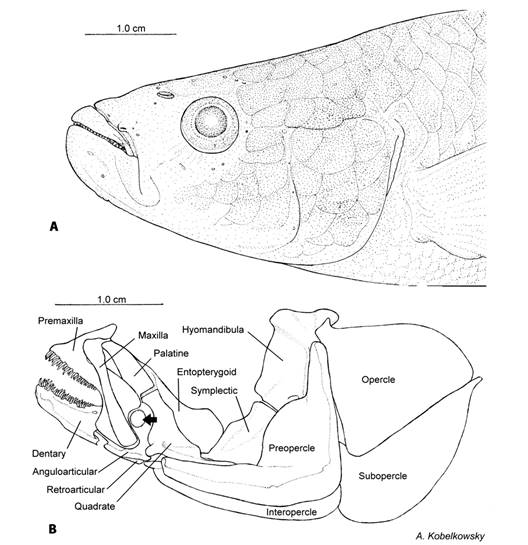
Fig. 1 Cephalic region of Tlaloc hildebrandi. A. Lateral view. B. Visceral skeleton: mandibular skeleton, palatine series, mandibular suspension and opercular series. Arrow point to coronoid cartilage.
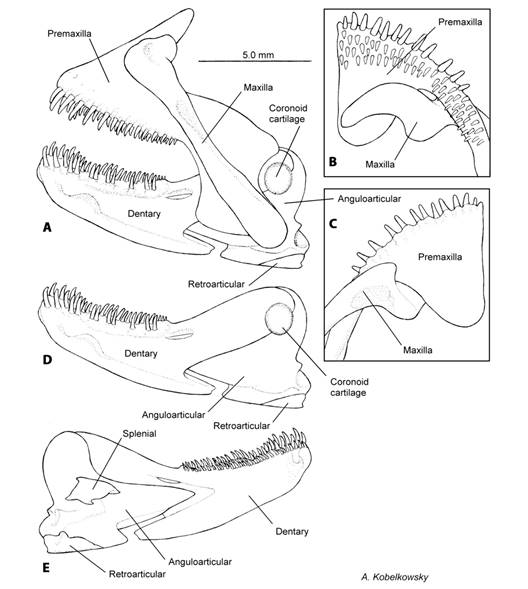
Fig. 2 Mandibular skeleton of Tlaloc hildebrandi. A. Left lateral view of upper and lower jaws. B. Ventral view of the upper jaw. C. Dorsal view of the upper jaw. D. Left lateral view of the lower jaw. E. Medial view of the lower jaw.
The maxilla is an elongate and narrow bone, with the anterior margin contacting the superior edge of the premaxilla (Fig. 2A, Fig. 2B, Fig. 2C). The external surface of the maxilla has a concave area where it joins the anterior border of the palatine (Fig. 1B).
The coronoid process of dentary is elongate and narrow, ending in a blunt angle, joined to the external surface of the anguloarticular. Externally on the two bones it is the coronoid (Fig. 2D). The dentary also has a short ventral process in the lower side of the bone. The anterior-dorsal margin of the dentary has two rows of teeth, a single outer row of medium sized-teeth, and several internal rows of smaller teeth. The dentary has a branch of the lateral line system (Fig. 2A, Fig. 2D).
The anguloarticular forms a large triangular process that inserts in the dentary anteriorly, and forms the coronoid process joined dorsally with the dentary (Fig. 2E). In its postero-inferior angle the anguloarticular has a fossa that joins with the condyle of the quadrate (Fig. 1B, Fig. 2D). The retroarticular is a small bone located posteroventral to the anguloarticular (Fig. 2D, Fig. 2E). The coronomeckelian is a small and triangular bone that firmly joins the medial surface of the anguloarticular (Fig. 2E).
Palatine series: The palatine series is formed by the palatine and the entopterygoid (Fig. 1B). The palatine is flat and straight, and joined anteriorly to the maxilla.
The palatine makes contact through a cartilaginous portion of the quadrate, and medially with parts of the entopterygoid. The entopterygoid is thin; its internal border is concave and forms an anterior process that covers parts of the internal surface of the palatine, contacting the quadrate medially and the symplectic posteriorly.
Mandibular suspension: The mandibular suspensorium is formed by the hyomandibula, symplectic and quadrate (Fig. 1B). The hyomandibula articulates by means of condyles with the sphenotic, pterotic, and prootic bones of the neurocranium, and the opercle and preopercle bones of the opercular series. The anteromedial hyomandibula margin is undulated and approximately vertical, and contacts the lateral surfaces of the preopercle and symplectic. The symplectic is broad and obliquely oriented, with a robust medial section, and the ventral margin is acute and located between the entopterygoid and the quadrate (Fig. 1B). The quadrate is relatively large and irregular-shaped, the anterior portion is truncate and enlarged anterodorsally, the posterior portion has a thin retroarticular process connected with the medial surface of the preopercle.
Opercle: The opercular series is formed by the preopercle, opercle, interopercle and subopercle bones (Fig. 1B). The preopercle is L-shaped, with a large median shelf wrapping around the symplectic, a convex anterior margin, and a laterosensory canal groove on its posterior margin. The opercle is triangular, with a convex superior edge, a truncate anterodorsal process, and a straight posteroventral margin. The subopercle is large with an acute anterior end. The interopercle is elongate, with a pointed anterior end, and a ligamentous attachment to the retroarticular.
Branchial apparatus: The branchial apparatus is formed by two basibranchial, three hypobranchial, five ceratobranchial, four epibranchial, and three pharyngobranchial bones (Fig. 3A, Fig. 3B, Fig. 3C, Fig. 3D, Fig. 3E). The articular faces of the first two basibranchials contact the hypobranchials. The hypobranchials are rectangular-shaped and gradually become smaller posteriorly (Fig. 3B, Fig. 3D). Ceratobranchials 1-4 (Fig. 3D) have a groove on their lateral surface to accommodate the branchial arteries and are the largest bones, of the branchial apparatus. The fourth ceratobranchial bone has teeth in its dorsal surface. The fifth ceratobranchial or lower pharyngeal bone is robust with its anterior extreme convex and the posterior extreme truncate, ventrally it forms a condyle where it joins to the pharyngoclavicularis externus muscle. Its dorsal surface is covered by teeth (Fig. 3D, Fig. 3E).
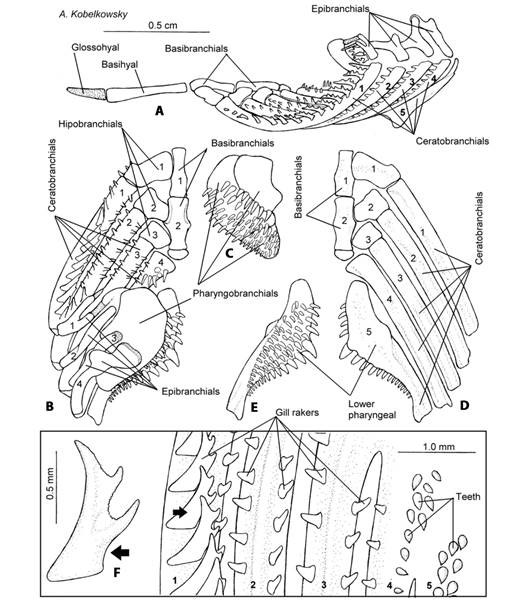
Fig. 3 Branchial skeleton of Tlaloc hildebrandi. A. Left lateral view of the branchial apparatus. B. Dorsal view of the branchial apparatus. C. Ventral view of the upper pharyngeal bones. D. Ventral view of the branchial apparatus. E. Dorsal view of the lower pharyngeal bone. F. Lateral view of a gill raker on the external row of the first branchial arch; numbers 1-5, ceratobranquiales.
Epibranchials (Fig. 3A, Fig. 3B) are small bones and joined by the levator externalis muscle. The first epibranchial is bifurcated with each branch contacting a cartilage, and lacking a dorsal process. Epibranchials two and four possess a dorsal process. The dorsal process of the third epibranchial joins the anterior process of the fourth epibranchial.
The pharyngobranchials or upper pharyngeal bones (Fig. 3C) are three flat elements of the branchial apparatus, and have numerous teeth on their ventral surfaces. The second pharyngobranchial bone is the largest and the third pharyngobranchial is the smallest, which unites posteriorly with the retractor dorsalis muscle.
The anterior arm of the first branchial arch of T. hildebrandi has 13 to 15 gill rakers. The gill rakers of this species do not have teeth and are found on both margins of branchial arches 1 to 3, with the exception of the internal surface of branchial arch 4 (Fig. 3F). The gill rakers are triangular-shaped, large and thin, with three cusps on the internal border of the first arch. In contrast, they are triangular-shaped, small, and without cusps in the rest of the branchial arches.
The teeth of T. hildebrandi are conical and slightly curved backwards. Teeth are found on the following bones: premaxilla, dentary, ceratobranchial 4, and pharyngobranchials 1 to 3. The teeth in the premaxilla and dentary are arranged in two groups. The first row is a single group of large teeth with about the same size; the second row lies behind the first, and has numerous irregularly arranged and smaller teeth (Fig. 2A, Fig. 2B).
The teeth of ceratobranchial 4 are located (Fig. 3B, Fig. 3F) on the anterior portion of the bone. The teeth of the lower pharyngeal bones are larger on the medial border and gradually become smaller at the external edge (Fig. 3E). The teeth of pharyngobranchial 1 are confined to its posterior edge; teeth cover the majority of the ventral surface of pharyngobranchial 2, and the entire surface of pharyngobranchial 3 (Fig. 3C).
Hyoid apparatus: The hyoid apparatus is formed by the glossohyal, basihyal, urohyal, dorsal and ventral hypohyals, ceratohyal, epihyal, interhyal, and six branchiostegal-ray bones (Fig. 4A). The basihyal is enlarged and joins anteriorly to the glossohyal. The urohyal is enlarged, laterally compressed, triangular in a lateral view, and the posterior portion is convex (Fig. 4B). Dorsal hypohyal absent; anterior extension of anterior ceratohyal ventral to ventral hypohyal. The ceratohyal is elongate and narrow in the middle where it joins the two branchiostegal rays and posteriorly joins three branchiostegal rays (Fig. 4A).
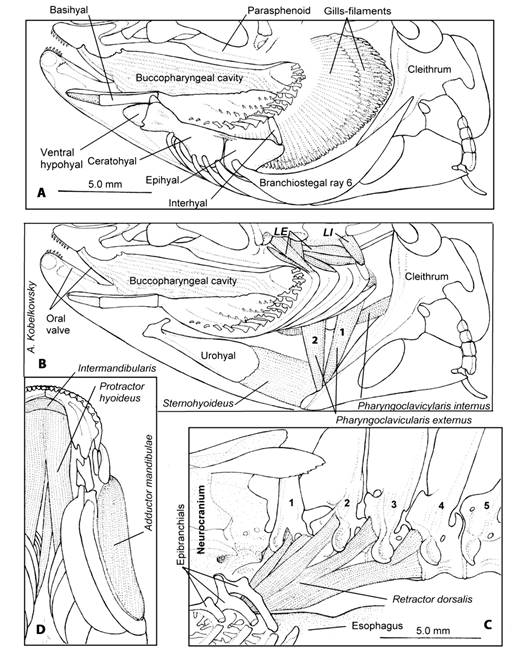
Fig. 4 Hyoid and branchial musculature of Tlaloc hildebrandi. A. Lateral left view of the buccopharyngeal cavity and hyoid apparatus. B. Lateral left view of the branchial and hyoid musculature; LE, levatores externi, LI, levatore interni; 1-2, pharyngoclavicularis externus C. Lateral view of the retractor dorsalis muscle; numbers 1-5, precaudal vertebrae D. Ventral view of the gular region.
The epihyal is triangular-shaped and articulates anteriorly with the ceratohyal by a suture, and it also contacts the sixth branchiostegal ray. The epihyal joins the interhyal at its anteroposterior margin. The interhyal is cylindrical-shaped, thin and contacts the preopercle by means of a cartilage.
Visceral musculature: The visceral musculature of T. hildebrandi is organized into mandibular, hyoid and branchial arches. The most complex mandibular muscle is the adductor mandibulae (Fig. 5) which is divided into four sections: A1, A2, A3, and Aw (Fig. 5C). Its origin is from several areas of the mandibular suspension and preopercular, its insertion is on both the upper and lower jaws. The levator arcus palatini muscle originates from the lateral process of the sphenotic and inserts on the lateral surface of the hyomandibula. The adductor arcus palatini muscle originates from the parasphenoid and it inserts on the entopterygoid and palatine (Fig. 5A, Fig. 5C). The intermandibularis muscle (Fig. 4D) is narrow and inserts on the internal surface of both dentaries near the mandibular symphysis.
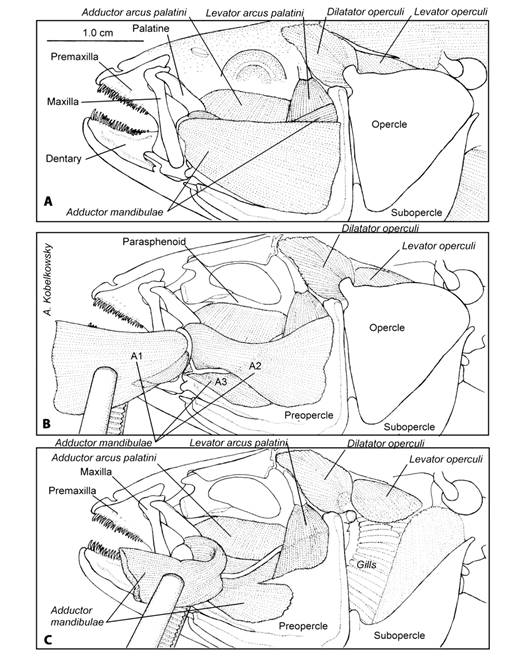
Fig. 5 Mandibular musculature of Tlaloc hildebrandi. A. Left lateral view of the cephalic region. B. Adductor mandibulae muscle projecting section A1, A2 and A3 C. Mandibular and opercular musculature.
The protractor hyoideus muscle (Fig. 4D) originates from the dentary and inserts on the ceratohyal, reaching the third branchiostegals. The retractor dorsalis muscle (Fig. 4C) is formed by several sections that originate from precaudal vertebrae 1-4 and inserts on the posterior border of the third pharyngobranchial. The dilatator operculi muscle originates from the sphenotic process and inserts on the opercular anterodorsal process. The levator operculi muscle originates from the pterotic and inserts on the internal surface of the opercular, near its superior margin (Fig. 5B, Fig. 5C). The levatores externi muscles originate from the sphenotic and insert on all four epibranchial bones. The levatores interni muscles are situated medially to the levatores externi (Fig. 4B). The sternohyoideus muscle (Fig. 4B) originate from the cleithrum and inserts on the posterior border of the urohyal. The pharyngoclavicularis externus muscle (Fig. 4B) is divided into two sections (1 and 2) and originates from the anteroventral portion of the cleithrum and inserts on the ventral surface of the lower pharyngeal bone. The pharyngoclavicularis internus muscle (Fig. 4B) originates from the anterior surface of the cleithrum and inserts on the ventral surface of the lower pharyngeal bone.
Visceral cavity: The visceral cavity of T. hildebrandi (Fig. 6A) is wide and enclosed by the precaudal vertebrae, the first hemal arch, the anal fin musculature, the infracarinalis media muscle, the pelvic girdle, infracarinales anteriores muscles, the pectoral girdle, pleural ribs, epipleural ribs and axial musculature. The liver and gas bladder occupy about 50 % of the visceral cavity; the pancreatic tissue gradually invades the liver along the branches of the portal vein. The combined hepatic and pancreatic tissue are collectively called the hepatopancreas. The digestive tube, spleen and gonads occupy the remaining space. The gas bladder does not have a pneumatic duct and makes contact with the dorsal surfaces of the stomach and gonads. The gonads develop between the gas bladder and the intestine. The female genital opening and the male urogenital opening are located immediately behind the anus.
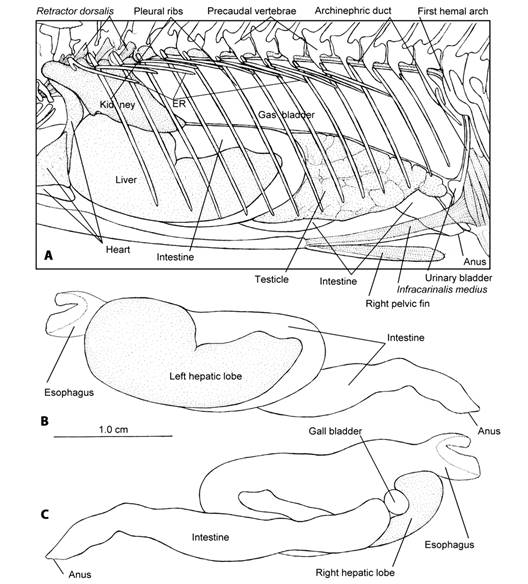
Fig. 6 Visceral cavity of Tlaloc hildebrandi. A. Left lateral view; ER, epipleural ribs B. Left lateral view of the digestive tube and liver. C. Right lateral view of the digestive tube and liver.
The digestive tube of T. hildebrandi is formed by the esophagus and the intestine. The stomach is missing (Fig. 6B, Fig. 6C). The esophagus begins from the upper and lower pharyngeal bones and it is funnel-like (Fig. 7A, Fig. 7B); numerous thin longitudinal folds and grooves form the lining. The first third of intestine is wide and irregular folds form the lining (Fig. 7B). Between the esophagus and intestine is the connection of the common biliary duct, being the feature indicating the absence of the stomach.
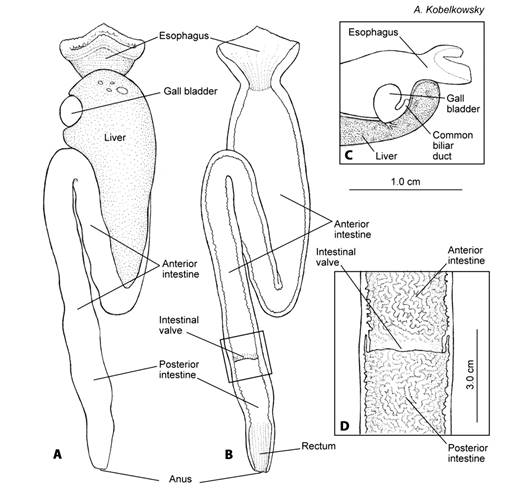
Fig. 7 Digestive tube of Tlaloc hildebrandi. A. Ventral view. B. Ventral view of the frontal section of the digestive tube. C. Section of the intestines with the intestinal valve in ventral view. D. The intestinal valve divides the intestine in anterior (prevalvular) and posterior (postvalvular) intestine.
The intestine is relatively short, and displays a single fold located below the anterior portion of intestine. The intestinal index was determined as 0.3 (0.24-0.45). The rest of the intestine is straight and oriented posteriorly (Fig. 6B, Fig. 6C, Fig. 7A, Fig. 7B). The surface of the intestinal lumen is covered by folds and shallow grooves in a reticulate pattern. An intestinal valve is posteriorly situated, dividing the intestines into anterior and posterior portions (Fig. 7B, Fig. 7C). The last portion of the posterior intestine is the rectum, which may be recognized by the presence of longitudinal and shallow grooves and folds.
The liver has two lobes; the left lobe is always larger and covers a greater portion of the stomach on its left side, and the initial part of the intestine (Fig. 6B). The right lobe of the liver is smaller and forms a groove to accommodate the gall bladder. The gall bladder is spherical-shaped (Fig. 6C).
Diet: Of the total analyzed intestines, 11.7 % (7) was found empty and 88.3 % (53) were found with food. Eighteen trophic categories were recognized, being insects and crustaceans the two largest taxa, together account for 97 % of dominance (IVI). Organic matter (MON) and unidentified fish scales and insects (INI) were determined as trophic categories. According to Table 1, insects of the order Diptera, Ephemeroptera, Hymenoptera and Coleoptera were the dominant prey items (IVI = 66.6 %) of the Tlaloc hildebrandi diet; ostracods (13 % IVI) were recognized in the diet as a secondary prey category and the rest of the prey components as accidental (less than 10 %). The insects’ larvae of the Chironomidae family (Diptera), accounted for 53 % (IVI) of the Chiapas killifish diet (Table 1).
Table 1 Diet composition of Tlaloc hildebrandi.
Food type | Diet composition (%) | ||
% N | % FO | % IRI | |
Insects | 66.6 | ||
Diptera | 55.9 | 50.4 | 53.1 |
Chironomidae | |||
Chaoboridae | 2.4 | 5.0 | 3.7 |
Simulidae | 1.7 | 1.4 | 1.6 |
Dixidae | 0.9 | 1.4 | 1.2 |
Syrphydae | 1.6 | 1.4 | 1.5 |
Stratiomyidae | 0.2 | 1.4 | 0.8 |
Ephemeroptera | 3.4 | 4.3 | 3.8 |
Hymenoptera | 0.2 | 0.7 | 0.4 |
Coleoptera | 0.3 | 0.7 | 0.5 |
Crustaceans | 31.3 | ||
Ostracoda | 14.0 | 13.5 | 13.7 |
Amphipoda | 6.2 | 5.7 | 5.9 |
Isopoda | 9.6 | 8.5 | 9.1 |
Cladocera | 2.0 | 1.4 | 1.7 |
Copepoda | 0.2 | 1.4 | 0.8 |
Diplopoda | 0.4 | 0.7 | 0.6 |
INI | 0.6 | 0.7 | 0.7 |
MON | 0.3 | 0.7 | 0.5 |
Fish scales | 0.1 | 0.7 | 0.4 |
Bold value indicates the sum of each diet group (% IRI).
Discussion
The anatomy of the digestive system of Tlaloc hildebrandi largely corresponds to that of teleost fishes. However, T. hildebrandi has the “coronoid cartilage”, registered here for the first time; the close location of this cartilage to the posterior notch of the premaxillary, probably limits the backward movement of jaws. The anterior extension of the quadrate functionally compensates for the absence of an ectopterygoid, which is a character of Cyprinodontiformes (Parenti, 1981).
The number of bones that carry teeth in T. hildebrandi is relatively reduced compared with other teleosts (Wainwright, 1989), including those of the gill rakers. However, T. hildebrandi exhibits teeth on fourth ceratobranchial bone.
The number of gill rakers in the first-branchial arch is within the range of other profundulid species (Domínguez-Cisneros et al., 2023; Nelson et al., 2016). However, the presence of large, thin and tricuspid gill rakers on the first-branchial arch is a new observation in T. hildebrandi.
The adductor mandibulae muscle is composed of four sections as in many other teleosts (Kenaley et al., 2019; Winterbottom, 1973); the large volume of section A1 suggests a strong movement of jaws during mouth closure.
The relative high number of sections of the retractor dorsalis muscle suggests an effective swallowing in contrast to what happens in other teleosts (Wainwright, 1989).
The pharyngoclavicularis externus in T. hildebrandi is formed by two elements (1 and 2), but in many teleost fishes this muscle has a single element, as in G. atripinnis (Kobelkowsky, 2005).
The organography of the visceral cavity of T. hildebrandi is the typical of that of teleost fishes, as illustrated in Chirostoma estor (Kobelkowsky & Figueroa, 2018) and Eugerres mexicanus (Kobelkowsky & Terán-Martínez, 2020).
The absence of the stomach in T. hildebrandi observed in the present study coincides with that of Hale (1965) in Poecilia reticulata and that of Wilson and Castro (2011) in Cyprinodontiformes. The thickening of the first portion of the intestine in Goodea atripinnis (Kobelkowsky, 2005) is misnamed as stomach. The character denoting the absence of the stomach is the communication of the common bile duct to the digestive tube at the junction of the esophagus with the intestine.
Gut type and modes of intestinal morphology have been used for the categorization of fish in relation to feeding (Karachle & Stergiou, 2010). Furthermore, it has been shown that for a given body length, gut length of herbivorous fish is larger than that of omnivores, and that of omnivores larger than that of carnivorous species (Karachle & Stergiou, 2010; Nikolski, 1963). The values estimate of intestinal index in T. hildebrandi was of 0.24-0.45; this Ii-values varied within the expected range (<1) for carnivores species (Karachle & Stergiou, 2010; Ward-Campbell et al., 2005). In this sense, T. hildebrandi diet in wild environments consists predominantly of insects.
Teleost fishes commonly have pyloric caeca (Sano, 2021), however they are absent in T. hildebrandi. The presence of the intestinal valve is a remarkable character of the intestine in T. hildebrandi, as is also observed in some flatfish species of Paralichthyidae (Gisbert et al., 2004; Kobelkowsky & Rojas-Ruiz, 2017) and of species of Sparidae (Cataldi et al., 1987). These fish are carnivores, which are also characterized by the presence of a very short intestine. Although there are substantial changes to the bony elements of the feeding apparatus within cyprinodontiforms (Hernández et al., 2009) changes in intestinal morphology, may be just as important in enhancing novel feeding modes. One of these structures is the intestinal valve; the intestinal valve appears as a constriction of the intestinal mucosa dividing the intestine in two regions, the prevalvular (anterior) and postvalvular (posterior) intestine (Gisbert et al., 2004). It is possible that the valve act then passively avoiding the reflux of the intestinal material, as well as directing the gut contents to the rectum (Nachi et al., 1998; Oliveira-Ribeiro & Fanta, 2000).
Stomach content analysis is widely used to determine food composition, feeding strategies, trophic position, energy flow (Hyslop, 1980). Food habit and trophic morphology studies of fishes are necessary to understand the role they play in the food web (Gerking, 1994; Luczkovich et al., 1995; Pease et al., 2020). We found that T. hildebrandi fed mainly on insects (66.6 % IRI), especially Chrironomus larvae (> 50 % IRI). The feeding behavior of T. hildebrandi is scarcely documented; Velázquez-Velázquez et al. (2007) described aspects related to feeding, reproduction and growth of the Chiapas killifish; they concluded that it is an insectivorous fish, with a specialized diet, based mainly on insect larvae.
The descriptive and comparative morphology has played an important role in the reconstruction of the evolutionary history and classification of cyprinodontiform fishes, often providing useful phylogenetic information at different taxonomic levels (Costa, 2006; Dominguez-Cisneros et al., 2023; Ghedotti & Davis, 2013; González-Díaz et al., 2014). In addition, morphological characterization provides a good approximation of feeding modes or types of prey that are used differentially by species (Ornelas-García et al., 2018). The descriptive nature of our results provides an effective method to analyze the relationship between diet and morphology of cyprinodontiform fishes. This study showed a significant correlation between ecomorphological traits and trophic habits (diet composition); this information may be useful for ecological niche studies (Calixto-Rojas et al., 2021), among members of the family Profundulidae and other groups within the Cyprinodontoidei suborder.
Our study provided clear evidence that diet-morphology specialization occurs in this fish species studied. The food content in the intestine, mainly of insects, the robustness of the jaws, the low number of gill rakers and the shortness of the intestine, are aspects that allow us to conclude that T. hildebrandi is carnivore fish of entomophagous type.
Ethical statement: the authors declare that they all agree with this publication and made significant contributions; that there is no conflict of interest of any kind; and that we followed all pertinent ethical and legal procedures and requirements. All financial sources are fully and clearly stated in the acknowledgments section. A signed document has been filed in the journal archives.