Introduction
Orchidaceae represents one of the most diverse families of flowering plants, consisting of about 35 000 species, which have fascinated botanists and plant enthusiasts over centuries (Barthlott et al., 2014), due to its extensive horticultural, medicinal, and culinary uses. The Cattleya genus is one of the most popular and widely cultivated in this family; the high ornamental value of its members and large ability for genetic recombination are attractive to the market (Galdiano et al., 2017). Cattleya crispa Lindl. is an ornamental epiphyte, endemic to the Brazilian Atlantic Forest (Van Den Berg, 2020); it grows slowly, and its generation time is estimated at about ten years (CNCFlora, 2022). Due to predatory extractivism and human-induced habitat loss this species appears on the Red List of Brazilian Flora (CNCFlora, 2022). As a species threatened with extinction, C. crispa is protected internationally under the appendix II of the Convention on International Trade in Endangered Species of Wild Fauna and Flora (UNEP-WCMC, 2022).
Programs for ex situ conservation of wild plant germplasm are fundamental to preserving the world's declining biodiversity (Merritt et al., 2014). To achieve this goal, in vitro culture techniques provide important tools, however it is necessary to understand the biology of the seeds, as well as the structure and function of the protocorm, to optimize methodologies for the of orchid seedlings (Yeung, 2017). Accordingly, morphoanatomical research on seeds and on post-seminal development of Cattleya plants in vitro has been carried out (Bazzicalupo et al., 2021; Gallo et al., 2016; Hosomi et al., 2012; Salazar-Mercado & Vega-Contreras, 2017). This type of studies contributes to the understanding of physiological processes, to the interpretation of germination tests and to the development of efficient propagation techniques and conservation programs (Corredor-Prado et al., 2014; Gallo et al., 2016).
For long-term conservation, cryopreservation is a recommended technique for plant with non-orthodox seeds, vegetatively propagated plants, and rare and endangered species (Engelmann, 2011). Adequate protocols can provide high plant regrowth after thawing, thus facilitating the establishment of cryobanks of plant genetic resources, in an organized and strategic manner (Benelli, 2021). The use of seeds allows the maintenance of a wider genetic basis and is appropriate for endangered species, since the parent plant does not have to be destroyed to obtain the seeds (Kulus & Zalewska, 2014). In orchids, the cryopreservation of seeds of some species has been successful (Kaur, 2019). However, complexities in the behavior of low-temperature storage still require explanation and resolution (Merritt et al.,2014).
Considering that the advancement of knowledge on reproductive structures and useful for conservation programs (Gallo et al., 2016), this study aimed to characterize morpho-anatomical aspects regarding germination and post-seminal development from C. crispa seeds; as well as studying the effect of cryopreservation on these seeds. We hypothesized that the immersion of C. crispa seeds in liquid nitrogen does not cause a negative effect on plant development processes.
Material and methods
Seed material: Seeds of C. crispa come from the ex situ conservation collection of the Orquidário Frederico Carlos Hoehne - Institute of Botany, located in Água Funda, São Paulo-Brazil. Seven months after cross-pollination, six mature capsules were collected from three different individuals. All capsules were in pre-dehiscence stage. Seed moisture content (MC) was determined by the low-constant temperature oven method (ISTA, 1985), at 103 ± 2 ºC for 17 h. Three seeds replicate of 10 mg were used, and the moisture content was expressed as a percentage:
W1 = weight of aluminum boat, W2 = weight of aluminum boat + seeds before drying, W3 = weight of aluminum boat + seeds after drying.
In vitro culture: The seeds were soaked in sterile-distilled water with a drop of surfactant detergent (Tween™ 20) during 10 min., in constant agitation. Then, they were sterilized with 0.5 % sodium hypochlorite (NaClO) for 10 minutes and rinsed three times with sterile distilled water. Solution changes were made using a sterilized Pasteur pipette. The seeds were sown on Murashige & Skoog (1962) medium supplemented with 30 g/l sucrose (P.A. Sigma™), solidified with 2 g/l gelling agent (Phytagel: Sigma™), and set into pH 5.5 before being sterilized at 120 ºC for 15 minutes. About 1 000 seeds were cultured in polystyrene Petri dishes (150 mm × 15 mm) with 20 ml of culture medium (four replicates were made). The cultures were maintained in growth room at 25 ± 2 ºC and photoperiod of 16 h with luminous intensity 50-60 µmol m−2 s−1 by clear fluorescent light.
Morpho-anatomical description: The material was analyzed under stereomicroscope, light microscope, and electron microscopy. The characters analyzed were coloration, shape, length, and the presence of polysaccharides. For biometric description 100 ripe seeds were randomly selected and had their length recorded by Image J version 1.8.0 software (National Institutes of Health, Bethesda, Maryland, USA). We consider mature seeds those developed 7 months after the cross, before being inoculated in vitro. Then, seeds inoculated in vitro as indicated above, were examined weekly under a stereoscopic microscope (SZH 10: Olympus™), to evaluate developmental stages from seed to seedling formation. We classify these stages according to an adaptation of Arditti (1967) (Table 1). Collections for microscopic analyses were performed 0, 7, 15, 30 and 60 days after sowing (DAS).
Table 1 Stages initial development in vitro of Cattleya crispa seeds adapted from Arditti (1967)
Stage | Description |
0 | Unviable seeds |
1 | Swollen and green embryo with ruptured testa (= germination) |
2 | Early globular protocorm (bodies formed by the continued embryo enlargement after germination= protocorm) |
3 | Protocorm showing a pointed vegetative apex and rhizoids |
4 | Protocorm with one emerged leaf |
5 | Protocorm with two spreading leaves |
6 | Seedling showing two or more leaves with root presence (= seedling) |
Light microscopy (LM). The fresh samples were removed from the culture medium and dabbed dry on filter paper. The material was fixed in 2.5 % glutaraldehyde and 0.1M phosphate-buffered saline (PBS) (1:1, v/v) followed by dehydration in series of ethanol aqueous solutions. The samples were infiltrated with hydroxyethyl-methacrylate (Historesin: Leica™), according to the manufacturer's instructions. For sectioning (5 µm), we used a manual microtome (Slee Cut 4055: Mainz™). The histochemical tests performed were periodic acid-Schiff reagent (PAS) for polysaccharides (Feder & O'Brien, 1968), and toluidine blue O (TB-O) for metachromasy (O'Brien et al., 1964). The images were obtained using a light microscope (BX40: Olympus™) with a high-resolution color digital camera (DP71: Olympus) and Capture Pro 5.1 Image Software.
Scanning electron microscopy (SEM). After fixation and dehydration as described above, the samples were placed on strips of carbon tape, and affixed on the sample stub to continue dehydration, by the low surface tension solvent 1,1,1,3,3,3-hexamethyldisilazane (HMDS). The dried samples were covered with 20 nm of gold in metallizer (EM SCD 500: Leica™), to be studied under a scanning electron microscope (JSM-6390LV: Jeol™).
Transmission electron microscopy (TEM). The material was fixed with 2.5 % glutaraldehyde, 0.1 M sodium cacodylate buffer (pH 7.2) and 0.2 M sucrose. The samples were then post-fixed in 1 % osmium tetroxide for 6 h, dehydrated in graded acetone series and embedded in Spurr's resin (Leica™). After sectioning, the material was stained with aqueous uranyl acetate followed by lead citrate. The sections were examined under a transmission electron microscope (JEM-1011: Jeol™).
Cryopreservation: Two treatments were designed: cryopreserved seeds in liquid nitrogen, referred to as +LN, and non-cryopreserved seeds in liquid nitrogen, referred to as -LN. The cryopreservation of the seeds (+LN) was carried out by ultra-rapid freezing by direct immersion in liquid nitrogen (-196 ºC). Four 1.5 ml plastic cryotubes containing 20 mg of seeds were used. After 48 h, the cryotubes were thawed in a water bath at 40 ºC for 2 min. Then, the seeds were sterilized and cultured as previously described. Non-cryopreserved seeds (-LN) were sterilized and cultured immediately. Four replicates per treatment were considered. The protocorms formed from seeds (+LN and -LN) were sub-cultured on the same medium type.
A subset of seeds from both treatments was used to assess viability using the 2,3,5-triphenyltetrazolium chloride (TTC) test. The seeds were soaked in distilled water for 17 h at 25 ± 2 °C. The water was removed, and the material was soaked in 0.5 % TTC solution in total darkness for 15 h. After staining, viability (%) was determined by counting the number of seeds that showed red coloration (viable). A lack of red coloration and/or pale pink colors would indicate the death of the embryo (Salazar-Mercado et al., 2020), and therefore the non-viable seed (Fig. 1). Four replications of 300 seeds were analyzed using a microscope (BX40: Olympus™).
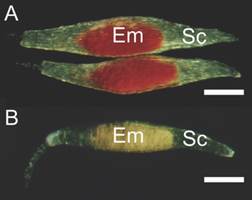
Fig. 1 Cattleya crispa seeds submitted to the tetrazolium viability test. A. Viable embryo has red color. B. Unviable embryo, non-colored. Em embryo; Sc seed coat. Bar: 200 µm.
Seed germination (%), survival rate (%) and protocorms weight (mg) were evaluated 5 months after sowing. Swollen and green embryo with ruptured testa was the criteria used to define germination. The germination was calculated by dividing the germinated seeds per total amount of seeds inoculated in each Petri dish. The survival rate was evaluated by counting as live the green protocorms and seedlings among the germinated ones. Lastly, weight of the protocorms (mg) was obtained from 30 protocorms of each Petri dish. To identify if immersion in liquid nitrogen influences the development of C. crispa, data were subjected to analysis of variance (t-test, P < 0.05) using Statistica® software (Statsoft Inc., Tulsa, OK, USA).
Results
Moisture content and seed morphology: Cattleya crispa seeds presented 5 % water content. They presented whitish-yellow coloration, fusiform shape and length varying in the range of 700-900 µm (excluding the suspensor). The seeds were relatively undifferentiated and characterized by the presence of one ellipsoidal shaped embryo at the center and a slightly pigmented seed coat without any visible endosperm or cotyledon (Fig. 2A). The chalaza extremity was tapered and closed, and the basal region had an overture at the micropylar end, and in some seeds, the suspensor projected through the micropyle (Fig. 2B, Fig. 2C, Fig. 2D). The suspensor is multicellular and consists of 2-3 cell layers. The seed coat cells were elongated, rounded at the end and without intercellular gaps. The orientation of the testa cells was parallel to each other.
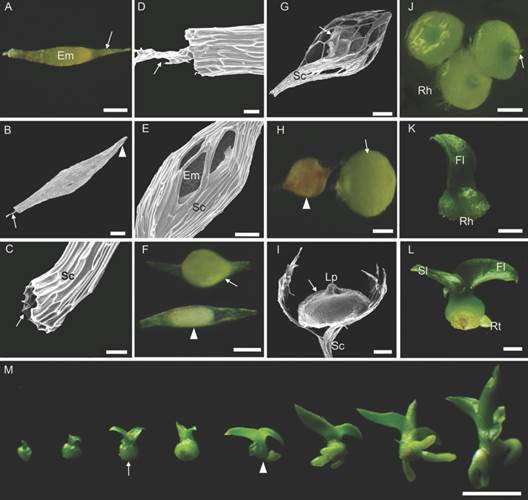
Fig. 2 Seed, germination, and post-seminal development in vitro of Cattleya crispa. A.-D. 0 DAS: General aspect of external seed morphology. A. Slightly pigmented seed coat (arrow) with the embryo inside. B. Arrow suspensor projected through the micropylar end; arrowhead: closed chalaza extremity. C.-D. Close view of the micropylar end. C. Arrow: opening in the seed coat. D. Arrow: suspensor. E.-F. 7 DAS. E. Seed germination. Ruptured seed coat. F. Arrowhead: unviable seed (Stage 0); arrow: swollen and germinated embryo (Stage 1). G. 15 DAS: Continuous growth of the chlorophyll structure. H. 30 DAS: arrowhead: protocorm with signs of oxidation; arrow: globular-shaped protocorm (Stage 2). I.-J. 60 DAS: I. Exposed protocorm (arrow), with broken seed coat. J. Protocorms with leaf primordia (arrow) and rhizoids (Stage 3). K. 80 DAS: Protocorm with the first leaf emerged (Stage 4). L. 110 DAS: Seedling with two leaves and the presence of root (Stage 6). M. Gathering of the development process, from the protocorm with leaf primordia until complete seedling stage. Arrow: protocorm with two spreading leaves and absence of root (Stage 5). Arrowhead: marks the end of the protocorm stage (Stage 6) with complete seedlings forward. Em embryo; Rh rhizoids; Fl first leaf; Sl second leaf; Rt root; Sc seed coat; Lp leaf primordia. Bar: 50 µm (C., D., E.); 100 µm (A., B., F., G., H., I.); 500 µm (J.); 1 mm (K.-M.).
Morphoanatomy of post-seminal development: Seeds at the 5 DAS on the culture medium presented the swollen embryo due to the imbibition process, however, it was not possible to differentiate viable from non-viable seeds. The germination process started 7 DAS (stage 1), when the embryo was swollen and green, visibly different from unviable seeds, which showed white color and no alterations in the embryo/seed coat ratio (stage 0) (Fig. 2E, Fig. 2F). The seeds presented continuous growth of the chlorophyll structure, filling and stretching the central zone of the ruptured seed coat (15 DAS) (Fig. 2G). By the 30 DAS, the globular protocorm is identified (stage 2). An early oxidation with reddish-brown color appearance was also visible in some protocorms (Fig. 2H). The vegetative organs started to develop at 60 DAS (stage 3). The upper part of the protocorms showed a vegetative apex containing the leaf primordium in formation, whereas at the base the rhizoids were observed (Fig. 2I, Fig. 2J). At 70-80 DAS, the first leaf emerged in some protocorms (stage 4) (Fig. 2K).
Although the germination process showed to be morphologically homogeneous, protocorms were observed at different development stages over time. With 100-110 DAS, the protocorms showed a well-spread second leaf (stage 5), while the first leaf gradually became wider and thicker. The roots were observed at 150 DAS (stage 6); we define this morphological change as the ending mark of the protocorm stage and the beginning of the seedling phase (Fig. 2L, Fig. 2M).
The embryo was formed by a protoderm that delimited the promeristem (0 DAS) (Fig. 3A). The longitudinal sections showed an embryo with elliptic shape and the discernible chalazal-micropylar axis. The chalaza end was closed and composed of smaller and denser cells, while the micropylar region contained larger cells, with a suspensor projecting from the open micropylar end (Fig. 3B). At the beginning of germination (7 DAS), the swelling embryo became more globous due to imbibition and increased cell number. Changes in shape and volume were accompanied by histological differentiation, evidencing the embryo bipolarity (Fig. 3C). Within 15 DAS, the protocorm showed lateral expansion. The shoot apex showed intense meristematic activity, with anticlinal divisions of the protoderm cells and divisions in several planes in the parenchyma cells, while the suspensor and the basal region cells began to disappear, and the seed coat collapsed and was retained in the medium (Fig. 3D). At 30 DAS, the protoderm became epidermis, presenting rhizomes and trichomes. Continuous cell divisions gave the protocorm a flat shape, with parenchymal cells with large vacuoles (Fig. 3E). At 60 DAS, some protocorms began differentiation at the shoot apex (Fig. 3F), while in others they already showed the leaf primordium (Fig. 3G). On the other hand, the brown protocorm samples showed widespread cell death (Fig. 3H).
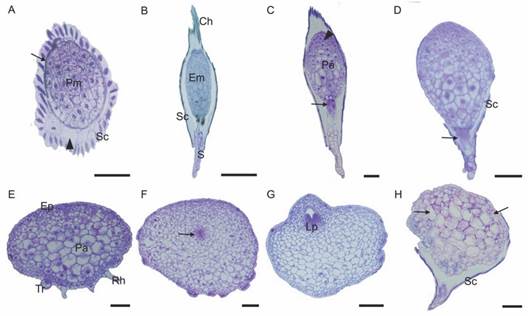
Fig. 3 Histological aspects of Cattleya crispa during germination and post-seminal development in vitro. A. 0 DAS: Cross-section. Embryo composed of protoderm (arrow) and promeristem. Seed coat encloses the air space indicated by the arrowhead. B. 0 DAS: Longitudinal section. The suspensor is projected through the open micropylar end. C. 7 DAS: Germination. The shoot apex cells in divisions (arrowhead) and the hypophysis cells (arrow). D. 15 DAS: Lateral expansion of the protocorm. Degradation of the suspensor and the basal region cells (arrow). E. 30 DAS: Protocorm with large lateral expansion. Parenchyma with large vacuoles. Trichomes and rhizoids in the basal region. F.-H. 60 DAS. F. Differentiation of the shoot apex cells (arrow). G. Visible leaf primordia. H. Brown protocorm showing cell death. Arrows indicate altered cells. Pm promeristem; Sc seed coat; Em embryo; S suspensor; Ch chalaza; Pa parenchyma; Ep epidermis; Rh rhizoids; Tr trichome; Lp leaf primordia. Bar: 50 µm (A); 200 µm (B); 100 µm (C-H).
The cells of the embryo (0 DAS) were rich in lipid and protein bodies allotted in globoids structures of varying sizes, which, accompanied by limited early starch granules, are the primary reserves of C. crispa seeds (Fig. 4A, Fig. 4B). During the imbibition process (7 DAS), some events were observed: mitotic activity in the epidermal cells; rapid deposition of polysaccharides all over the embryo (Fig. 4C); and digestion of protein bodies, which are probably a result of the activation of protease metabolism. In addition, the starch grains got bigger (Fig. 4D). The polysaccharide granules were arranged around the nucleus of the cells after 15 DAS (Fig. 4E). Due to the rapid digestion of the lipids, glyoxysomes became visible and likely to be found in proximity to the lipid bodies. Large amyloplasts containing starch grains were evident (Fig. 4F). Parenchyma cells became largely vacuolated by the 30 DAS, and a gradient of polysaccharide density is visible from the basal region of the protocorm to the upper region (Fig. 4G, Fig. 4H). Polysaccharides at 60 DAS were largely mobilized to the cells of the shoot apex in differentiation and to the cell layers close to the epidermis (Fig. 4I). These cells have dense cytoplasm, which evidence the formation and coalescence of lipidic bodies (Fig. 4J).
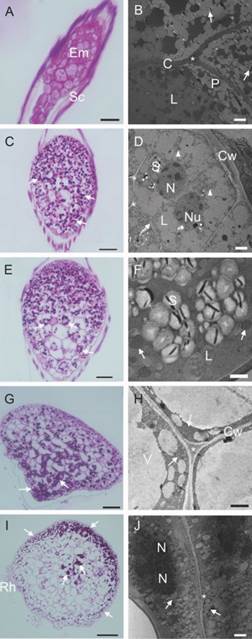
Fig. 4 Reserve compounds during germination and post-seminal development in vitro of Cattleya crispa seeds. A.-B. 0 DAS. A. starch granules did not appear with PAS staining. B. Embryo rich in lipid and protein bodies. Arrows indicate a few early starch granules that did not appear with PAS staining. C.-D. 7 DAS. C. Germination. Deposition of polysaccharides (arrow). D. Arrow: cell walls were still thin; arrowheads largely digested protein bodies. E.-F. 15 DAS. E. The polysaccharides tend to congregate around the nucleus (arrow). F. Presence of glyoxysomes, found in proximity to the lipid bodies in process of digestion (arrow). G.-H. 30 DAS. G. Increased polysaccharides in the basal region of the protocorm (arrow). H. Parenchyma cells largely vacuolated with disorganized protein (arrow). I.-J. 60 DAS. I. Polysaccharides mobilized to the cells of the shoot apex in differentiation and to the cell layers close to the epidermis (arrow). J. Cells with extensive lipidic bodies formation (arrow). Em embryo; Sc seed coat; Cw Cell wall; L lipid bodie; P protein bodies; S starch grain; N nucleus; Nu nucleolus; Rh rhizoids; * Middle lamella. Bar: 50 µm (A., E.). 100 µm (C., G., I.). 2 µm (B., D., J.). 1 µm (F., H.).
We observed that most of the protocorms collapsed around 60 DAS. The living protocorms had completely lost the seed coat, whereas in the brown protocorms the seed coat was still attached (Fig. 5). Ultrastructural alterations in the parenchyma of dying protocorms confirmed a generalized cell death (Fig. 5D, Fig. 5H).
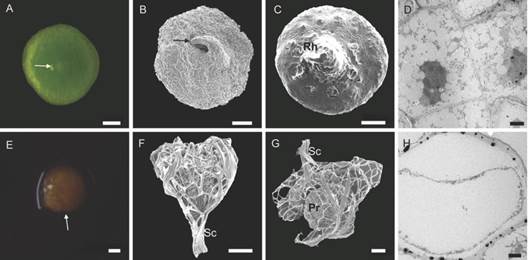
Fig. 5 Protocorms of Cattleya crispa collected at 60 DAS. A.-D. Green and alive protocorms without the seed coat. A.-B. Vegetative apex with the leaf primordium in formation (arrows). C. rhizoid formation. D. TEM image shows intact parenchyma. E.-H. Dying protocorms. E. Protocorm with brown color (arrow). F.-G. protocorm with the seed coat attached. H. TEM image shows cell death. Rh rhizoids; Sc seed coat; Pr protocorm. Bar: 2 µm (D., H.). 100 µm (A., B., C., E., F., G.). 200 µm (A.). 500 µm (E.).
Seed cryopreservation: viability (%), germination (%), survival rate (%) and protocorms weight (mg) did not show significant differences between +LN and -LN (Table 2). Despite high viability and germination rates, most of the protocorms collapsed (reddish-brown protocorms), which led to a low survival rate in both treatments (Table 2).
Table 2 Effect of cryopreservation of Cattleya crispa seeds by direct immersion in liquid nitrogen.
Variable | non-cryopreserved seeds (-LN) | cryopreserved seeds (+LN) |
Seed viability (TCC) (%) | 87.15 ± 1.99 ns | 88.04 ± 2.20 ns |
Seed germination (%) | 78.32 ± 3.37 ns | 81.14 ± 2.33 ns |
Survival rate (%) | 8.48 ± 3.61 ns | 11.12 ± 3.92 ns |
Protocorms weight (mg) | 104.27 ± 1.90 ns | 104.85 ± 2.82 ns |
Data are the mean value ± standard error. The letters ns indicate not significant differences (ANOVA, P < 0.05) between +LN and -LN.
Discussion
Seed morphology: Considering the five seed size categories established by Barthlott et al. (2014) (Very small 100-200 µm; small 200-500 µm; medium 500-900 µm; large 900-2 000 µm; and very large 2 000-6 000 µm), our data indicate that C. crispa has medium-sized seeds. This category includes the seeds most found in the Orchidaceae family (Barthlott et al., 2014). On the other hand, the shape (fusiform) and pigmentation (whitish-yellow) in C. crispa seeds have also been reported in other epiphytic orchids, including the Cattleya genus (Diantina et al., 2020). Ramudu et al. (2020) indicate that fusiform seeds appear to be the basic form from which all other seed shapes evolved in this family.
Some studies suggest a probable relationship between the presence of twisted testa cells along the longitudinal axis with the epiphytic habitat (Barthlott et al., 2014; Gamarra et al., 2018). However, C. crispa presented testa cells with parallel orientation, which is a feature shared with terrestrial taxa (Gamarra et al., 2018). Epiphytic orchids of the genera Ansellia, Graphorkis and Liparis also presented this type of orientation (Gamarra et al., 2018). Regarding the long multicellular suspensor found in C. crispa, previous studies have also reported it in other Cattleya species and other members of the Laeliinae subtribe (Bazzicalupo et al., 2021; Gallo et al., 2016). Bazzicalupo et al. (2021), indicate that the suspensor dimension does not influence seed development.
Morphoanatomy of post-seminal development: The categorization of development stages allows the measuring of the seedling's development and compares the effectiveness of different germination procedures (Hoang et al., 2016). Even though some authors consider the loss of the testa with posterior formation of a tuberiform structure called protocorm as the characterizing event of orchid germination (Arditti, 1967), we do not consider this as the main factor for C. crispa germination. We define germination as Salazar-Mercado & Vega-Contreras (2017) did for Cattleya trianae. In the germination of C. crispa the testa was broken, and the embryo was visible as a small green propagate. This event occurred seven days after sowing. However, in some species of orchids the onset of germination occurs two months after seed sowing (Dolce et al., 2020). Subsequently, the C. crispa seeds form the protocorms, which are observed in different stages of development throughout the evaluated time. According to Nikishina et al. (2007), orchid seeds are quite heterogeneous, and, among sown seeds, there is usually a population of strong seeds, which largely overcomes the others in their development. The initial growth of C. crispa was similar to that reported for other orchids (Abrahám et al., 2012; Hossain et al., 2010).
Regarding the reserve compounds in orchid seeds, Yeung (2017) indicate that the embryos have cells full of storage products: abundant proteins and lipids, while rarely starch granules. These compounds were found in C. crispa and have been reported in other species of the genus (Schvambach et al., 2022) as well as in other groups of plants (Corredor-Prado et al., 2014; Martelo-Solorzano et al., 2022). In C. crispa, the evident presence of polysaccharides during post-seminal development, including starch, is attributed to the mobilization of lipids and imbibition of the sucrose from the culture medium. Penfield et al. (2005) reported that this mobilization leads to the formation of acetyl Co-A with the subsequent synthesis of sucrose through gluconeogenesis. If the levels of polysaccharides in the cytosol are too high, they can be stored as transient starch. We believe that there is a correlation between the accumulation of starch grains during the germination and the degradation of primary reserves. The mobilization was probably greater than the required for these initial stages. Therefore, to maintain the osmotic balance, the excess was converted into starch reserve.
Seeds of Cattleya do not have an inner integument that provides physical dormancy, which is commonly present in temperate orchids (Custódio et al., 2016). Likewise, in C. crispa, we did not identify integumentary dormancy once the seed coat allows water penetration and subsequent germination. However, we observed that in some seeds the cover is persistent, affecting the development of the protocorm which initiates an oxidation process that ends with cell death. These results are in accordance with previous findings for other orchids species. According to Dalzotto & Lallana (2015), the seed coat of Bipinnula pennicillata does not limit the asymbiotic germination of the seeds; instead, it causes a delay in obtaining protocorms with apical bud and rhizoids. Other study with ten species of Cattleya showed that, by the 63 DAS, the protocorms' quality declined and some of them became shriveled and brown (Hosomi et al., 2012). Therefore, we suggest procedures to increase the survival rate of protocorms, by softening or breaking down the seed coat. For this, Zeng et al. (2014) indicate the use of cold treatments, prolonged imbibition, chemical scarification of the testa with sodium or calcium hypochlorite, or mechanical damage. In this way, it is necessary to expand the research focused on C. crispa seed pretreatments, which could lead to better results. As Arditti (1967) suggests, orchid seeds, despite their diminutive and fragile appearance, can resist and survive relatively harsh treatments.
Seed cryopreservation: In general, the germinability of cryopreserved seeds is high among tropical orchid species (70-100 %) (Popova et al., 2016). It should be noted that the water content of the seeds is an important factor for their successful cryopreservation, since low water levels in embryo cells reduce lethal intracellular freezing injuries (Hirano et al., 2005). Therefore, the high viability and germination of C. crispa in this study was related to the initial optimal value of water content of seeds (5 %), which indicates their orthodox nature and allows a simple cryopreservation technique. This confirms that orchid seeds with a moisture content below 13 % can be successfully cryopreserved by direct immersion in liquid nitrogen (Popova et al., 2016). However, for other orchid species, is necessary to use cryoprotectant solutions (Galdiano et al., 2017; Merritt et al., 2014; Vettorazzi et al., 2019). Several studies have successfully cryopreserved different orchid species finding that germination rates after the liquid nitrogen storage remained equal or higher (Nikishina et al., 2007; Popova et al., 2016). Similarly, our results demonstrated that seed viability, germination, survival rate, and the weight of the formed protocorms were not significantly altered by direct immersion of seeds in liquid nitrogen. Therefore, the establishment of cryobanks presents great potential for long-term storage of C. crispa seeds. According to Silva et al. (2021), the development of methods for secure storage of seeds contributes to the preservation of genetic diversity, which is crucial in the case of species that are vulnerable or endangered.
On the other hand, the tetrazolium test in seeds showed to be effective to evaluate the physiological quality in C. crispa similar to other studies with orchids, the results of the tetrazolium test were consistent with the seed germination data (Galdiano et al., 2017; Hosomi et al., 2012; Vettorazzi et al., 2019). This test has been successfully used to evaluate cryopreserved seeds of other orchids (Vettorazzi et al., 2019).
To our knowledge, this is the first study that has developed an abiotic culture of C. crispa using biotechnological tools, as well as the first morpho-anatomical and ultrastructural report during the germination, post-seminal development, and cryopreservation of this species. Through the monitoring of the evaluated development stages, we identified that the seed coat is sometimes persistent, affecting the development of the protocorm. Our hypothesis was accepted, since we found that the viability and germination of the seeds, as well as the formation of the protocorms, did not vary significantly after the immersion of the seeds in liquid nitrogen. The cryocapacity of these seeds is evidence that cryopreservation can be a strategy for the long-term conservation of C. crispa. We recommend the assessment of different seed pretreatments, in order to optimize the obtention of viable protocorms. The results of this work contribute to the overall biology of C. crispa and to the propagation and storage of genetic material for conservation purposes.
Ethical statement: the authors declare that they all agree with this publication and made significant contributions; that there is no conflict of interest of any kind; and that we followed all pertinent ethical and legal procedures and requirements. All financial sources are fully and clearly stated in the acknowledgments section. A signed document has been filed in the journal archives.