Introduction
The fireworm Hermodice carunculata (Pallas, 1766) is a widespread polychaete found in tropical and temperate marine ecosystems (Righi et al., 2020; Wolf et al., 2014). It can prey on many benthic species, including corals, octocorals, and hydrozoans (Vreeland & Lasker, 1989; Witman, 1988). In the Caribbean, H. carunculata can prey heavily on the reef-forming coral Acropora cervicornis (Lamarck, 1816) (Fig. 1, Knowlton et al., 1990; Miller et al., 2014). The fireworm does not necessarily consume the entire colony; however, it can adversely affect coral demography (Miller et al., 2014). For instance, the survival and growth of A. cervicornis can be compromised if tissue consumption exceeds 20 % of the total colony size (see Mercado-Molina et al., 2018). Moreover, predation can result in population decline if large colonies retrogress into smaller sizes (Mercado-Molina, Ruiz-Diaz, Pérez, et al., 2015). In fact, in Jamaica, H. carunculata was linked to the extinction of an A. cervicornis subpopulation (Knowlton et al., 1990).
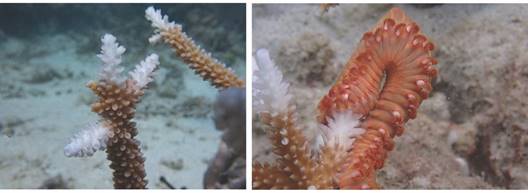
Fig. 1 Left: The white tips of an A. cervicornis colony show signs of recent tissue consumption by Hermodice carunculata. Right: Hermodice carunculata engulfing a colony outplant of the threatened coral Acropora cervicornis at Punta Melones reef in Culebra, Puerto Rico.
Although H. carunculata corallivory may negatively affect A. cervicornis, its feeding dynamic is poorly understood. Furthermore, very few studies have attempted to understand the effects of predation at the population level (Goergen et al., 2019; Wolf et al., 2014; Miller et al., 2014). The coral A. cervicornis is a threatened species that plays an essential role in maintaining the ecological functions and services of coral reefs. It contributes to reef accretion (Gilmore & Hall 1976; Tunnicliffe, 1981), promotes biodiversity (Agudo-Adriani et al., 2016), and shapes biological interactions (Weil et al., 2020). Due to its ecological importance, many restoration programs have been established across the region (Bayraktarov et al., 2020; Hernández-Delgado et al., 2014). However, the lack of information about the predatory behavior of fireworms limits practitioners'ability to develop restoration plans that can counteract the aggressive behavior of the fireworms. By understanding the predation dynamics of H. carunculata, coral outplanting can, for instance, be timed and located based on where and when the species is more active. Such information can be used to predict future trends of restored populations under different predation scenarios.
This study aims to describe the predation dynamics of H. carunculata on a restored population of the threatened coral A. cervicornis in Puerto Rico. The following questions were answered: 1) Do predation rates vary in space and time? 2) Are introduced colonies more susceptible to predation than local colonies? 3) Is there a relationship between colony complexity and predation susceptibility? 4) Does predation by H. carunculata put the viability of the restored population at risk? The answer to these questions will contribute to a better understanding of the processes that can hinder the success of coral reef restoration and help improve recovery plans for A. cervicornis.
Materials and methods
Study site: The study was conducted in the Island Municipality of Culebra, approximately 30 km east of the mainland of Puerto Rico (Fig. 2). In 2014, the USA National Oceanographic and Atmospheric Administration designed Culebra as part of the Northeastern Reserves System Habitat Focus Area due to its representative biodiversity of Caribbean marine ecosystems and its socio-economic value. The study was carried out specifically at Punta Melones reef (PMEL), where Sociedad Ambiente Marino (SAM) is mitigating the effects of reef degradation by increasing the density of A. cervicornis. PMEL, located on the western coast of Culebra, has a maximum depth of five meters and is dominated by macroalgae assemblages (e.g., algae turfs and Ramicrusta sp.). The macroalgal community also includes Dictyota spp., but their abundance varies considerably throughout the year (unpublished data). The sea fan Gorgonia ventalina (Linnaeus, 1758) is the predominant octocoral species, being particularly abundant in the shallower zones of the reef (e.g., reef crest). Approximately 5 % of the reef is covered by corals, mostly Porites astreoides (Lamarck, 1816) and Porites porites (Pallas, 1766). Before the start of the restoration program, A. cervicornis was absent from the study site. Information about the abundance and distribution of H. carunculata in PMEL is lacking. However, we have observed the fireworms across the whole reef, including reef flats, sandy areas, and areas of high topographic relief.
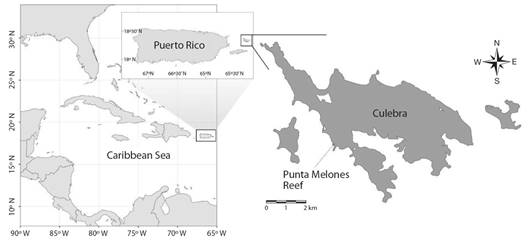
Fig. 2 The study was conducted at Punta Melones reef, located in the Island Municipality of Culebra, Puerto Rico.
Restored populations: At the beginning of the study in December 2020, three 60 m2 (30 m x 2 m; Fig. 3) permanent belt transects, separated by 5 m each, were established parallel to the coast. In each transect, 150 fragments of A. cervicornis (median colony size = 17.40 cm) were fixed to the reef substrate using concrete nails and plastic cable ties. Coral fragments were harvested from in-situ coral nurseries operated by SAM. The physical structure of the reef framework differed between the transects. Transect A, the southernmost, was a reef flat dominated by small dead coral boulders and flat consolidated substrate, being the less complex (Rugosity Index = 1.17) of the three transects established. Transect B was laid across a moderately complex reef slope (Rugosity Index = 1.19) with a consolidated bottom. Transect C, the northernmost, was characterized by pinnacles and ridges up to 3 m in height, visually dominated by holes and crevices. In this sense, Transect C was the most complex (Rugosity Index = 1.24) of the three reef areas studied. Topographic relief was estimated using the chain method to calculate its rugosity index (Mercado-Molina, Montañez-Acuña, et al., 2015; Nemeth & Appeldoorn, 2009). A 10 m long chain was superimposed along the contour of the reef substrate, and the rugosity index was calculated as the ratio between the length of the chain and the distance it covered along the contour of the reef substrate (Nemeth & Appeldoorn, 2009).
Prevalence and incidence of predation: 33 colonies were tagged within each of the transects (total = 99) to monitor the prevalence and incidence of fireworm predation events. Tagged colonies were visited seven times between December 2020 and August 2022. Prevalence was defined as the percentage of colonies that showed signs of predation during a given time, whereas incidence was the number of new predation cases detected during the same period. Log-linear analysis was performed to determine whether the incidence and prevalence of fireworms predation were independent of time and location. Fate (F) was set as the response variable (e.g., predated vs. non-predated), whereas time (T) and location (L) were considered the explanatory factors. Following Caswell (2001) and Fingleton (1984), 0.5 was added to each cell value within the contingency tables to avoid estimation problems for values equal to 0. Log-linear analysis was performed in R (R Core Team, 2017) using the library MASS (Venables & Ripley, 2013).
Effect of predation on coral demography: The growth and survival of the tagged coral colonies were tracked seven times between December 2022 and August 2022. A coral colony was considered dead if no apparent live tissue was evident. Following Mercado-Molina, Ruiz-Diaz & Sabat (2015), growth rates were estimated as the change in daily linear extension (final length − initial length / total number of days) and expressed as cm/day. To estimate colony size, in-situ photographs (scale-by-side) of each colony were taken from different perspectives to ensure that all branches were captured. The initial and final sizes of the colonies were then calculated by adding up the linear lengths of the branches, excluding partial mortality when appropriate. Coral Point Count with Excel extensions (CPCe; Kohler & Gill, 2006) software was used for processing the photographs. Survivorship patterns and coral growth were compared between predated vs. non-predated colonies using the Kaplan-Meier Survival analysis and Mann-Whitney-U test, respectively. Survival analysis was performed in R using the package simsurv (Brilleman et al., 2021).
Demographic modeling: The restored fragments (collected from in-situ coral nurseries) were classified into two size classes, small (overall size ≤ 25 cm) and large (overall size > 25 cm). A size-based matrix population model was developed to estimate the growth rate of the restored population under two scenarios: 1. considering only predated colonies and 2. considering all colonies. The demographic model followed Equation 1:
(Equation 1)
Where the number of small-sized colonies (s) and large-sized colonies (l) at time t + 1 (one year into the future) equals the current number of colonies in each of the two size classes multiplied by a two-by-two matrix of the transition probabilities among size classes. The diagonal elements in the matrix represent the probabilities of colonies surviving and remaining in their current size class (stasis: Sss; Sll). The Rsl element of the matrix represents the contribution of large-sized colonies to the small-size class by size regression due to fireworm consumption of live tissue. Gls represents the probability of a colony transitioning from the smallest to the largest size class. The two size classes were chosen to maintain a sample greater than 25 colonies for each size category.
Two transition matrices were created, one accounted for only coral colonies that suffered H. carunculata predation, and the second included both predated and non-predated colonies. The dominant eigenvalue was calculated for each matrix to obtain the finite population rate of increase (λ). A life table response experiment (LTRE) analysis was performed to provide information on the magnitude of variation in a particular life cycle transition that contributed most to the observed difference in λ between treatments (e.g., fireworm corallivory). Elasticity was also used as an analysis to determine the transition rate that would contribute the most to λ values. Demographic analyses were performed in R (R Core Team, 2017) using the package popbio (Stubben & Milligan, 2007).
Colony susceptibility: The susceptibility of local and introduced populations to predation was compared. To accomplish this, we conducted a second outplanting event between January and February 2022 in which colony fragments were collected from the original restored population (e.g., >1-year-old, local population) as well as nurseries outside the study site (e.g., introduced population). Following the same methodology described above, colonies were outplanted along the permanent transects in the same numbers (e.g., 33 colonies per transect). Coral fragments were revisited in April 2022 and July 2022. The Chi-square test was used to determine whether the number of colonies predated was associated with their origin (local vs. introduced). Chi-square analysis was performed using R (R Core Team, 2017).
Results
Incidence and prevalence of colony predation: Results of the log-linear analysis indicate that predation rates of H. carunculata varied in space and time (Table 1). However, no significant interaction was found between the location (transect) and time (Table 1). Predation on coral outplants was first detected in February 2021 (Fig. 4). After the first sight, predatory attacks spiked considerably over time. April 2022 was the month with the highest percentage of new colonies predated. In August 2022, the incidence of predation decreased, probably because fewer colonies were at risk of being predated since most had already been predated. Prevalence of predation reflected the increasing incidence trends, suggesting that affected colonies could not regenerate the lost tissue (Fig. 5). By the end of the study, approximately 84 % of the colonies showed signs of predation. Corals outplanted in the northernmost location (Transect C) were more prone to predation than colonies outplanted in the southern part (Transect A and Transect B). In Transect C, the mean incidence rate per survey time was 42 % (range: 27-67 %), whereas, at Transect A and Transect B, the rate of new detections per survey time was approximately 21 % (range: 0-42 %). Of the 33 tagged colonies monitored in Transect C, only one was not predated (97 % predation) compared to seven and eight at Transect A (79 % predation) and Transect B (76 % predation), respectively. In general, predation within Transect C accounted for at least 44 % (range: 44-100 %) of new sights detected during survey times and ~ 40 % of the total colonies predated. Predated colonies also presented a moderate vulnerability for further predation events, with an average of 34 % probability of experiencing further attacks. Outplants along Transect C were more likely to be re-predated than outplants in the other two transects (Fig. 6). It was also found that fireworm predation events were significantly more prevalent in introduced colonies than in those collected from the local site (Chisq =8.58, P = 0.003, Fig. 7). The observed spatial pattern of predation could be a consequence of the spatial variation in abundance of the fireworms (Fig. 8).
Table 1 Results of the log-linear analysis comparing the effect of location and time on colony fate (e.g., predated vs. no predated) of the restored Acropora cervicornis population at Punta Melones reef, Culebra, Puerto Rico.
Effect | Models | Delta G | Delta df | P |
Location | TL, F vs. TL, FL | 7.35 | 2 | 0.025 |
Time | TL, F vs. TL, FT | 70.96 | 6 | < 0.0001 |
Location, given time | TL, LF vs. TL, FT, FL | 86.51 | 6 | < 0.0001 |
Time, given location | TL, TF vs. TL, FT, FL | 22.90 | 2 | < 0.0001 |
Location x Time x Fate | TL, FT, FL vs. TLF | 14.31 | 12 | 0.281 |
T= time; L = location (transect); F = fate (predated or not predated); df = Degree of freedom.
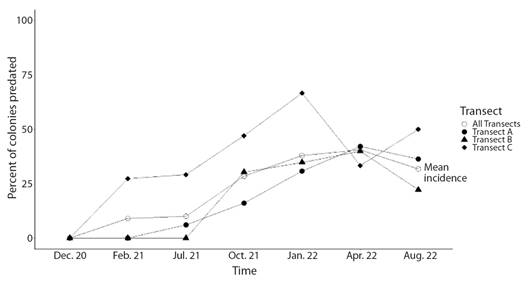
Fig. 4 Spatiotemporal pattern of predation incidence by the fireworm Hermodice carunculata on Acropora cervicornis outplants.
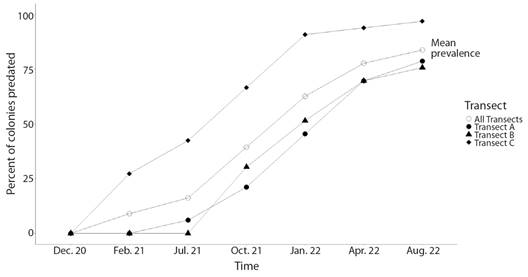
Fig. 5 Spatiotemporal pattern of predation prevalence by the fireworm Hermodice carunculata on Acropora cervicornis outplants.
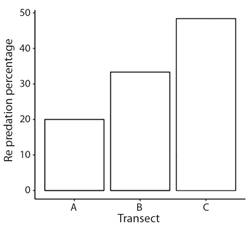
Fig. 6 Spatial comparison of the probability of a coral outplant to be re-predated by the fireworm Hermodice carunculta along the three transects established at Punta Melones reef, Culebra, Puerto Rico.
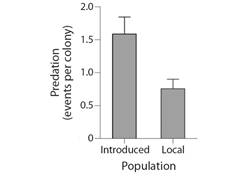
Fig. 7 Comparison of predation rates between colonies originating from Punta Melones (PMEL), referred as local, and colonies collected from outside nurseries (Introduced).
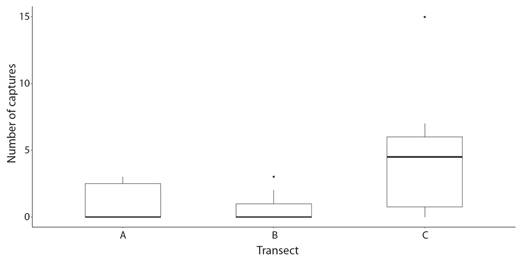
Fig. 8 Spatial variation in the abundance of the fireworm Hermodice carunculta in Punta Melones reef, Culebra, Puerto Rico.
Effect of fireworm corallivory on outplants demographic performance: Results of the Mann-Whitney-U-test indicate that predation significantly affected coral growth (W = -942, P < 0.0001). Before being attacked, coral outplants grew at 0.54 cm/day (± 1.03 SD; median = 0.47), decreasing to -0.24 cm/day (± 0.50 SD; median = -0.32) after being partially consumed by the fireworm (Fig. 9). Colonies outplanted in Transect A (W = 77, P = 0.013) and B (W = 136, P < 0.0001) showed the largest decrease in growth rates, declining 17 % and 21 % faster than colonies in Transect C (Fig. 9). Growth rates before and after the predation event did not vary statistically among colonies growing in Transect C (W = 60, P = 0.40). The log-rank test based on the Kaplan-Meier survival curve (Fig. 10) indicates that fireworm predation did not significantly affect the survival of colonies (Chisq = 3.1, P = 0.08). Survival between predated and non-predated coral colonies differed only by 10 % towards the end of the study.
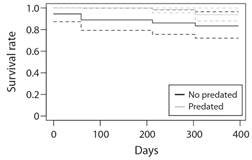
Fig. 10 Kaplan-Meier comparing survival pattern between predated and non-predated outplanted colonies of Acropora cervicornis.
Effect of initial size and the number of branches on predation rates: Results of the logistic regression analysis indicate that the vulnerability of a colony to be predated increases with the number of colony branches (i.e., branching complexity, z = 3.997, P < 0.0001) but not with colony size (z = -1.337, P = 0.181, Fig. 11). However, McFadens's R2 value of 0.03, a vif value higher than 5, and a ROC value of 0.53 indicate the models were not necessarily a good fit for the data; therefore, results should be interpreted carefully.
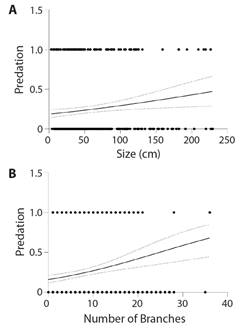
Fig. 11 Relationship between the probability of predation and colony size (A) and colony branching (B).
Population response to fireworm predation: The estimated population growth rate for the scenario considering only predated colonies was 0.99 compared to 0.91 when considering both predated and no-predated colonies (Fig. 12). Under the two scenarios, the demographic transition that contributes the most to the estimated λ was stasis of large colonies (Fig. 13). LTRE analyses indicate that the difference in λ could be attributed to a reduction in the stasis of large-sized colonies under the scenario considering both predated and non-predated outplants (Fig. 14).
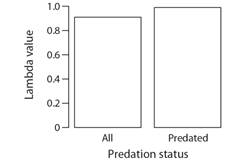
Fig. 12 Estimated population growth rates for two scenarios of predatory activity considering both predated and non-predated (ALL) and considering only predated colonies. Values below 1 indicate that the population growth rate is negative (i.e., declining).
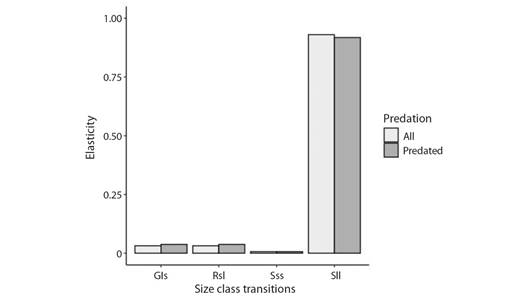
Fig. 13 Contribution of demographic transitions to the estimated population growth under two scenarios of predatory activities. Considering both predated and non-predated (ALL) and considering only predated colonies. S = stasis, G = growth, R = retrogression; s = small, l = large.
Discussion
The observed rate of predation by H. carunculata on A. cervicornis outplants was 84 %, which is ~1.5 times greater than what was reported in the Florida Keys (Miller et al., 2014). Biotic and abiotic factors specific to the localities, such as fireworm abundance and substrate complexity, may account for the contrasting results between our study and that of Miller et al. (2014). However, there is also the possibility that different methodological approaches could explain the higher predation rate we observed. For instance, Miller et al. (2014) assessed colony predation in restored populations two years old, whereas we followed predation on newly fixed coral outplants. Coral outplanting can be stressful either because of the pruning and handling or because coral fragments are placed in a new environment. Colonies not yet recovered from the collection event or acclimated to their new environment may be under higher stress than healed colonies already experiencing prevailing environmental conditions, making them more prone to be attacked by H. carunculata. Therefore, we could have quantified predation during a period when coral fragments were more susceptible to being predated. It could also be possible that after two years in the reefs, coral outplants developed a degree of resistance or deterrence toward fireworm predation. Indeed, Miller et al. (2014) found that predation rates by H. carunculata on A. cervicornis in both wild and restored populations were similar.
Fireworms were less likely to consume coral fragments originating from PMEL than from off-site nurseries. This finding suggests that exposure to a new environment rather than pruning or handling makes corals more vulnerable to predation. As a species that typically reproduces by branch fragmentation, A. cervicornis may be more able to deal with fragmentation-related damage than with changes in environmental conditions.
There was a significant decline in growth rates once the colony was partially consumed by the fireworm, providing further evidence of predation's demographic cost. Decreased growth rates after being predated could result from the coral diverting energy toward reducing mortality risk (i.e., Anthony et al., 2009). Predated colonies developed a calcified bulge (or neoplasia, Bak, 1983) at the interface between the live tissue and the exposed skeleton. The bulge, considered a physical barrier for repelling the spread of the algae colonizing dead portions of the colony, is energetically costly and may contribute to the observed decrease in coral growth while increasing survival probability (Bak, 1983; Mercado-Molina et al., 2018). Indeed, no significant difference in survival was observed between predated and non-predated outplants, as none of the affected colonies were consumed entirely or were infected by fireworm-borne diseases (see Miller et al., 2014). The low mortality of predated coral outplants opposes previous reports, such as the extirpation of colonies in Jamaica partly due to a fireworm outbreak (Knowlton et al., 1990). However, our finding agrees with that of Goergen et al. (2019), who observed minimal mortality in A. cervicornis wild populations during a non-outbreak period. Thus, it could be argued that H. carunculata may not lead to considerable colony loss if its population is relatively low.
As demonstrated in previous demographic studies on A. cervicornis (Mercado-Molina, Ruiz-Diaz, Pérez, et al., 2015; Mercado-Molina et al., 2020), the stasis of large colonies was the most important transition rate for population growth in PMEL. Large colonies are better able than smaller ones to withstand the negative demographic effects of partial mortality (Hernández-Delgado et al., 2014; Mercado-Molina, Ruiz-Diaz, Pérez, et al., 2015; Mercado-Molina et al., 2018) explaining, in part, the importance of large colonies for population viability. For instance, when a small colony of A. cervicornis loses more than 20 % of its living tissue, its survival is ~ 33 % lower than when a large colony loses the same amount of tissue (Mercado-Molina et al., 2018). Thus, the preference for H. carunculata predating on large colonies (see below) may contribute to the relatively low impact of predation at the population level.
Surprisingly it was found that the estimated λ for the demographic scenario considering only the colonies that were attacked by the fireworms was higher than when predated and non-predated colonies were combined. LTRE analysis indicates that such a result was due to the difference in the probability of large colonies surviving and remaining in the largest size class. Under the predated-only scenario, the stasis of large colonies was ~ 97 %, compared to 88 % when considering all colonies. This suggests that fireworm predation does not necessarily force corals into a smaller class size or lead to the death of the colony. It also implies that another source of tissue loss (or death) affects the demographic transitions of large colonies not predated by the fireworm. We witnessed some colonies predated by the corallivorous snail Coralliophila abbreviata; however, the population-level effect of snail predation may be limited because very few colonies were affected. It is known that A. cervicornis could be very susceptible to minor or moderate local variations in environmental parameters (Mercado-Molina, Ruiz-Diaz, Pérez, et al., 2015); thus, it is possible that changes in environmental conditions not perceptible to us (e.g., light incidence, temperature) led to the mortality of colonies not predated by H. carunculata.
Goergen et al. (2019) and Miller et al. (2014) found that colony attacks by H. carunculata did not follow a consistent temporal pattern. In contrast, we found that the incidence and prevalence of predation showed an increasing trend over time. The discrepancy between studies could be associated with the capacity of corals to heal after predation. In the case of Miller et al. (2014), some colonies regained the lost tissue, which we did not observe during our study. A low capacity to regenerate the tissue that has been lost is not uncommon in A. cervicornis (Miller et al., 2014; Mercado-Molina et al., 2018). However, it is unclear why some colonies can heal while others do not. Determining the effects of predation on the immune system of the coral can provide valuable insights into how injured colonies recover (Ruiz-Diaz et al., 2016); thus, comparative studies between predated and non-predated colonies are recommended.
The positive relationship between colony complexity and the probability of being predated could also explain the temporal increment in predation incidence and prevalence. In A. cervicornis, branch formation tends to increase with colony size (Mercado-Molina et al., 2016); consequently, as a colony grows, it becomes more complex. Morphological complex corals could provide a higher surface area of nearby tissue for consumption while allowing the fireworm to spend little energy foraging. In fact, most of the predated colonies had multiple branches consumed. Feeding on large and more complex colonies could also result from the fireworm preferring colonies in a compromised energetic state. For instance, as A. cervicornis grows, it devotes more energy to growth than maintenance and defense (Darling et al., 2012; Mercado-Molina, Ruiz-Diaz & Sabat, 2015; Mercado-Molina et al., 2016), possibly limiting its ability to counteract fireworm aggression.
Although coral outplants across the three transects (Transect A through Transect C) showed a progressive increase in fireworm predation over time, the area where corals were located proved to be critical, as suggested by Goergen et al. (2019) and Miller et al. (2014). Predation rates were greater along Transect C, probably because the fireworm was more abundant along this transect. Transect C was the most complex of the three reef areas studied. For a cryptic species, such as H. carunculata, a substrate characterized by many crevices and holes can provide protection and may increase abundance, as was the case in our study. Nevertheless, Wolf et al. (2014) found the highest abundance of H. carunculata in areas of low rugosity. Therefore, it is possible that the spatial distribution of the fireworm across our study site could be related to other factors not necessarily associated with the structure of the reef substrate (e.g., sediment composition, Wolf et al., 2014).
In conclusion, this study confirms that H. carunculata can predate heavily on A. cervicornis outplants (Calle-Triviño et al., 2017; Miller et al., 2014), negatively affecting coral growth. Nevertheless, outplant survival was not compromised. Such a demographic response to predation assures a minimal effect at the population level. Being large and complex is a buffer against predation because even when outplants are partially consumed, their survival tends to be high. Thus, outplanting large and complex colonies could be a strategy to improve the success of coral reef restoration (Goergen & Gilliman, 2018; Mercado-Molina, Ruiz-Diaz, & Sabat, 2015; Pérez-Pagán & Mercado-Molina, 2018), especially when introducing colonies from external sources. Reduced growth rate, however, can be prejudicial in the long term by, for example, delaying the time a coral outplant can reach a refuge size and its reproductive potential. H. caranculata could also be a vector of coral diseases (Miller & Williams, 2007; Miller et al., 2014; Sussman et al., 2003). Thus, it is recommended to keep fireworm populations as small as possible. When designing a restoration program, attention to the area (e.g., small-scale topographic relief) where corals will be outplanted must be considered. However, it is not clear yet what features of the reef's substrate better explain changes in the abundance of H. carunculata. Studies in this direction are urgently needed.
Ethical statement: The authors declare that they all agree with this publication and made significant contributions; that there is no conflict of interest of any kind; and that we followed all pertinent ethical and legal procedures and requirements. All financial sources are fully and clearly stated in the acknowledgements section. A signed document has been filed in the journal archives.