Introduction
Coral reefs are considered one of the world's most productive and biodiverse ecosystems and provide important human services (Spalding et al., 2001). Their high biodiversity is related to the physical complexity of the reef structure, benthic groups heterogeneity, and architectural three-dimensionality, all dependent upon coral accretion (Álvarez-Filip et al., 2009). Unfortunately, coral communities are also among the most threatened ecosystems due to the cumulative effects of multiple natural and anthropogenic stressors that have led to massive bleaching and mortality events worldwide (Brainard et al. 2018; Spalding & Brown, 2015; Vargas-Ángel et al., 2019); over the past 20 years alone, at least 50 % of the coral reefs have been lost, and the remaining 70-90 % are considered to be highly threatened, becoming urgent and an international priority for their conservation (Souter et al., 2020)
Active restoration programs are now frequently employed (Boström-Einarsoon et al., 2020) as a tool to mitigate the loss of coral cover by ''outplanting” corals to where ecosystem assemblage and function are re-established (Bayraktarov et al., 2016; Edwards, 2010); complete reef rehabilitation is the long-term goal of most such initiatives (Boström-Einarsoon et al., 2020; Montoya-Maya et al., 2016; Shackelford et al., 2013). However, factors such as community life history, historical shifts in the local biota, spatio-temporal variation in regional- and local-scale environmental conditions (particularly seawater quality), and the physiology of the target restoration species must all be considered prior to active restoration efforts (Combilet et al., 2022; Montoya-Maya et al., 2016; Suding, 2011).
Monitoring of outplants is imperative (Guest et al., 2011; Shaish et al., 2008), as the success of any restoration program does not only depend on the technique initially employed but also on the local response of the coral species (Bayraktarov et al., 2016; Sunding, 2011). Since coral growth and survival are influenced by temperature, light, wave energy, sedimentation, nutrients, pH, and aragonite saturation state (Lough & Cooper, 2011; Manzello et al., 2017), it is critical to demonstrate that the outplants can acclimatize to what might be distinct conditions from where the source colonies were obtained (Shackelford et al., 2013). Given this, it is surprising that seasonal variation has not been considered in the early stages of restoration projects, especially given that coral growth and survival may change over monthly and annual timescales (Lough & Cooper, 2011; Manzello et al., 2017; Romero-Torres et al., 2020; Tortolero-Langarica et al., 2017).
Most coral restoration sites are located in the Caribbean and Indo-Pacific regions (Boström-Einarsoon et al., 2020), with few efforts underway in the Eastern Tropical Pacific (ETP) despite the massive loss of coral cover in the region that has occurred in recent years (e.g., Carriquiry et al., 2001). Effective restoration protocols recently implemented along the ETP, though it is important to 1) select robust corals and, 2) target the coral species that have historically been distributed in the site to be restored (Combilet et al., 2022; Ishida-Castañeda et al., 2020; Lizcano-Sandoval et al., 2018; Nava & Figueroa-Camacho, 2017; Tortolero-Langarica et al., 2014, Tortolero-Langarica et al., 2019; Tortolero-Langarica et al., 2020). Herein we used a low-impact approach to evaluate the growth of Pocillopora spp. outplants over time in the Northeastern Tropical Pacific. We hypothesized that seawater temperature (SWT, i.e., season) and reef location (offshore vs. inshore) could influence coral growth and survival over the one-year monitoring period.
Materials and methods
The Central Mexican Pacific (CMP) harbors one of the most important coral communities in the ETP (Glynn & Ault, 2000). The coral community of Islas Marietas National Park, an offshore marine protected area (referred to as simply ''Islas Marietas” throughout the text) located 7.9 km northeast (20.69 °N, 105.57 °W) to an inshore reef known as ''Punta de Mita” (PM, 20.76 °N, 105.54 °W). Both sites (Fig. 1), used to maintain a coral cover of nearly 40 % in the late 1990s, but mass coral bleaching during the 1997-98 ENSO event led to 90 % mortality (Carriquiry et al., 2001). Afterwards, coral cover in the region showed a continued decline in response to more frequent, intense and, unpredicted stress events (e.g., ENSO; Santoso et al., 2017) and localized anthropogenic pressures (e.g., tourism, overfishing, and coastal development); nevertheless, a slow but steady recovery in specific areas have been recorded (Cupul-Magaña & Rodríguez-Troncoso, 2017; Martínez-Castillo et al., 2022), acting as a possible refuge of coral reef organisms as observed in other subtropical reefs, as long as management and conservation strategies are implemented (Beger et al., 2014).
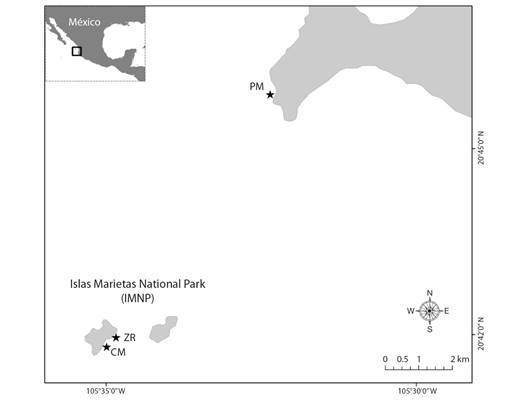
Fig. 1 Study area. Offshore sites in Islas Marietas National Park (IMNP): Zona de Restauración (ZR) and Cueva del Muerto (CM). Inshore reef: Punta de Mita (PM).
At Islas Marietas, the mean SWT is ~ 27 °C, with an annual range of 18-30 °C (Palacios-Hernández et al., 2010). The coral community is characterized as a patchy reef, located between 1-15 m, with the highest live coral cover, with high abundance of branching corals ≤ 8 m depth (Hernández-Zulueta et al., 2017), as also the presence of bare rock and few dead coral skeletons (Sotelo-Casas et al., 2019). The area is a touristic hotspot due to the high quality seascape for SCUBA and free-diving, which in past years have caused physical damage to the coral colonies, and in addition to other local-scale impacts such as seawater pollution, led to the implementation of a program designed to restrict the number of visitors (Cupul-Magaña & Rodríguez-Troncoso, 2017).
The PM reef experiences even higher anthropogenic pressure due to rapid coastal urbanization, the development of golf courses, and luxury tourist development (ECOPLAMB, 2004), which have become the main local stressors as inorganic nutrient levels and sedimentation have increased from terrestrial run-off (Martínez-Castillo et al., 2020). The SWT in situ averages ~ 26 °C, ranging from 20.8 to 30.6 °C (Palacios-Hernández et al., 2010). The area used to harbor a well-developed shallow reef patch with a ≈ 35 % of live coral coverage (Martínez-Castillo et al., 2022), which was lost during the mass mortality associated to the intense 1997-98 ENSO event (Carriquiry et al., 2001), leaving a full path of dead coral matrix as available substrata to transplant coral fragments. The coral coverage has shown a slow natural recovery; however, due to its depth (1-3 m) and proximity to the coast, it is considered a highly vulnerable site, but with the potential to be restored despite these sub-optimal local conditions (Martínez-Castillo et al., 2020; Martínez-Castillo et al., 2022).
The sites to restore were selected according to the following characteristics: 1) historical high coverage (over 30 %) of Pocillopora spp. colonies and 8-10 % of healthy coral coverage, 2) stable dead coral matrix or rocks with cavities upon which coral fragments could attach, 3) healthy fragments of opportunity. The coral fragments are referred to as ''fragments of opportunity”, i.e., those detached from parent colonies through natural processes such as currents, swells, or bioerosion (Monty et al., 2006). The restoration protocol was implemented at two offshore sites from Islas Marietas, Zona de Restauración (ZR) and Cueva del Muerto (CM), as well as the only coastal patch reef PM. Fragments were arranged in a total area of 200 m2 at ZR (~5-7 m depth), 75 m2 at CM (~ 4-6 m), and 125 m2 at PM (~ 2-3 m).
The reef restoration protocol was based on the method described by Tortolero-Langarica et al. (2014). Initially, fragments of opportunity (50-100 mm in length), were collected near the restoration area (no further than ≈ 50 m away) and carefully inspected in situ to avoid the use of no-healthy fragments with evidence of bleaching, tissue damage, or bioeroding sponges (e.g., Cliona spp.). Each healthy fragment was fixed to the natural substrata using plastic cable ties (sensuEdwards, 2010), either in a horizontal or vertical orientation (depending on fragment morphology and substrate geometry), to reach the best support (tightening of the cable tie; Fig. 2A-D). During the first restoration effort, 183 fragments were tagged and assessed monthly during a one-year period (Fig. 2B) at ZR (n = 63), CM (n = 78), and PM (n = 42). Individually, both length (maximum linear distance from base to tip) and width (maximum diameter perpendicular to the vertical axis of the fragment) were measured using plastic calipers (FOY 6”; precision = 0.05 mm; Fig. 2C). Self-attachment was considered to have occurred when evidence of calcification at the base could be visualized and quantified as a percent of fragments per month (% mo); the survival rate was also recorded (%).
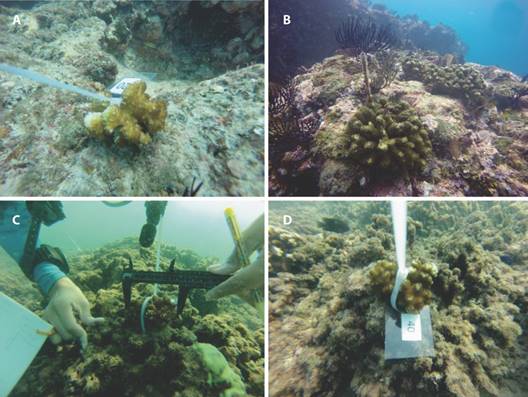
Fig. 2 Coral propagation technique. A) Coral vertically attached to rocky substrata (site: Cueva del Muerto). B) Example of one-year coral colony after intervention; the cable tie stays within the colony for visual identification. C) Fragment measured by using plastic calipers, D) Coral horizontally attached to dead stable coral matrix.
It is important to notice that the cable tie was not removed as the fragment calcified around it, and the removal could detach the colony from the substrata; and it is a ''visual reference” to differentiate the natural recruited from the transplanted colonies. In addition, to continue with the restoration process afterward outplanting, 1 139 fragments were transplanted but not tagged across both inshore and offshore sites.
In addition, sea water temperature (SWT) was recorded in situ every 25 min with digital loggers (HOBO® Pendant Onset) installed near the fragments at each restoration site. Coral growth was correlated with the season (Palacios-Hernández et al., 2010), with a specific emphasis on uncovering differences between the temperate-dry season (January to May) and the warm-rainy season (August to December).
Annual (mm yr-1) and monthly growth (mm mo-1) rates were calculated, and all data were tested for normality (P < 0.05) and homogeneity of variance (P < 0.05) using Shapiro-Wilk and Levene's tests, respectively. Since data neither exhibited normal distributions nor homogeneous variances, non-parametric Kruskal-Wallis tests were instead used to test for differences in coral growth over time and across sites. Whenever results were significant, post-hoc comparisons were calculated using Dunn's tests (P < 0.05). Chi-squared tests were used to compare attachment and survivorship (rate and percentage, respectively) between sites and between seasons. A Mann-Whitney test was used to compare coral growth between Islas Marietas reefs (offshore) and PM (inshore patch reef). All statistical analyses were performed using SigmaPlot® ver. 11 (SPSS Inc., USA).for Windows, and an alpha of 0.05 was set for all analyses.
Results
At the end of the year, 84 % of the fragments survived, and PM presented a survival rate of ~ 75 % that was significantly higher than the other two sites (X2 = 6.96, P < 0.05; Fig. 3A). Also, differences in self-attachment were observed between sites; at PM, fragments attached after four months, with an overall attachment rate of 83 % by the end of the year. Meanwhile, ZR presented the lowest rates, as the first fragments were attached after nine months, and by the end of the year, only 36 % were complete fixed to the substrata (Fig. 3B).
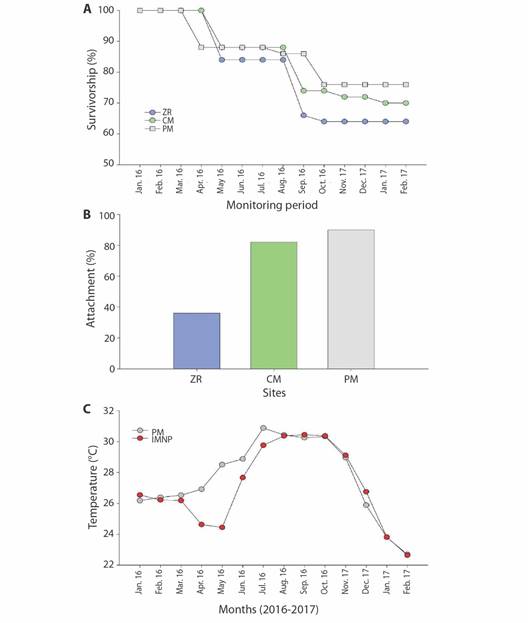
Fig. 3 Coral fragment (%) survivorship and attachment. (A) Coral survivorship over one-year (2016-2017). (B) Coral attachment efficiency, inshore reef: Punta de Mita (PM), offshore reefs: Zona de Restauración (ZR) and Cueva del Muerto (CM).
Seawater temperature was recorded for both sites. Islas Marietas (offshore site) temperature average was 27.07 ± 0.07 °C with the minimum values during February (22.6 ± 0.02 °C) and a maximum in September (30.4 ± 0.01 °C). At PM (inshore site), the average SWT was 27.6 ± 0.67 °C, and it ranged between 22.6-30.8 °C during the same months (Fig. 4). Significant SWT differences were detected between zones and seasons; the temperature's most noticeable difference was at ZR in the warm-rainy season (H' = 26.97 interaction effect of season x site, P < 0.001).
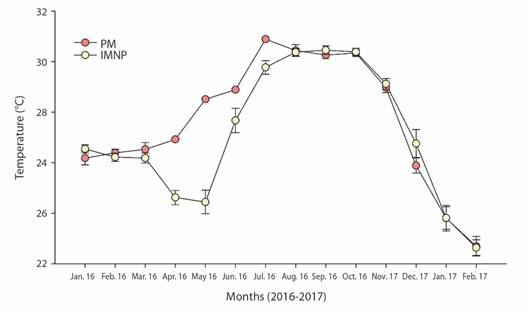
Fig. 4 Seawater temperature (mean ± SD) recorded in situ at Punta Mita inshore (PM = orange circles) and Islas Marietas offshore (IM = yellow circles) restoration sites.
The mean monthly coral growth rate was of 3.3 ± 0.1 mm mo-1; the lowest growth rate was recorded at ZR (2.3 ± 0.1 mm mo-1), and the highest growth rate was recorded at PM (4.3 ± 0.1 mm mo-1; Fig. 5A). Significant differences were recorded among sites (H = 92.210, P < 0.001), with growth rates of corals of ZR significantly lower. An overall difference between the Islas Marietas sites and PM was also observed (U-Mann = 22410, P < 0.001). Finally, no variation in growth rate between sites was observed (Fig. 5B).
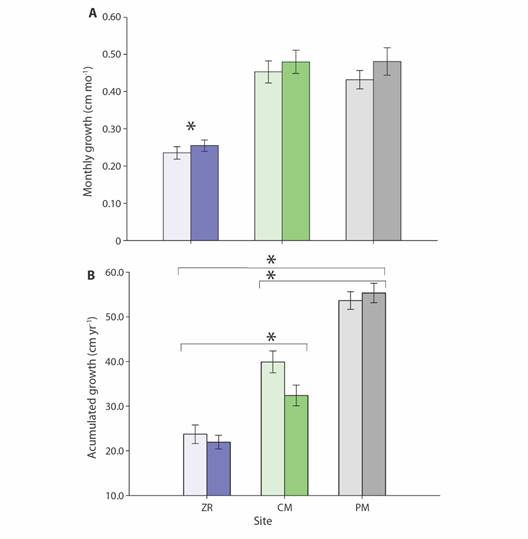
Fig. 5 Growth (mm ± SE) of Pocillopora spp. fragments. A) mean monthly growth rate (mm mo-1 ± SE). B) mean annual growth rate (mm yr-1 ± SE). Sites: Punta de Mita (PM), Zona de Restauración (ZR) and Cueva del Muerto (CM). Light color bars = length, intense color bars = width. Asterisk (*) denote statistical differences between groups.
After one-year intervention period, coral growth ranged from 51.2 ± 1.7 to 82.4 ± 3.4 mm yr-1 in length and from 34.6 ± 2.0 to 66.1 ± 3.1 mm yr-1 in width (Fig. 6A). After one-year, these corals presented an overall size increase of 63.7 % (pooled data), with higher growth rates in PM (92 %) than in the colonies of Islas Marietas (39 %). The annual accumulated growth was 39.9 ± 11.5 mm yr-1 in length and 36.5 ± 19.5 mm yr-1 in width, with higher mean values for PM (53.6 ± 12.7 mm yr-1) vs. the two offshore reefs (31.8 ± 11.4 mm yr-1; H' = 95.267, P < 0.001; Fig. 3), with no differences between seasons (P > 0.05; Fig. 6B).
Discussion
Asexual propagation is the method primarily used in coral restoration programs (Boström-Einarsson et al., 2020). Prior implementation is important to clear out the motivation, variables, and possible outcomes (Bayraktarov et al., 2019; Suding et al., 2015). Both the method of obtaining fragments (e.g., fragmentation of adult and healthy colonies), as well as the transplantation, which usually includes the introduction of nurseries and, a posterior outplanting phase, led to increasing the financial cost with ≥ 55 % of long-term survival rate (Bayraktarov et al., 2016; Bayraktarov et al., 2019, Boström-Einarsson et al., 2020). The ETP region's hydrodynamic conditions restrict the suitable sites where nurseries or artificial reefs can be installed (Combilet et al., 2022); therefore, direct propagation has become one of the most practical and standardized active restoration strategies to rehabilitate coral communities along the ETP (Lizcano-Sandoval et al., 2018; Nava & Figueroa-Camacho, 2017; Tortolero-Langarica et al., 2014; Tortolero-Langarica et al., 2019). However, to increase the efficiency before the propagation, fragments of opportunity should be individually assessed to avoid non-healthy fragments, use the in-site substrata (e.g., rock or dead coral matrix), and assume that physiological indicators, such as growth, survival, and resistance, will differ between sites (in this case offshore and inshore coral communities) and possibly over the years, as a response to local and regional pressures. Therefore, direct relocation of corals may require local modifications to the protocol, including the selection of substrata, use of tie wraps, epoxy glue, marine cement, etc., according to the site characteristics.
Restoration programs should prioritize the biological assemblages, species composition and representation to promote conditions from historical trends (Guest et al., 2011; Suding et al., 2015). The ETP reef and coral communities are considered mono-specific as Pocillopora spp. is the most abundant reef-builder, which, despite the effect of massive bleaching and mortality events, has shown recently to have high resistance and resilience to non-optimal conditions (Manzello et al., 2017; Rodríguez-Troncoso et al., 2016; Romero-Torres et al., 2020). Besides their coverage, branching corals have higher growth rates and provide more available shelter or substrata to other organisms than other local genera, such as massive Pavona spp. or encrusting Porites spp. (Lizcano-Sandoval et al., 2018; Nava & Figueroa-Camacho, 2017; Tortolero-Langarica et al., 2014; Tortolero-Langarica et al., 2019; Tortolero-Langarica et al., 2020), increasing the relevance of promoting active protocols to increase their presence in the coral community.
The results showed differences in growth rate at the micro-scale level, despite their proximity; and also, unexpectedly, the growth rates were lower than those previously recorded in the central Mexican Pacific (Nava & Figueroa-Camacho, 2017; Tortolero-Langarica et al., 2014; Tortolero-Langarica et al., 2019). Coral growth responds to regional conditions and global phenomena (Manzello et al., 2017; Tortolero-Langarica et al., 2019), promoting similar effects along the region; however, local conditions (e.g., depth, wave action, nutrient concentration, and space competitors) also determines the growth rate (Lough & Cooper, 2011). A seasonal increase of nutrients in the region can be caused by seasonal upwellings (Palacios-Hernández et al., 2010), but at Punta Mita the constant run-offs cause abnormal high concentrations of inorganic nutrients and sediments (Martínez-Castillo et al., 2020) from fertilizers of the gulf course or discharged from the touristic development (ECOPLAMB, 2004). Corals from Punta Mita exhibited a higher growth rate than Islas Marietas colonies, which is surprising as PM has been described as a less-optimal site for coral development, but the high light regime due to the low depth and high nutrients, are beneficial conditions for the endosymbionts and consequently energy translocated for growth (Fabricius, 2005; Martínez-Castillo et al., 2020). Furthermore, both conditions also contribute to the corals'ability to feed by suspension (Anthony & Fabricius, 2000); however, the long-term effect on coral skeletal density has to be determined, as it can result in less dense and more fragile skeletons, which can increase the vulnerability of the reef framework (Chazottes et al., 2002; Fabricius, 2005).
Both, short- and long-survival rates are indicators of success (Bayraktarov et al., 2016). The results showed a ≥ 83 % of survival, and specifically, Punta de Mita showed a higher survival rate than the offshore sites and high self-attachment to the substrata. For restoration purposes, fragment survival and attachment depends as previously mentioned, by fragment health but also the stress caused to corals due to manipulation, depth, bio-erosion, macro-algal competition, the proximity of reefs to sources of anthropogenic stress and fragment size, yet these were no evaluated herein (Bowden-Kerby, 2001; Guest et al., 2011; Shaish et al., 2008). In addition, despite coral fragments used in this study were visibly healthy and showed no signs of bleaching, algal cover, or sponge, ~ 50 % of Pocillopora spp. coral colonies along the ETP are invaded by Cliona vermifera, the most abundant coral-excavating sponge (Alvarado et al., 2017; Bautista-Guerrero et al., 2014), which may compromise the coral colony structure and negatively impacts coral attachment.
Seasonality could also be considered as a determining factor in the success of the restoration program as seawater temperature is one of the most important factors for coral growth, reproduction and survival (Anthony & Fabricius, 2000). In the central Mexican Pacific SWT range from 19 °C during the temperate-dry season to 31 °C during the warm-rainy season, with daily minimum-maximum ranges of 5 °C, which are attributed to seasonal upwelling and internal waves (Palacios-Hernández et al., 2010). Our surveys show a higher growth rate during the warm-rainy period, which can be considered a regular pattern (Tortolero-Langarica et al., 2017), as long as the temperature does not overcome the species'thermal threshold.
Therefore, ENSO-driven temperature anomalies and the historical local resistance of the corals should also be considered. As previously described, the 1997-98 El Niño event elicited bleaching and massive mortality in the region (Carriquiry et al., 2001), with evidence of low but constant recovery at specific sites along the ETP (Rodríguez-Troncoso & Cupul-Magaña, 2016; Rodríguez-Troncoso et al., 2016; Romero-Torres et al., 2020) although the influence of quasi-oscillatory positive and negative thermal anomalies occurring in the region in an average period of 2 years (Palacio-Hernández et al., 2010; Santoso et al., 2017). The transplantation coincided with the 2015-2016 El Niño event, so far considered the most extreme of the 21st century (Santoso et al., 2017), with a dramatic impact on coral reefs along the equatorial Pacific (e.g., Brainard et al., 2018, Vargas-Ángel et al., 2019). However, at the CMP, the thermal anomalies did not cause massive bleaching or mortality but can explain the lower growth rates. Still, the survival rate is higher than the one recorded in other studies (Bayraktarov et al., 2016; Boström-Einarsson et al., 2020), and more importantly, a positive growth rate during adverse global and local (urban development and touristic activities) conditions.
The correct implementation of a well-structured restoration initiative and long-term assessment and monitoring stand to bring ecological and societal benefits, particularly in regions characterized by high urban and touristic pressures. Any restoration protocol must be replicable at a regional level and have the flexibility to consider the local characteristics of the site. Before starting a restoration program, it is suggested to evaluate the area and consider: 1) presence of fragments of opportunity or donor colonies, 2) the availability of natural substrata, 3) Evidence of low density of internal bioeroders, 4) determining those sites that may be donors but are not optimal for intervention, 5) seasonal temperature fluctuation and nutrients and sedimentation rate, and 6) the resistance or ability to recover from stress conditions (e.g., ENSO events). The success of any restoration program depends to a large extent on establishing them in sites that show in their recent history a capacity for acclimatization to stressful conditions, and when implemented in the continuous monitoring, as the goal is to reach the self-sustaining, where human intervention is minimal and only passive restoration tools are necessary, assisting the recovery of the coral coverage and the associated organisms but to reach the rehabilitation of the site (Boström-Einarsson et al., 2020). The Mexican central Pacific has proven to be a reef area that can be considered a coralline refuge, where active restoration protocol should be planned for the long term to ensure the maintenance of these high-value ecosystems.
Ethical statement: the authors declare that they all agree with this publication and made significant contributions; that there is no conflict of interest of any kind; and that we followed all pertinent ethical and legal procedures and requirements. All financial sources are fully and clearly stated in the acknowledgements section. A signed document has been filed in the journal archives.
Author contributions: RPG, APRT, ALCM conceived and designed the research; RPG, APRT, ALCM JJATL performed the fieldwork and obtained the data; RPG, APRT, JJATL, LDKG analyzed the data; APRT, ALCM contributed materials and field trips; RPG, APRT, JJATL wrote and edited the manuscript.